ABSTRACT
This study aims to highlight the beneficial effect of the phosphorus on enhancing of growth plant, the efficiency of use rhizobial symbiosis and ionic partition in chickpea grown under salt stress. Exposure of plants to salt stress (0, 150 mM of NaCl) caused ionic imbalance, which resulted in increased Na+ and P and reduced K+ contents in the leaves and root. Indeed, stressed plants showed decrease of plant growth and phosphorus use efficiency. The efficiency use of rhizobial symbiosis was also affected by salinity. However, addition of two different level of phosphorus (37 and 55 mM) to saline soil increased significantly availability of P in plant organs. Specially, the (150 mM NaCl × 37 mM P) mixture increased (33%) phosphorus use efficiency, induced better nodulation and increased plant biomass which results in the high efficiency in use of the rhizobial symbiosis. Our findings suggest that the combination of low level of P to saline soil presumably improved the tolerance of chickpea plant to salinity.
Abbreviations: phosphorus (P); phosphorus use efficiency (PUE); biological nitrogen fixation (BNF); plant dry weight (PDW); yeast extract mannitol (YEM); efficiency in use of the rhizobial symbiosis (EURS); shoot dry weight (SDW); symbiotic nitrogen fixation (SNF).
Introduction
Symbiotic nitrogen (N2) fixation of legume-Rhizobium couple plays an important role in sustainable nitrogen management in agriculture. The nitrogen fixed by the root nodules affects the nitrogen cycle of nature and have great economic importance. For more than 100 years, biological nitrogen fixation (BNF) has commanded the attention of scientists concerned with plant mineral nutrition, and it has been exploited extensively in agricultural practice (Burris Citation1994). The BNF is of great practical importance because the use of nitrogenous fertilisers has resulted in unacceptable levels of water pollution (increasing concentrations of toxic nitrates in drinking water supplies) and the eutrophication of lakes and rivers (Sprent and Sprent Citation1990).
Cicer arietinum L. (chickpea) is a symbiotic species cultivated worldwide. Its grain is considered an important source of proteins (25.3%–28.9%) for human food (Hulse Citation1991). However, growth, nodulation and nitrogen fixation of this species is adversely affected by saline soil which generated by irrigated agriculture. Of nearly, 160 million ha of cultivated land under irrigation worldwide, about one-third is already affected by salt, which makes salinity a major constraint to food production (Manchanda and Garg Citation2008). Saline soils occupy 3.2 million hectares in Algeria (Le Houérou Citation1993) whose 1200 ha are subject to irrigation system. Numerous studies showed that salt stress (i) reduces water potential and growth plant (Munns Citation2002; Benlloch-Gonzalez et al. Citation2005), (ii) creates ion imbalance and nutrient deficiency (Hassanein Citation2000; Tejera et al. Citation2006) and (iii) causes osmotic stress and ion toxicity (Munns Citation2005) in plant. The harmful influence of salinity on nodulation, nodule size and N2 fixation, also increases with the increase in concentration, according to the studies held by Swaraj and Bishnoi (Citation1999), Flowers et al. (Citation2010).
The chickpea production is also limited by large variability of yields which is often due to low content of phosphorus (P) of the soil where is grown particularly in under Mediterranean and tropical climates (Alkama et al. Citation2012). Phosphorus (P) is a major soil nutrient limiting crop production because of its high P fixation binding to the solid phase (Kideo and Kubicki Citation2004) and low diffusion coefficiency (Pypers et al. Citation2006). The efficiency of phosphorus can be low in the soil, with only 10%–30% of the P applied available for plant uptake in the year of application (Lynch and Beebe Citation1995). Plant species response to P deficiency by different physiological and/or morphological adaptation mechanisms for improving nutrient use efficiency under infertile soils (Romheld Citation1988). These mechanisms include acidification of rhizosphere by root exudates of organic acid, protons and phosphatases, whose role is to dissolve insoluble P of the soil (Tang et al. Citation2004; Alkama et al. Citation2012). To increase the absorption surface, root hair and density of the root resulting in more exploration of the soil volume (Vance Citation2001). In P deficiency conditions, legumes respond by optimising the metabolic phosphorus use efficiency (PUE) by producing more biomass per unit of P consumed fixing and more nitrogen per unit of nodule P so the PUE for symbiotic nitrogen fixation (Vadez and Drevon Citation2001). In light of this, the objective of this study was to evaluate the effect of two phosphorus concentrations in moderating toxic effects of salt stress on plant growth, efficiency use of rhizobial symbiosis, phosphorus use efficiency and to determine the extent of tolerance to salinity in Cicer arietinum L.
