ABSTRACT
The features of N transformations in humid subtropical soils vary greatly due to the wide variations of soil properties, particularly soil pH. Purpose: To understand whether the effects of countermeasures regulating N transformation varied with the prevailing soil N transformation characteristics. Materials and methods: The effects of nitrapyrin, rice straw and its biochar applications on N dynamics at the beginning stage (first 24 h) of a zonal acid soil (JX soil, pH 5.26) and a non-zonal alkaline soil (SC soil, pH 7.62) from the humid subtropics in China were investigated via a 15N tracing laboratory incubation. Results and conclusions: Rice straw incorporation affected almost all processes involved in and
production and consumption, and decreased the net mineralisation and nitrification rates in both soils significantly. Nitrapyrin decreased the net nitrification rates in both studied soils by affecting different N transformation processes. In the SC soil, nitrapyrin reduced the net nitrification rate by inhibiting the gross autotrophic nitrification rate while the gross autotrophic nitrification in the JX soil was negligible and nitrapyrin decreased its net nitrification via a stimulation of the gross rate of dissimilatory nitrate reduction to ammonium (DNRA). Biochar stimulated the gross rates of mineralisation,
immobilisation, autotrophic nitrification and DNRA significantly, but did not affect the net mineralisation and nitrification rates in both soils. Countermeasures to regulate N transformations are tightly linked to the prevailing soil N transformation characteristics and should be taken into account for the recommendation of suitable management options.
Introduction
Previous investigations (Zhang et al. Citation2013) have reported that autotrophic nitrification rate was commonly low, resulting in the inorganic N being dominated by -N in the zonal soils, which are well weathered and acidic in the humid subtropical China. Thus the risk of nitrate leaching and runoff is minimal and N can potentially be conserved effectively in the soils since due to the low pH, ammonia volatilisation are also very low. In contrast to the zonal mature soil occurring in humid subtropical regions, majority of non-zonal purple soils classified as Purpli-Udic Cambisols that are neutral or alkaline and have developed from purple rocks, are widely distributed in the hilly areas in the upper reaches of the Yangtze River (Li et al. Citation2016). High soil pH in purple soils is associated with large nitrification rates and a predominance of
-N (Wang et al. Citation2015). The purple soils are also prone to erosion due to the hilly topography, intensive cultivation and the wet climate in the areas. Therefore, nitrate leaching and runoff is a key pathway of N loss and an important nitrogen pollution source in the areas where purple soils occur (Zhu et al. Citation2009; Yang et al. Citation2013). To reduce the risk of N losses through nitrate leaching and runoff in purple soils, various countermeasures have been proposed over the past few decades (Zhu et al. Citation2009; Zhou et al. Citation2012; Wang et al. Citation2015).
Nitrification inhibitors have been used to reduce the potential for N loss and increase fertiliser use efficiency (Islam et al. Citation2007; Fangueiro et al. Citation2009; Chen et al. Citation2010; Fisk et al. Citation2015). Nitrification inhibitors are natural or synthetic compounds that retard being oxidised to
by microorganisms, which is the first step of the nitrification process by suppressing the activities of ammonia oxidisers (especially ammonia oxidising bacteria (AOB)) in the soils. This delay conserves the immobile
in the soil for a distinct period, thereby reducing the production of
that can easily leach and runoff under wet conditions.
Globally, agricultural production generates approximately 4 billion metric tons per year of crop residues (Lal Citation2005). Crop residues are widely used as additives that sustain soil organic matter (SOM) and influence the N transformations dynamics and N availability (Ocio et al. Citation1991; Bird et al. Citation2003). The simultaneous addition of N fertilisers and crop residue incorporation may stimulate microbial N immobilisation during the initial decomposition of labile straw-derived organic matters because of the high C/N ratio of these matters (Fontaine and Barot Citation2005; Moorhead and Sinsabaugh Citation2006). Generally, in the condition of whether using composts or not applying organic matters, nitrate is difficult to be immobilised, but the existence of crop residues can improve the assimilation of nitrate as same as ammonium (Nishio et al. Citation2001). Nevertheless, incorporation of crop residues in the soil may also promote the decomposition of native soil organic matter because of a positive priming effect (Muhammad et al. Citation2007).
Another strategy that has potential agronomic benefits is application of biochar to agricultural soils. Biochar is defined as a carbon rich and biodegradation-resistant product created by thermally decomposing organic materials under partial or complete exclusion of oxygen and temperatures of <700°C (Lehmann and Joseph Citation2009). Addition of biochar can affect a few soil nitrogen cycle processes and the effect depends on the biochar feedstock and its pyrolysis conditions, as well as local environmental and climatic conditions and the respective soil properties (Clough et al. Citation2013). However, the results of biochar effects on soil nitrogen dynamics are contradictory. For example, it has been proven that biochar can stimulate, reduce or dose not influence soil N mineralisation (Cheng et al. Citation2012; Dempster et al. Citation2012; Nelissen et al. Citation2012). It has also been reported that biochar can enhance N immobilisation or had no effect (Bruun et al. Citation2012; Cheng et al. Citation2012), as also for nitrification are the same (Castaldi et al. Citation2011; Song et al. Citation2014). Therefore, more research is needed to elucidate the full effects of biochar on N transformations in agricultural systems to fill in the gaps in our current understanding of the inherently connected interaction between biochar and the N cycles (Clough and Condron Citation2010).