Materiel and methods
Site description and growth conditions
Field experiments were conducted using chickpea cultivar Flip 84-79C selected by ICARDA at the Experimental Farm of ITGC (Institut Technique des Grandes Cultures) Algiers, Algeria (Altitude: 24 m, Latitude: 36° 43′ North, Longitude: 30° 84″ East). Climatically, the area is in the sub-humid zone with mean annual temperature across the sites ranged between 10 and 30°C, and mean annual rainfall is about 672 mm with maximum rainfall in the period between November and February. Field trials were conducted in March and harvested in late July during the 2010–2012 cropping season (5 months). Physical and chemical parameters of soil collected to a depth of 0 cm to 30 cm and characterised on base of many standard methods (). The soil is silt-clay and initial pH of the soil was measured with a soil/water 1/5 (v/v). Electrical conductivity (EC) was determined with a conductivimeter using an aqueous extract of soil. The soil available P (Olsen P) was determined belong Olsen et al. (Citation1954). Rhizobia cultures in agar YEM (Yeast Extract Mannitol) revealed that the soil have good biological activity with sufficient Rhizobia content (Vincent Citation1970).
Table 1. Chemical and physical proprieties of the soil before sowing.
Experimental design was a randomised complete block design with three replicates on size plot of (3 m × 6 m). The plot was divided in subplot was 1 m × 1 m having four rows. In each subplot, the chickpea crop was sown with row to row distance of 0.20 m, plant to plant distance of 0.10 m and plant density was 40 plants per subplot. The main plot treatments consisted of three phosphorus fertiliser rates (P) including control: 0, 90, 200 kg h−1 of P2O5 in the form of super triple phosphate (STP) corresponding to 37 and 55 mM of P respectively and salt stress with two concentrations (S): 0 and 150 mM of NaCl. All solutions were prepared in distilled water. In Algeria, the chickpea crop depends on efficient application of 200 kg P2O5 ha–1 for optimal crop production. However, preliminary tests showed that the dose of 90 kg h−1 P had a positive effect on growth and symbiotic efficiency of chickpea cultivar Flip 84-79C. Similarly studies have shown that the application of 90 kg P2O5 ha−1 was sufficient to improve biomass and resulted in maximum grain yield of chickpea (Abdul Basir et al. Citation2008; Islam et al. Citation2012). The 150 mM of NaCl is often found in saline soils of Algeria (Djili Citation2000). There were six treatments having different combinations of phosphorus and NaCl levels: Control, P1 (37 mM P), P2 (55 mM P), S (150 mM NaCl), SP1 (S + 37 mM P), and SP2 (S + 55 mM P). During the first 2 weeks and before the development of functional nodules, irrigation requirement of crop was fulfilled once a week with different nutrients solutions to ensure 100% of the water needs of the plant and avoid water stress.
Plant harvest and statistical analysis
Plant growth parameters
A total of three plants in each subplot were randomly selected at 40 days after sowing (DAS) and dried at 70C°. Dry weight of the plants was measured before to separate them into shoots and nodulated roots. Roots and nodules were carefully separated from rhizospheric soil, washed and then the nodules were detached. Nodulation of plants is estimated by counting the number formed nodule per root (Alkama et al. Citation2012).