Although many investigations have been carried out about the influences and mechanisms of regulation measures, only little attention has been paid on how the intrinsic N transformations affect the impact of countermeasures on regulating N transformations so far. It is particularly important to measure gross N transformation rates, as net rates indicate the combined effects of gross N transformation in soil, making it difficult to understand the actual rate of N cycling in soils (Hart et al. Citation1994). We hypothesised that different countermeasures could reduce nitrate contents via influencing different N transformation processes. Consequently, the objective of this research was to compare the short-term instantaneous effects of nitrapyrin, rice straw and its biochar applications on N transformation, particularly on N supply and nitrate contents, in the zonal acid soil and non-zonal purple soil in the humid subtropical China by determining the soil gross N transformation rates.
Materials and methods
Soil samples
The studied soils were sampled from plough layers (0–20 cm) of the Yanting Agro-Ecological Station of Purple Soil, Sichuan Province (SC) and Longhushan, Jiangxi Province (JX), respectively. The climate of the site in SC is moderate subtropical monsoon. The annual precipitation in this site is 1157 mm while the annual average air temperature is 17.3°C. Similarly, characteristics of the site in JX is also a typical moderate subtropical monsoon climate. Its average annual precipitation is 1785 mm and annual air temperature is 18.4°C. The soils collected from SC was derived from purple sandstone and classified as Cambisols (FAO-UNESCO-ISRIC Citation1990). The soil collected from JX was derived from Tertiary red sandstone and classified as Acrisols (FAO-UNESCO-ISRIC Citation1990). All soil samples were sieved to pass through 2 mm mesh, homogenised, and then kept at 4°C before the laboratory 15N-tracing experiment. The soil properties were presented in .
Table 1. Properties of the studied zonal acid (JX) and non-zonal alkaline (SC) soils collected in the humid subtropical China.
Treatments of the 15N tracing experiment
For each soil, there were four treatments, respectively: (1) control without countermeasures (CK), (2) application of nitrapyrin (NC), (3) incorporation of rice straw (NS), (4) addition of biochar made from the rice straw (NB). There were two 15N-labelled treatments in this experiment (each in triplicate), one was labelled with ammonium (15NH4NO3) at 10.22 atom% 15N excess, and the other one was labelled with nitrate (NH415NO3) at 10.19 atom% 15N excess. 20 g of soil (oven-dry basis) were prepared for each treatment in the 250 mL Erlenmeyer flasks. Soil samples in the 250 mL flasks were mixed with ground (0.3 mm) rice straw (0.2 g, equivalent to 1% of soil) or biochar (0.2 g, equivalent to 1% of soil) for NS and NB treatments, respectively. The properties of straw and biochar were shown in . These flasks were all pre-incubated at 25°C for 12 h to study the short-term instantaneous of countermeasures on regulating soil N transformations. Then two millilitres of 15NH4NO3 or NH415NO3 solution were added to separate flasks evenly to provide concentrations of 30 mg -N kg−1 soil or 30 mg
-N kg−1 soil. For the NC treatment, nitrapyrin was applied simultaneously with NH4NO3 at a concentration of 2.5‰ of the applied N content. Then, adjusted each treatment to 60% water holding capacity (WHC) by adding distilled water. All flasks were incubated at 25°C for 24 h. After adding NH4NO3, the soil samples were extracted at 0.5, 6, 12 and 24 h with 2 M KCl solution and the ratio was 1:5 (w/v) soil/solution. The extracts were used to detect the inorganic N concentrations and their 15N enrichments.
Table 2. Properties of rice straw and biochar made of rice straw for use in the study.
Analyses of soil properties
According to Lu (Citation2000), the soil properties such as pH, soil organic C (SOC) and total N (TN) were analyzed. A DMP-2 mV/pH detector (Quark Ltd, Nanjing, China) was used to determine soil pH in a ratio of 1:2.5 (v/v) soil/water. In order to determine SOC and TN, wet-digestion and semi-micro Kjeldahl digestion were used, respectively. Soil samples were extracted with 2 M KCl and shaken for 1 h. Then, a part of the extracts was added with MgO and distilled for concentration analyzing, subsequently adding Devarda’s alloy to the sample, followed by distillation once again to determine
concentration by titration with H2SO4. The distillates were acidified with H2SO4 and dried at 80°C after titration. The 15N abundance of inorganic N samples was detected using an isotope ratio mass spectrometer coupled with an automated C/N analyzer (IRMS 20–22, Sercon, Crewe, UK).
15N tracing model
According to Müller’s model, ten gross N transformation processes occurred simultaneously were quantified (Müller et al. Citation2007): MNlab, mineralisation of labile organic nitrogen to ammonium; INH4_Nlab, immobilisation of ammonium to labile organic nitrogen; MNrec, mineralisation of recalcitrant organic nitrogen to ammonium; INH4_Nrec, immobilisation of ammonium to recalcitrant organic nitrogen; ONrec, oxidation of recalcitrant organic nitrogen to nitrate (heterotrophic nitrification); ONH4, oxidation of ammonium to nitrate (autotrophic nitrification); INO3, immobilisation of nitrate to recalcitrant organic nitrogen; DNO3, dissimilatory nitrate reduction to ammonium (DNRA); ANH4, adsorption of ammonium on cation exchange sites and RNH4ads, release of adsorbed ammonium. In order to calculate the transformation rates, we applied the measured inorganic N concentrations and 15N excess to the model and used zero-order, first-order or Michaelis–Menten kinetics. This tracing model minimised the misfit between observed and modelled inorganic N concentrations and their 15N excess to optimise the kinetic parameters for varieties of N transformations synchronously for calculating gross rates (Müller et al. Citation2007).
Calculation and statistical analyses
In view of the kinetic parameters and settings in the model, the units of gross N transformation rates were mg N kg−1 soil day−1.
Based on equations 1a and 1b, the total gross mineralisation rates (TM) and total gross immobilisation rates (TI) were quantified, respectively.
(1a)
(1b) Difference between the inorganic N concentrations (
+
) of the final and initial stages were presented as the net mineralisation rates (NM), and it was expressed on a per day basis. Similarly, the net nitrification rates (NN) were calculated in the same way as the changes of nitrate concentrations.
Statistical analyses were carried out by SPSS 19.0 (SPSS, Inc., USA) plotted using OriginPro 8 (OriginLab, Inc., USA). Influences of diverse countermeasures on net and gross N transformation rates among all treatments for each soil were assessed by using least significant difference (LSD) test and ANOVA analysis.
Results
Net transformation rates
In JX soil, the net mineralisation (NM) and net nitrification rates (NN) were lower than which in SC soil (). The net mineralisation rates in the CK, NC, NS and NB treatments of JX soil were 0.62, −0.62, −48.72 and 0.52 mg kg−1 d−1, respectively, showing that the NS treatments decreased net mineralisation rate (p < 0.05) and the other two treatments had no obvious effects. Rice straw incorporation observably increased the net immobilisation rate of inorganic N, showed by both and
concentrations decreasing rapidly (Figure 1S(C, D) and Figure 2S(C, D)). Similar effects of nitrification inhibitor, rice straw and its biochar on the net mineralisation and net nitrification rates were observed in SC soil. The net mineralisation was also reduced in the NS treatment of the SC soil. The NC and NS treatments decreased the net nitrification rates compare with the CK treatment (p < 0.05), but the NB treatment had no effects on net nitrification rates in both two soils () (p > 0.05).
Figure 1. Net mineralisation and nitrification rates in JX and SC soils (CK) and those amended with nitrapyrin (NC), rice straw (NS) and biochar (NB). Error bars represent standard deviations. Lowercase letters indicate significant difference between treatments in each soil (p < 0.05). SC, soil collected from Sichuan Province; JX, soil collected from Jiangxi Province.
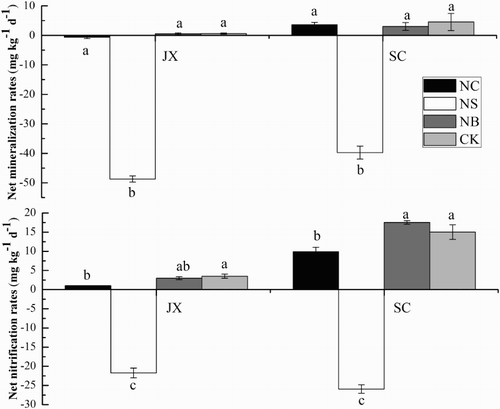
Gross N transformation rates
By running 15N tracing model developed by Müller et al. (Citation2007) with the inputs of measured and
concentrations (Figure 1S and Figure 2S) and their 15N enrichment (Figure 3S) during the 15N tracing incubation, ten gross N transformation rates simultaneously occurred in all treatments of JX and SC soils were quantified. The gross N transformation rates were shown in . The results indicated that the total gross N mineralisation rates (TM) in CK, NC, NS and NB treatments of SC soil were 8.05, 6.93, 37.45 and 45.32 mg kg−1 d−1, respectively. Compared with both CK and NC, TM rates in NS and NB were higher (p < 0.05), but there were no significant differences between the NC and CK treatments (p > 0.05) ((A)). The gross mineralisation rates in JX soil of CK, NC, NS and NB were 1.17, 8.21, 16.66 and 5.27 mg kg−1 d−1, respectively, which were lower than those in the SC soil of corresponding treatments. The gross mineralisation rates in the NC, NS and NB of JX soil were higher than that of the CK treatment (p < 0.05) and the highest rate was quantified in the NS treatment ((A)). In SC soil, the total gross
immobilisation rates (TI) could be ignored in the CK treatment and was very low in the NC treatment (only 0.1 mg kg−1 d−1), but high in the NS and NB treatments (47.90 and 46.96 mg kg−1 d−1, respectively) ((B)). On the contrary, the TI rates in the NC and NS treatments of JX soil (10.30 and 45.37 mg kg−1 d−1 respectively) were greater than that in the CK treatment (4.56 mg kg−1 d−1). However, the TI rate in the NB (9.04 mg kg−1 d−1) of JX soil was not different to CK ((B)). In addition, the tracing model showed the rates of release of adsorbed
in all treatments were negligible except the NS and NB in SC soil, and rates of adsorption of
in all were negligible except NS in JX and NB in SC soil ((G, H)). Biochar application promoted the rates of adsorption and release of
in SC soil ((G, H).