Mineral analysis and PUEI
Available and total P were analyzed by the molybdate blue method by reading the absorbance at 820 nm after colour development at 100°C for 10 min (Murphy and Riley Citation1962). For sodium (Na+) Potassium (K+) contents determination, samples (0.5 g) of dried shoots and nodules were dissolved in nitric acid (0.5% HNO3). This solution was diluted with distilled water and filtered on Whatman paper. The mineral contents were determined by flame emission photometry (Ghoulam et al. Citation2002).
Phosphorus use efficiency indexes
Shoot dry matter yield (SDM) and total P content of the plant organs were used to calculate the Phosphorus Efficiency Index PUEI (SDM2 / total P content) of plants (Siddiqi and Glass Citation1981; Furlani et al. Citation2001).
Efficiency use of rhizobial symbiosis
In order to assess the effect of P supply with or without salinity on plant growth and symbiotic efficiency, the efficiency in utilisation of the rhizobial symbiosis (EURS) was estimated by the slope of the regression model (i.e. y = ax + b) of shoot biomass as a function of nodule biomass (Alkama et al. Citation2012).
The data were analyzed by analyses of variance (ANOVA) performed using STATISTICA version 6.0. The significance of the treatment effect was determined using Post-hoc test. When ANOVA indicated that there was a significant value, comparisons of mean values were performed using the least significant difference method (LSD).
Results
Response of chickpea cultivar Flip 84-79C to P treatment combined or no to salinity was investigated. Dry biomass varied significantly from 2.64 to 4.57 g plant−1 under non-saline condition as function of P level applied, and from 2.43 to 3.04 g plant−1 under salinity with or without P condition ((A)). Generally, under saline and non-saline conditions the low phosphorus application (37 mM) increased significantly the dry biomass but (55 mM) P concentration decreased the dry biomass compared to control.
Figure 1. Effect of phosphorus fertilisation and sodium combination (NaCl deficiency with dark bars versus NaCl addition with gray bars) on plant dry weight (A) and nodules dry weight (B) on 60 days old C. arietinum L. cv Flip 84-79C cultivated in the field. Bars represent ± SD of three replicates. Mean comparisons among treatments are the small letters. Means followed by one common letter are not significantly different in the LSD test (p ≤ .05).
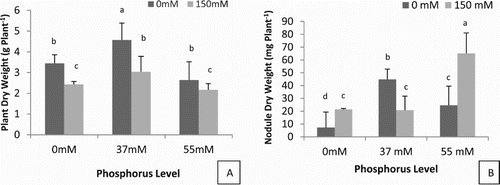
The results indicated that without a salt, a both phosphorus doses (P1 and P2) significantly decreased the total nodules number by 50% while the nodule biomass was significantly increased compared to control ((B)). The SP1 (37 mM P) plants presented the highest nodule number reaching 35 nodules plant−1 and SP2 highest nodule biomass (65 mg plant−1 than salinity (150 mM of NaCl). However, no significantly difference was observed in nodule biomass between S, SP1 and P2.
The effect of phosphorus fertiliser and/or the salinity, on the efficiency of use of the Rhizobial symbiosis (EURS) was evaluated by the values of the shoot biomass was plotted as a function of those of the nodule biomass (). The slope value of the regression curve, namely the EURS, was higher under conditions of SP treatments (84%) than under control and salinity conditions by 49% and 46% respectively, in contrast, to the both (37 mM P) and (55 mM P) induced the lowest EURS (15%) than other treatments. The biomass of shoots and nodules of the P, SP and S plants were positively correlated by r2 = 0.13, r2 = 0.68 and r2 = 0.65 respectively. However, the plants control presented negative correlation coefficient by r2 = −0. 98 ().
Figure 2. Effect of phosphorus fertilisation and sodium combination (control with gray squares; P with phosphorus white squares; NaCl alone with gray triangles; mixture NaCl × P with dark squares) on efficiency of use of the rhizobial symbiosis (EURS) on 60 days old C. arietinum L. cv Flip 84-79 cultivated in the field. The shoot was separated from the root at the cotyledonary node, and then weighed after 48 h at 70°C. Nodules were separated from the roots, counted and weighed separately. The equations inserted in the graphs describe the regression function (y = ax + b) with (x: nodule dry weight and y: shoot dry weight, the slope corresponds to EURS; the R2 corresponds to the coefficient of correlation between shoot dry weight and nodule dry weight.
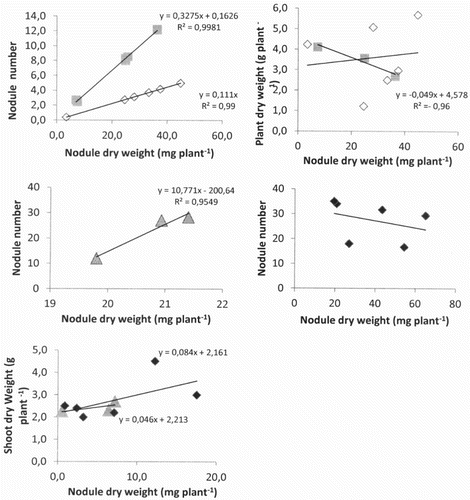
Sodium and potassium accumulation
illustrates that all treatments induced Na+ accumulation 3 to 4 folds higher in root than shoot whereas, the combination (S × 37 mM P) induced the lowest Na+ accumulation in leaves and root reaching 14 and 44 mg.g−1 respectively. These results indicate that this treatment might be able to protect plant parts against Na+ ions. Highest sodium accumulation in roots was found under salinity and P1 treatment (12.4 and 16.9 mM. G−1) compared to remaining cases. In contrast, in spite of their close association with the root, the lowest Na+ accumulation in shoot was observed under both (S × 37 mM P) and (37 mM P) treatments. The potassium accumulation increased slightly in shoot than root. The salinity induced high accumulation of K+ ion in the shoot nearly the same in control and SP1 treatment. In contrast, at (S × 37 mM P) combination the K+ concentration was less than 50% in root ().
Table 2. Effect of phosphorus fertilisation and sodium combination on sodium (Na+) and potassium contents (K+) in leaves and roots, 60 days old of C. arietinum L. cv Flip 84-79C. Data are means ± standard deviation of three replicates.
Phosphorus content and P use efficiency indexes
The significant different was noted in P content between plant and treatments (). Control and SP2 plants accumulated less amount of phosphorus while the other treatments induced increment P content in total plant. Generally, the highest P content in leaves was determined in P1 and low P level combined to salt whereas the lowest P content was determined in control, P2 and salinity conditions. In addition, the phosphorus use efficiency indexes (PUEI) was minimised by salt stress, while PUEI attained higher level under SP1, P1 and control treatments reaching respectively 0.43 and 0.32 g2 mg−1 .
Table 3. Effect of phosphorus fertilisation and sodium combination on phosphorus content (P) in plant organs and phosphorus use efficiency indexes (PUEI), 60 days old of C. arietinum L. cv Flip 84-79C. Data are means ± standard deviation of three replicates.
Discussion
The dry biomass of plants was higher under non-saline than saline condition. Salinity reduced whole plant growth about 30% than control and 50% compared to SP plants ((A)). Similar studies have revealed that salt stress limits plant productivity in legumes through diminished photosynthetic efficiency, nitrogen fixation and carbon metabolism (Ferri et al. Citation2000; Garg and Singla Citation2004). The fresh and dry weights of the shoot system are affected, either negatively or positively, by changes in salinity concentration, type of salt present, or type of plant species (Bayuelo Jimenez et al. Citation2002; Taffouo et al. Citation2010; Abdul Qados Citation2011).