Figure 2. Gross N transformation rates in JX and SC soils (CK) and those amended with nitrapyrin (NC), rice straw (NS) and biochar (NB). Error bars represent standard deviations. Lowercase letters indicate significant difference between treatments in each soil (p < 0.05). SC, soil collected from Sichuan Province; JX, soil collected from Jiangxi Province. TM, total organic N mineralisation rate; TI, total immobilisation rate; INO3,
immobilisation rate; ONrec, heterotrophic nitrification rate of organic N; ONH4,
oxidation rate; DNO3, dissimilatory
reduction to
(DNRA); ANH4, adsorption of ammonium on cation exchange sites; RNH4, release of adsorbed ammonium.
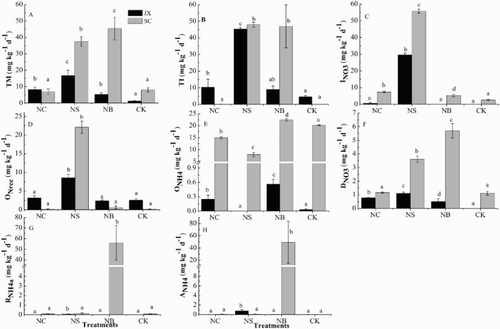
For the JX soil, was mainly produced via heterotrophic nitrification (oxidation of organic N). Gross heterotrophic nitrification rates in the four treatments ranged from 2.42 to 8.56 mg kg−1 d−1 and in the NS treatment this rate was dramatically greater than the other three treatments (p < 0.05) ((D)). However, the gross autotrophic nitrification rates in these four treatments were very low ((E)) and 98.8%, 92.7%, 100% and 81.1% of the
were produced via heterotrophic nitrification in the CK, NC, NS and NB treatments, respectively. In contrast, the gross autotrophic nitrification rates in the CK, NC, NS and NB treatments of SC soil were 20.22, 15.01, 7.94 and 22.49 mg kg−1 d−1, respectively ((E)). Particularly in the CK, NC and NB of SC soil, autotrophic nitrification accounted for 99.2%, 99.0% and 97.3% of
production, respectively. Whereas in the NS treatment, the gross heterotrophic nitrification rate (22.07 mg kg−1 d−1) was higher than the autotrophic nitrification rate (7.94 mg kg−1 d−1). From these results, all the treatments had no significant effects on gross heterotrophic nitrification rates of these two studied soils except for the NS treatment, which could significantly increase these rates.
Immobilisation of nitrate and DNRA were the two main nitrate consumption pathways in the two soils. In the nitrapyrin and straw treatment of the JX soil, the rates of gross immobilisation were 0.67 and 29.59 mg kg−1 d−1, respectively, but which in the CK and NB treatments were negligible ((C)). The gross
immobilisation rates in SC were higher than that of JX. The rates in SC soil were in the order CK (2.55 mg kg−1 d−1) < NB (5.27 mg kg−1 d−1) < NC (7.42 mg kg−1 d−1) < NS (55.81 mg kg−1 d−1) ((C)). The DNRA rates in the CK treatment of JX could be neglected while in the NC, NS and NB they were 0.78, 1.10 and 0.51 mg kg−1 d−1 respectively, all greater than in the CK ((F)). In NS and NB of SC soil, the DNRA rates were higher than the CK (p < 0.05), and the rates ranked as CK (1.12 mg kg−1 d−1) ≈ NC (1.16 mg kg−1 d−1) < NS (3.62 mg kg−1 d−1) < NB (5.69 mg kg−1 d−1) ((F)).
Discussion
The present study showed that the effects of nitrapyrin, rice straw and biochar on the net mineralisation rates and nitrification rates were similar in both studied soils. Net mineralisation rates were only affected significantly by the incorporation of rice straw and net nitrification rates decreased after nitrapyrin and rice straw application (). However, the effects of these three countermeasures on N transformation processes were not always same in both soils at the beginning stage according to the results obtained by the tracing model ().
Effects of countermeasures on N availability
Net mineralisation rates are the result of the interaction between various gross organic N mineralisation and inorganic N immobilisation rates that are responsible for the production and consumption of inorganic N. Nitrapyrin had no apparent influence on the net mineralisation rate in both soils. However, a different response of the gross N rates in these two soils was apparent. In the SC soil, nitrapyrin had no influence on either total gross N mineralisation or total gross immobilisation rate, while in the JX soil, nitrapyrin had a stimulatory response to both total gross N mineralisation and
immobilisation ((A)). These results point to an influence of nitrification inhibitor on microbial activity and not just nitrification. A stimulation of microbial activity through increase of CO2 was for instance observed by Smith and Weeraratna (Citation1975). Furthermore, it has been shown that soil gross N mineralisation rates are mainly related to microbial biomass/activity and total soil C and N concentrations (Ros Citation2012; Ernfors et al. Citation2014). Nitrapyrin can be degraded during the incubation period and provided sources of C and N (Chalk et al. Citation1990). The inconsistent effects of nitrapyrin on the total gross mineralisation rates and inorganic N immobilisation rates of SC and JX soils are most likely be attributable to the differences () in soil texture (Jarvis et al. Citation1996; McGeough et al. Citation2016), organic C and N contents and C/N ratio (Shen et al. Citation1984; Chalk et al. Citation1990).