The data indicated that phosphorus alone had pronounced effect on plant dry biomass as well as SP combinations. Among the fertilisers doses, phosphorus level 37 mM was found to be the best rate for plant growth (48%) more than other treatments ((A)). In addition, the lowest P supply showed the high dry biomass under salinity while the lowest dry biomass where observed with high soil available P under salinity. This result may show that low P application improved significantly the plant growth of chickpea cv Flip 84-79C.
Similarly phosphorus levels increased nodulation and nodule biomass than that of control and salinity treatments. The maximum (34) numbers of nodules plant−1 were recorded from those plots which were fertilised with 37 mM P and 55 mM P alone and with salinity, while minimum (21) were noted from salinity alone and control treatments ( ). Inhibitor effect of salinity on nodulation has been attributed to decrease in rhizobial colonisation and shrinkage and lack of root hair formation (Swaraj and Bishnoi Citation1999; Mudgal et al. Citation2010).). In accordance with nodule biomass growth, no significant changes were observed between S, P2 and SP1. The SP2 combination improved the nodule mass compared to control nodule which presented the lowest nodule dry weight ((B), 2). These results agree with those reported by Abdul Basir et al. (Citation2008) who concluded that phosphate sources significantly increased nodulation and growth of the crop. High nodulation in SP plants can be explained by the perfect protection of earlier establishment of new nodules against the accumulation of ions Na+ and Cl−, these results confirm those previously found by Sadji-Ait kaci et al. (Citation2017) on improve of nodule physiology under salinity condition by phosphorus supply.
Generally, the ions variation in organs has been associated with tolerance of legume to salt stress (Tejera et al. Citation2006). Salinity increased ions (Na+, K+) concentration in plant organs (). However, both ions accumulation decreased under control and SP1 combination. Indeed, the Na+ uptake increased 2-fold than K+ uptake, suggesting that a competition occur between Na and K absorption in the plants resulting in a Na/K antagonism (Benlloch-Gonzalez et al. Citation2005). This result suggest that under (37 mM P x salinity) the Na+ uptake was more affected in root as a result in nodule (data not shown) thus the P fertiliser might reduce the ionic toxicity due to salinity. Results showed that salt stress and SP2 treatments increased about 50% the P content in shoot and root but the P use efficiency (PUE) was strongly affected. However, the control and SP1 plant showed high phosphorus use efficiency (PUE) whereas; the P at (55 mM × 150 mM of NaCl) decreased the PUE of plants (). This result indicated the increase of phosphorus uptake by the plant which in turn would result in more energy available for nitrogen fixation by Rhizobia (Fitter and Garbaye Citation1994). The better tolerance of SP1 and SP2 plants to salinity was observed compared to stressed plants and it related to greater phosphorus use efficiency (PUE) that is illustrated by superior growth of shoot, roots and high nodulation. It was also indicated that the shoot growth and N2 fixation were determined mainly by the efficiency in P utilisation (Rodino et al. Citation2009).
Based on shoot dry weight and nodule biomass of P-fertilized plants, the symbiotic efficiency (EURS) is measured by the plant biomass/nodule mass rate (Purcino et al. Citation2000). EURS was increased in chickpea under phosphorus supply with or without salinity, this increased was highly observed with (S × P) interaction compared to salinity (). It is well known that the efficiency of symbiotic nitrogen fixation shows a high sensitivity to salinity expressed by a reduction in soil survival of Rhizobia, nodule number and weight, nitrogenase activity, and thus reducing the symbiotic nitrogen fixation (Rai Citation1992). Addition of phosphorus fertiliser to saline soil (SP) increased the slope (EURS) of regression curve (84%) and the correlation of plant dry matter with nodule biomass (64%) compared to salinity (46%), P and control conditions. These results confirm the reliability of the tested parameters as an indicator for efficiency in nitrogen fixation. High EURS suggested the nodule P requirement probably in relation to the high energy requirement of the symbiotic nitrogen fixation (SNF) process. Improve of symbiotic performance under (SP) interaction confirmed previous results obtained for mineral composition. As for that, showed (Na+) concentration reduced in leaves and root. In addition, the K+ content reduced with (23%) in plant organs under (S × P) mixture compared to salinity. The adverse effect of soil salinity on the growth and development of crops can be due to poor soil physical conditions and to nutritional imbalance and interference with uptake of essential nutrients or sodium toxicity (Mohammad et al. Citation1998; Tejera et al. Citation2006). It was found that the plant root growth under osmotic stress conditions was stimulated by localising the P fertilisers in the root zone (Mohammad et al. Citation1998). The present study provides additional evidence that symbiotic traits could be improved in chickpea by improving the quality of the soil by adding phosphorus in comparison to salinity alone, because increase mineral nutrient levels in the plants would not only benefit for Rhizobia infection, but would also lead to increased photosynthesis, making a greater proportion of photosynthates available to symbiotic nitrogen fixation.