It was not surprising that the incorporation of rice straw with higher C/N ratio significantly decreased the net N mineralisation rates in these two soils in the early stage (Chen et al. Citation2013). Incorporation of rice straw significantly increased the gross mineralisation rates (p < 0.05, (A)), due to the additional source of organic N provided by incorporated rice straw and/or the positive priming effect on the mineralisation of soil organic matter (Shindo and Nishio Citation2005). Thus, the high C availability in rice straw application supported more active microbial populations. The large C/N ratio in the rice straw could not meet N demand for the rapid growth of microbial populations in the early stage of incorporation, thus promoting immobilisation rates of both and
((B, C)) which is in accordance with previous studies (Recous et al. Citation1990). The gross immobilisation rate of inorganic N was greater than that of gross mineralisation, which resulted in the occurrence of net immobilisation. The reduction of inorganic N contents due to the addition of rice straw indicated that the available N shortage would occur to plant growth in the early growth stages (Chapman Citation1997). Therefore, fertiliser management should be adopted based on the N compound that is in minimum and should be applied alongside with straw incorporation to alleviate any N limitation.
Addition of biochar made by rice straw significantly enhanced the gross mineralisation rates of both JX and SC soils, probably due to the priming effect of biochar on recalcitrant soil organic N mineralisation (Nelissen et al. Citation2012). However, in JX soil the increased gross organic N mineralisation rate was traded off completely by simultaneously increased gross immobilisation rates, but not
immobilisation. Consequently, the change in net mineralisation was not significant statistically in JX soil (). In SC soil, biochar also stimulated both the gross rates of
and
immobilizations significantly () and the increases in both
and
immobilizations completely traded off the increased rate of mineralisation (). Our results demonstrate clearly that the different effects of biochar on soil N dynamics were at least partially due to different soil properties. Thus, biochar effects on the mineralisation-immobilisation turnover in soil should be evaluated within the context of the prevailing soil properties which is in accordance with the other researches (Smith et al. Citation2010; Verheijen et al. Citation2010; Cheng et al. Citation2012).
The abiotic mechanisms affecting soil inorganic N after biochar application include adsorption and desorption. Our results showed that biochar addition significantly increased the rates of adsorption and desorption of in SC soil but not in JX soils (). It was probably related to soil pH. The adsorption effect of biochar was dominant in the alkaline soil, while in acid soil the effect of biochar on soil pH promotion was stronger than its adsorption effect. The exact mechanism for
adsorption and desorption by biochar in different soils were not identified and needed to be further studied.
Effects of countermeasures on
dynamics
Nitrapyrin decreased the net nitrification rates in both JX and SC soils (), thus minimise the risk of leaching and runoff in the humid subtropical soils. This result is line with studies where nitrapyrin has been evaluated as a nitrification inhibitor (Smith and Weeraratna Citation1975; Parkin and Hatfield Citation2010; Fisk et al. Citation2015). In contrast to many other studies where the effect on the net nitrification rate has been studied, we identified that nitrapyrin inhibited the gross
oxidation rate (). Moreover, nitrapyrin addition did not significantly affect heterotrophic nitrification defined as organic N directly oxidised to
(Zhang et al. Citation2015a) in both studied soils (). The results were in agreement with previous observations that nitrapyrin did not inhibit a range of fungi and heterotrophic bacteria (Goring Citation1962; Shattuck and Alexander Citation1963). The autotrophic nitrification defined as the oxidation of
to
(Zhang et al. Citation2015a) was the predominant process of
production and its rate was high up to 20.22 mg N kg−1 soil d−1 in SC soil. The nitrapyrin addition decreased the gross autotrophic nitrification rate by 26%, compared with the CK treatment in SC soil, because nitrapyrin lead to the inhibitory effect on ammonia monooxygenase (AMO) (Vannelli and Hooper Citation1992). The decrease in net nitrification in SC soil by nitrapyrin addition was further supported by its stimulating effect on
immobilisation (). The effect of nitrapyrin on the gross rate of autotrophic nitrification was distinctly different between the two soils. While it was negligible in JX soil and even increased under the NC treatment. The gross
rate decreased, as expected, in the JX soil. Due to the small rate in the JX soil the increase, has no practical implications (). Furthermore, different from the stimulating effect of nitrapyrin on
immobilisation rate in SC soil, nitrapyrin addition did not significantly affect
immobilisation rate in JX soil. Taking into account all processes involved in
production and consumption, we identified that one major effect of nitrapyrin was stimulating the dissimilatory
reduction to
(DNRA) process that led to the decrease in net nitrification in JX soil. However, the underlying mechanism for nitrapyrin stimulating DNRA process in the acid soil in the humid tropical region needs to be further investigated.
Among the three countermeasures investigated in the study, incorporation of rice straw was most effective to reduce the net nitrification in both JX and SC soils (). Heterotrophic nitrification rates in both soils were stimulated by the rice straw incorporation () due to the increase in labile organic N from the rice straw (Zhang et al. Citation2015b). However, the gross autotrophic nitrification rate in SC soil was significantly decreased by the incorporation of rice straw (), most likely due to the competition of hydrocarbons in rice straw with ammonia to the ammonia monooxygenase, thus reducing the rate of ammonia oxidation (Deni and Penninckx Citation1999) and/or immobilisation through competition for
with autotrophic nitrification (Khalil et al. Citation2005). The high C/N ratio in rice straw stimulated not only
, but also
immobilisation rates in both soils (). As a result, the net nitrification rates in both soils decreased significantly by the incorporation of rice straw.
So far the effect of biochar on ammonia oxidation is not fully understood (Clough et al. Citation2013). Biochar application possibly does not affect gross or net nitrification rates in the agricultural soils (Castaldi et al. Citation2011; Cheng et al. Citation2012). However, it has also been reported to show a significant effect on both activity and abundance of ammonia oxidising bacteria in soil (Ball et al. Citation2010; Clough et al. Citation2010). Our results showed consistent effects of biochar on the net nitrification rate and the gross rates of production and consumption processes in both soils, which are characterised by different properties. The addition of biochar had no significant influences on the gross rates of heterotrophic nitrification, but increased the gross autotrophic nitrification rates, which were traded off by the increased DNRA process by biochar in the two studied soils ( and ).
Overall, our study found that, shortly after application of various soil additives, application of N fertilisers with nitrification inhibitor nitrapyrin, incorporation of rice straw, and addition of biochar stimulate a differential response with respect to the soil properties which varied greatly between the zonal acid soil (JX soil) and non-zonal alkaline soil (SC soil) in the humid subtropical China. There were similar and general trends that incorporation of rice straw decreased both net mineralisation and nitrification rates most intensively, nitrapyrin decreased the net nitrification rate, but did not affect the net mineralisation rate significantly, and biochar did not affect both net mineralisation and nitrification rates in both studied soil significantly, although their effects on individual N transformation processes varied with soils. Therefore, the application of soil additives to control the soil N dynamics in the humid subtropical soils should be selected according to the regulation targets and in view of the soil properties.
Disclosure statement
No potential conflict of interest was reported by the authors.
Notes on contributors
Ying Zhao is a Ph.D. candidate at the School of Geography Sciences, Nanjing Normal University. Her research is about soil nitrogen transformation.
Jing Wang is employed at the College of Forestry, Nanjing Forestry University. Her research is about soil nitrogen transformation.
Zucong Cai is a professor at the School of Geography Sciences, Nanjing Normal University. His main research topic is about the relationship between soil nitrogen cycling and global change, including the relationship between nitrification/denitrification and nitrogen oxides emissions, and agricultural non-point source pollution.
Christoph Müller is a professor at Justus-Liebig University Giessen. His main research areas are: effect of climate change on ecological processes, elemental cycles in terrestrial ecosystems, and production processes of climate-relevant trace gases.
Jinbo Zhang is a professor at the School of Geography Sciences, Nanjing Normal University. He is mainly engaged in the study of soil carbon and nitrogen biogeochemistry cycle, focusing on soil nitrogen transformation processes and the relationship with environmental changes.
Additional information
Funding
References
- Ball PN, MacKenzie MD, Deluca TH, Holben WE. 2010. Wildfire and charcoal enhance nitrification and ammonium-oxidizing bacterial abundance in dry montane forest soils. J Environ Qual. 39:1243–1253. doi: 10.2134/jeq2009.0082
- Bird JA, van Kessel C, Horwath WR. 2003. Stabilization of 13C-carbon and immobilization of 15N-nitrogen from rice straw in humic fractions. Soil Sci Soc Am J. 67:806–816. doi: 10.2136/sssaj2003.0806
- Bruun EW, Ambus P, Egsgaard H, Hauggaard-Nielsen H. 2012. Effects of slow and fast pyrolysis biochar on soil C and N turnover dynamics. Soil Biol Biochem. 46:73–79. doi: 10.1016/j.soilbio.2011.11.019
- Castaldi S, Riondino M, Baronti S, Esposito FR, Marzaioli R, Rutigliano FA. 2011. Impact of biochar application to a mediterranean wheat crop on soil microbial activity and greenhouse gas fluxes. Chemosphere. 85:1464–1471. doi: 10.1016/j.chemosphere.2011.08.031
- Chalk PM, Victoria RL, Muraoka T, Piccolo MC. 1990. Effect of a nitrification inhibitor on immobilization and mineralization of soil and fertilizer nitrogen. Soil Biol Biochem. 22:533–538. doi: 10.1016/0038-0717(90)90190-B
- Chapman SJ. 1997. Carbon substrate mineralization and sulphur limitation. Soil Biol Biochem. 29:115–122. doi: 10.1016/S0038-0717(96)00302-1
- Chen HH, Li CX, Hu F, Shi W. 2013. Soil nitrous oxide emissions following crop residue addition: a meta-analysis. Global Change Biol. 19:2956–2964. doi: 10.1111/gcb.12274
- Chen D, Suter HC, Islam A, Edis R. 2010. Influence of nitrification inhibitors on nitrification and nitrous oxide (N2O) emission from a clay loam soil fertilized with urea. Soil Biol Biochem. 42:660–664. doi: 10.1016/j.soilbio.2009.12.014
- Cheng Y, Cai Z-C, Chang SX, Wang J, Zhang J-B. 2012. Wheat straw and its biochar have contrasting effects on inorganic N retention and N2O production in a cultivated Black Chernozem. Biol Fert Soils. 48:941–946. doi: 10.1007/s00374-012-0687-0
- Clough TJ, Bertram JE, Ray JL, Condron LM, O’Callaghan M, Sherlock RR, Wells NS. 2010. Unweathered wood biochar impact on nitrous oxide emissions from a bovine-urine-amended pasture soil. Soil Sci Soc Am J. 74:852–860. doi: 10.2136/sssaj2009.0185
- Clough TJ, Condron LM. 2010. Biochar and the nitrogen cycle: introduction. J Environ Qual. 39:1218–1223. doi: 10.2134/jeq2010.0204
- Clough TJ, Condron LM, Kammann C, Müller C. 2013. A review of biochar and soil nitrogen dynamics. Agronomy. 3:275–293. doi: 10.3390/agronomy3020275
- Dempster DN, Gleeson DB, Solaiman ZM, Jones DL, Murphy DV. 2012. Decreased soil microbial biomass and nitrogen minerlisation with eucalyptus biochar addition to a coarse textured soil. Plant Soil. 354:311–324. doi: 10.1007/s11104-011-1067-5
- Deni J, Penninckx MJ. 1999. Nitrification and autotrophic nitrifying bacteria in a hydrocarbon-polluted soil. Appl Environ Microb. 65:4008–4013.