In the current study, according to our results, the plants dry matter and nodulation decreased as the salinity increased whereas the sodium and phosphorus content increased in plant organs but phosphorus use efficiency decreased under salt stress. Nevertheless, the addition of two levels of phosphorus fertiliser influences the response of our plants cultivar growing under salinity. Particularly the low concentration added to saline soil might enhance salinity tolerance and efficiency use of rhizobial symbiosis by increase of phosphorus use efficiency. Indeed, this combination could reduce of adverse effect of salinity on plant growth. In addition, these results suggested that application of phosphorus to saline soil can result in the improvement of soil quality and long term sustainability of degraded soils.
Disclosure statement
No potential conflict of interest was reported by the authors.
Notes on contributors
H. Sadji-Ait Kaci is a lecturer in the Biology Department at the University of Sciences and Technology Houari Boumediene. Algeria. She is currently a Ph.D. candidate in soil microbiology at USTHB University, Algeria.
A. Chaker-Haddadj has obtained Ph.D. in plant symbiosis. Presently, she is lecturer in cell biology at the University of Sciences and Technology Houari Boumediene, Algeria. She has published research articles in plant symbiosis.
F. Aid is professor in the Biology Department at the University of Sciences and Technology Houari Boumediene, Algeria. She has published numerous research articles in plant physiology and presently she is working on abiotic stress tolerance of plant in USTHB, Algeria.
References
- Abdul Qados AMS. 2011. Effect of salt stress on plant growth and metabolism of bean plant Vicia faba (L.). J Saudi Soc Agric Sci. 10:7–15.
- Alkama N, Ounane G, Drevon JJ. 2012. Is genotypic variation of H+ efflux under P deficiency linked with nodulated-root respiration of N2–Fixing common-bean (Phaseolus vulgaris L.)? J Plant Physiol. 169:1084–1089. doi: 10.1016/j.jplph.2012.03.013
- Basir A, Shah Z, Naeem M, Bakht D, Khan ZH. 2008. Effect of phosphorus and farm yard manure on agronomic traits of chickpea (Cicer arietinum L.). Sarhad J Agric. 24(4):561–572.
- Bayuelo Jimenez JS, Debouk DG, Lynch JP. 2002. Salinity tolerance in phaseolus species during early vegetative growth. Crop Si. 42:2184–2192. doi: 10.2135/cropsci2002.2184
- Benlloch-Gonzalez M, Fournier J, Ramos J, Benlloch M. 2005. Strategies underlying salt tolerance in Halophytes are present in Cynara carduncullus. Plant Sci. 168:653–659. doi: 10.1016/j.plantsci.2004.09.035
- Burris RH. 1994. Biological nitrogen fixation-past and future. In: Hegazi NA, Fayez M, Monib M, editor. Nitrogen fixation with non-legumes. Cairo, Egypt: The American University in Cairo Press; p. 1–11.
- Djili K. 2000. Contribution à la connaissance des sols du Nord de l’Algérie [Thèse de doctorat]. Alger: INA; p. 243.
- Ferri A, Lluch C, Ocana A. 2000. Effect of salt stress on carbon metabolism and bacteroid respiration in root nodules of common bean (Phaseolus vulgaris L.). Plant Biol. 2:396–402. doi: 10.1055/s-2000-5956
- Fitter AH, Garbaye J. 1994. Interactions between mycorrhizal fungi and other soil organisms. Plant Soil. 159:123–132. doi: 10.1007/BF00000101
- Flowers TJ, Gaur PM, Gorda CL, Krichnamurthy L, Samineni S, Siddique KHM, Turner NC, Vadez V, Vashney RK, Colmer TD. 2010. Salt Sensivity in chickpea Rreview. Plant Cell Env. 1365:3040–3060.
- Furlani AC, Furlani PR, Tanaka RT, Mascarenhas HA, Delgado MP. 2001. Ariability in soybean germplasm for phosphorus uptake and use efficiency for dry matter yield. Dev Plant Soil Sci. 92:74–75.
- Garg N, Singla R. 2004. Growth, photosynthesis, nodule nitrogen and carbon fixation in the chickpea cultivars under salt stress. Braz J Plant Physiol. 16 (3):137–146. doi: 10.1590/S1677-04202004000300003
- Ghoulam C, Foursy A, Fares K. 2002. Effects of salt stress on growth, inorganic ions and proline accumulation in relation to osmotic adjustment in five sugar beet cultivars. Environ Exp Bot. 47:39–50. doi: 10.1016/S0098-8472(01)00109-5
- Hassanein AA. 2000. Physiological responses induced by shock and gradual salinization in rice (Oryza sativa L.) seedlings and the possible roles played by glutathione treatment. Acta Bot Hung. 42:139–159.
- Hulse JH. 1991. Nature, composition and utilization of grain legumes. Dans Uses of Tropical Legumes. Proceeding of Consulting Meeting, 27-30 March 1989. Édition Patencheru; ICRISAT Center, India, p. 502–524.
- Islam M, Mohsan S, Ali S, Khalid R, Afzal S. 2012. Response of chickpea to various levels of phosphorus and sulphur under rainfed conditions in Pakistan. Roman Agri Res. 29:175–183.
- Kideo KD, Kubicki JD. 2004. Molecular orbital theory study on surface complex structure of phosphates on iron hydroxides: Calculation of vibrational frequencies and adsorption energies. Langmuir. 20:9249–9254. doi: 10.1021/la0487444
- Le Houérou HN. 1993. Salt-tolerance plants for the arid region of the Mediterranean isoclimatic zone. In: Leith H, Al Massoom A, editor. Towards the rational use of high salinity tolerant plants; p. 403–422.
- Lynch JP, Beebe SE. 1995. Adaptation of beans (Phaseolus vulgaris L.) to deficient phosphorous availability. Hortsci. 30:1165–1171.
- Manchanda G, Garg N. 2008. Salinity and its effects on the functional biology of legumes. Acta Physiol Plant. 30:595–618. doi: 10.1007/s11738-008-0173-3
- Mohammad M, Shibli R, Ajouni M, Nimri L. 1998. - Tomato root and shoot responses to salt stress under different levels of phosphorus nutrition. J Plant Nutr. 21:1667–1680. doi: 10.1080/01904169809365512
- Mudgal V, Madaan N, Mudgal A, Singh A, Kumar P. 2010. Comparative Study of the Effects of Salinity on Plant Growth, Nodulation, and Legheamoglobin Content in Kabuli and Desi Cultivars of Cicer arietinum (L.). KBM J Biol. 1:1–4.