- Ernfors M, Brennan FP, Richards KG, Mcgeough KL, Griffiths BS, Laughlin RJ, Watson CJ, Philippot L, Grant J, Minet EP, et al. 2014. The nitrification inhibitor dicyandiamide increases mineralization-immobilization turnover in slurry-amended grassland soil. J Agr Sci-Cambridge. 152:S137–S149. doi: 10.1017/S0021859613000907
- Fangueiro D, Fernandes A, Coutinho J, Moreira N, Trindade H. 2009. Influence of two nitrification inhibitors (DCD and DMPP) on annual ryegrass yield and soil mineral N dynamics after incorporation with cattle slurry. Commun Soil Sci Plan. 40:3387–3398. doi: 10.1080/00103620903325976
- FAO-UNESCO-ISRIC. 1990. Soil Map of the World. Revised legend. World Soil Resources Report 60. Rome.
- Fisk LM, Maccarone LD, Barton L, Murphy DV. 2015. Nitrapyrin decreased nitrification of nitrogen released from soil organic matter but not amoA gene abundance at high soil temperature. Soil Biol Biochem. 88:214–223. doi: 10.1016/j.soilbio.2015.05.029
- Fontaine S, Barot S. 2005. Size and functional diversity of microbe populations control plant persistence and long-term soil carbon accumulation. Ecol Lett. 8:1075–1087. doi: 10.1111/j.1461-0248.2005.00813.x
- Goring CAI. 1962. Control of nitrification by 2-chloro-6-(trichloro-methyl) pyridine. Soil Sci. 93:211–218. doi: 10.1097/00010694-196203000-00010
- Hart SC, Nason GE, Myrold DD, Perry DA. 1994. Dynamics of gross nitrogen transformations in an old-growth forest: the carbon connection. Ecology. 75:880–891. doi: 10.2307/1939413
- Islam A, Chen D, White RE. 2007. Heterotrophic and autotrophic nitrification in two acid pasture soils. Soil Biol Biochem. 39:972–975. doi: 10.1016/j.soilbio.2006.11.003
- Jarvis SC, Stockdale EA, Shepherd MA, Powlson DS. 1996. Nitrogen mineralization in temperate agricultural soils: processes and measurement. Adv Agron. 57:187–235. doi: 10.1016/S0065-2113(08)60925-6
- Khalil MI, Hossain MB, Schmidhalter U. 2005. Carbon and nitrogen mineralization in different upland soils of the subtropics treated with organic materials. Soil Biol Biochem. 37:1507–1518. doi: 10.1016/j.soilbio.2005.01.014
- Lal R. 2005. World crop residues production and implications of its use as a biofuel. Environ Int. 31:575–584. doi: 10.1016/j.envint.2004.09.005
- Lehmann J, Joseph S. 2009. Biochar for environmental management: science and technology. London: Eatthscan.
- Li QQ, Luo YL, Wang CQ, Li B, Zhang X, Yuan DG, Gao XS, Zhang H. 2016. Spatiotemporal variations and factors affecting soil nitrogen in the purple hilly area of southwest China during the 1980s and the 2010s. Sci Total Environ. 547:173–181. doi: 10.1016/j.scitotenv.2015.12.094
- Lu RK. 2000. Soil Agro-chemical analyses. Beijing: Agricultural Technical Press of China. Chinese.
- Mcgeough KL, Watson CJ, Müller C, Laughlin RJ, Chadwick DR. 2016. Evidence that the efficacy of the nitrification inhibitor dicyandiamide (DCD) is affected by soil properties in UK soils. Soil Biol Biochem. 94:222–232. doi: 10.1016/j.soilbio.2015.11.017
- Moorhead DL, Sinsabaugh RL. 2006. A theoretical model of litter decay and microbial interaction. Ecol Monogr. 76:151–174. doi: 10.1890/0012-9615(2006)076[0151:ATMOLD]2.0.CO;2
- Muhammad S, Joergensen RG, Mueller T, Muhammad TS. 2007. Priming mechanism: soil amended with crop residue. Pak J Bot. 39:1155–1160.
- Müller C, Rütting T, Kattge J, Laughlin RJ, Stevens RJ. 2007. Estimation of parameters in complex 15N tracing models by Monte Carlo sampling. Soil Biol Biochem. 39:715–726. doi: 10.1016/j.soilbio.2006.09.021
- Nelissen V, Rutting T, Huygens D, Staelens J, Ruysschaert G, Boeckx P. 2012. Maize biochars accelerate short-term soil nitrogen dynamics in a loamy sand soil. Soil Biol Biochem. 55:20–27. doi: 10.1016/j.soilbio.2012.05.019
- Nishio T, Komada M, Arao T, Kanamori T. 2001. Simultaneous determination of transformation rates of nitrate in soil. Jarq-JPN Agr Res Q. 35:11–17. doi: 10.6090/jarq.35.11
- Ocio JA, Brookes PC, Jenkinson DS. 1991. Field incorporation of straw and its effects on soil microbial biomass and soil inorganic N. Soil Biol Biochem. 23:171–176. doi: 10.1016/0038-0717(91)90131-3
- Parkin TB, Hatfield JL. 2010. Influence of nitrapyrin on N2O losses from soil receiving fall-applied anhydrous ammonia. Agr Ecosyst Environ. 136:81–86. doi: 10.1016/j.agee.2009.11.014
- Recous S, Mary B, Faurie G. 1990. Microbial immobilization of ammonium and nitrate in cultivated soils. Soil Biol Biochem. 22:913–922. doi: 10.1016/0038-0717(90)90129-N
- Ros GH. 2012. Predicting soil N mineralization using organic matter fractions and soil properties: a re-analysis of literature data. Soil Biol Biochem. 45:132–135. doi: 10.1016/j.soilbio.2011.10.015
- Shattuck GE, Alexander M. 1963. A differential inhibitor of nitrifying microorganisms. Soil Sci Soc Am J. 27:600–601. doi: 10.2136/sssaj1963.03615995002700050039x
- Shen SM, Pruden G, Jenkinson DS. 1984. Mineralization and immobilization of nitrogen in fumigated soil and the measurement of microbial biomass nitrogen. Soil Biol Biochem. 16:437–444. doi: 10.1016/0038-0717(84)90049-X
- Shindo H, Nishio T. 2005. Immobilization and remineralization of N following addition of wheat straw into soil: determination of gross N transformation rates by 15N-ammonium isotope dilution technique. Soil Biol Biochem. 37:425–432. doi: 10.1016/j.soilbio.2004.07.027
- Smith JL, Collins HP, Bailey VL. 2010. The effect of young biochar on soil respiration. Soil Biol Biochem. 42:2345–2347. doi: 10.1016/j.soilbio.2010.09.013
- Smith MS, Weeraratna CS. 1975. Influence of some biologically active compounds on microbial activity and on the availability of plant nutrients in soils II. Nitrapyrin, dazomet, 2-chlorobenzamide and tributyl-3-chlorobenzylammonium bromide. Pestic Sci. 6:605–615. doi: 10.1002/ps.2780060608
- Song Y, Zhang X, Ma B, Chang SX, Gong J. 2014. Biochar addition affected the dynamics of ammonia oxidizers and nitrification in microcosms of a coastal alkaline soil. Biol Fert Soils. 50:321–332. doi: 10.1007/s00374-013-0857-8
- Vannelli T, Hooper AB. 1992. Oxidation of nitrapyrin to 6-chloropicolinic acid by the ammonia-oxidizing bacterium Nitrosomonas europaea. Appl Environ Microb. 58:2321–2325.
- Verheijen FGA, Jeffery S, Bastos AC, Velde M, Diafas IVD. 2010. Biochar application to soils: a critical scientific review of effects on soil properties, processes and functions. Luxembourg: Office for the official publications of the European Communities, 149.
- Wang J, Zhu B, Zhang JB, Müller C, Cai ZC. 2015. Mechanisms of soil N dynamics following long-term application of organic fertilizers to subtropical rain-fed purple soil in China. Soil Biol Biochem. 91:222–231. doi: 10.1016/j.soilbio.2015.08.039
- Yang X-L, Zhu B, Li Y-L. 2013. Spatial and temporal patterns of soil nitrogen distribution under different land uses in a watershed in the hilly area of purple soil, China. J Mt Sci-Engl. 10:410–417. doi: 10.1007/s11629-013-2712-7
- Zhang JB, Müller C, Cai ZC. 2015a. Heterotrophic nitrification of organic N and its contribution to nitrous oxide emissions in soils. Soil Biol Biochem. 84:199–209. doi: 10.1016/j.soilbio.2015.02.028
- Zhang JB, Wang J, Zhong WH, Cai ZC. 2015b. Organic nitrogen stimulates the heterotrophic nitrification rate in an acidic forest soil. Soil Biol Biochem. 80:293–295. doi: 10.1016/j.soilbio.2014.10.024
- Zhang JB, Zhu TB, Meng TZ, Zhang YC, Yang JJ, Yang WY, Müller C, Cai ZC. 2013. Agricultural land use affects nitrate production and conservation in humid subtropical soils in China. Soil Biol Biochem. 62:107–114. doi: 10.1016/j.soilbio.2013.03.006
- Zhao X, Wang JW, Wang SQ, Xing GX. 2014. Successive straw biochar application as a strategy to sequester carbon and improve fertility: a pot experiment with two rice/wheat rotations in paddy soil. Plant Soil. 378:279–294. doi: 10.1007/s11104-014-2025-9
- Zhou MH, Zhu B, Butterbach-Bahl K, Wang T, Bergmann J, Brüggermann N, Wang ZH, Li TK, Kuang FH. 2012. Nitrate leaching, direct and indirect nitrous oxide fluxes from sloping cropland in the purple soil area, southwestern China. Environ Pollut. 162:361–368. doi: 10.1016/j.envpol.2011.12.001
- Zhu B, Wang T, Kuang FH, Luo ZX, Tang JL, Xu TP. 2009. Measurements of nitrate leaching from a hillslope cropland in the central Sichuan basin, China. Soil Sci Soc Am J. 73:1419–1426. doi: 10.2136/sssaj2008.0259