- Munns R. 2002. Comparative physiology of salt and water stress. Plant, Cell Environ. 20:239–250. doi: 10.1046/j.0016-8025.2001.00808.x
- Munns R. 2005. Genes and salt tolerance: bringing them together. New Phytol. 167:645–663. doi: 10.1111/j.1469-8137.2005.01487.x
- Murphy J, Riley JP. 1962. A modified single solution method for the determination of phosphate in natural waters. Anal Chim Acta. 27:31–36. doi: 10.1016/S0003-2670(00)88444-5
- Olsen SR, Cole CV, Watanabe FS, Dean LA. 1954. Estimation of available phosphorus in soil by extraction with Sodium Bicarbonate; Circular 939. Washington (DC, USA): USDA.
- Purcino HMA, Festin PM, Elkan GH. 2000. Identification of effective strains of Bradyrhizobium for Arachis pintoi. Trop Agric. 77(4):226–231.
- Pypers PJ, Delrue J, Diels E, Smolders MR. 2006. Phosphorus intensity determines short-term P uptake by pigeon pea (Cajanus cajan L.) grown in soils with differing P buffering capacity. Plant Soil. 284:217–227. doi: 10.1007/s11104-006-0051-y
- Rai R. 1992. Effect of nitrogen levels and Rhizobium strains on symbiotic N2 fixation and grain yield of Phaseolus vulgaris L. genotypes in normal and saline-sodic soils. Biol Fertil Soils. 14:293–299. doi: 10.1007/BF00395466
- Rodino AP, Metrae R, Guglielmi S, Drevon JJ. 2009. Variation among common-bean accessions (Phaseolus vulgaris L.) from the Iberian Peninsula for N2-dependent growth and phosphorus requirement. Symbiosis. 47:161–174. doi: 10.1007/BF03179976
- Romheld V. 1988. The soil root interface: Its relationship to nutrient availability and plant nutrition. Res Inst Pomol Floricult. 41–58.
- Sadji-Ait kaci H, Chaker-Haddadj A, Aid F. 2017. Interactive effects of salinity and two phosphorus fertilizers on growth and grain yield of Cicer arietinum L. Acta SCAND B-S P. 67(3):208–216.
- Siddiqi MY, Glass AM. 1981. Utilization index: A modified Approach to estimation and comparison of nutrient utilization efficiency in plants. J Plant Nutr. 4:289–302. doi: 10.1080/01904168109362919
- Sprent JI, Sprent P. 1990. Nitrogen fixing organisms. Pure and applied aspects. London (UK): Chapman & Hall.
- Swaraj K, Bishnoi NR. 1999. Effect of salt stress on nodulation and nitrogen fixation in legumes. Indian J Exp Biol. 37 (9):843–848.
- Taffouo VD, Wamba OF, Yombi E, Nono GV, Akoe A. 2010. Growth, yield, water status and ionic distribution response of three bambara groundnut (Vigna subterranean (L.) verdc.) landraces grown under saline conditions. Int J Bot. 6(1):53–58. doi: 10.3923/ijb.2010.53.58
- Tang C, Drevon JJ, Jaillard B, Souche G, Hinsinger P. 2004. Proton efflux of two genotypes of bean (Phaseolus vulgaris L.) as affected by nitrogen nutrition and phosphorus sub-deficiency. Plant Soil. 260:59–68. doi: 10.1023/B:PLSO.0000030174.09138.76
- Tejera NA, Soussi M, Llunch C. 2006. Physiological and nutritional indicators of tolerance to salinity in chickpea plants growing under symbiotic condition. Env Exp Bot. 58:17–24. doi: 10.1016/j.envexpbot.2005.06.007
- Vadez V, Drevon JJ. 2001. Genotypic variability in P use efficiency for symbiotic N2 fixation in common-bean (Phaseolus vulgaris L.). Agronomie. 21:691–699. doi: 10.1051/agro:2001162
- Vance C. 2001. Symbiotic nitrogen fixation and phosphorus acquisition, plant nutrition in world of declining renewable resources. Plant Physiol. 127:390–397. doi: 10.1104/pp.010331
- Vincent JM. 1970. A Manual for the Practical Study of Root-nodule Bacteria. I.B.P. Handbook 15. Oxford: Blackwell Scientific Publications, Ltd.