ABSTRACT
Purpose: Roses are one of the ornamental species of major importance and economic value. Fertilisation programmes which maximise plant growth and quality, while minimising environmental impact are important. Here, we followed the N and K dynamics during rose development with the aim to define the nutrient demands as a basis for implementing fertigation programmes.
Materials and methods: Roses with one basal break were grown in a closed hydroponic system. Destructive samples were taken to determine dry weight, N and K content. In each sample, plants were sectioned into roots, rootstock, basal break, stems and leaves from the zone of active leaves and the zone of cutting flowers, as well as the flower bud in the latter.
Results: The shoots of the active leaves and cutting flowers zones exhibited a biphasic growth; dry weight, N and K increased after pruning of the zone of active leaves and the cutting flowers zone, however, in the second phase, total dry weight did not show a significant difference between the phenological phases, while the accumulation of N and K decreased throughout the entire plant.
Conclusions: Our results suggest that greenhouse roses develop a dynamic and complex balance between the aerial parts of the plants and the roots for storage and/or transport of photoassimilates, N and K. The total demand for N was 411 and 799 mg per plant for the initial shoot development of the active leaf zone and cutting flowers zone, respectively. The demand for K was 149 and 106 mg per plant for both shoots, respectively. The loss of N (555 mg per plant) and K (167 mg per plant) from roses plants to the root environment implies that lower fertiliser inputs can be used as these nutrients may be re-uptaken by new emerging roots.
Introduction
Roses (Rosa sp.) are an important ornamental species due to its high value and production, and its high demand worldwide. In roses, fertilisers applications significantly exceed the demands by plants (Franco-Hermida et al. Citation2017). Of particular concern in roses is nitrogen (N) and potassium (K) nutrition, as the balance between both nutrients is reported to affect yield, quality, cold hardiness, and postharvest physiology of ornamental plants (Joiner et al. Citation1983).
Production of high yields and good quality of cut flowers demands high nutrient applications in roses (Franco-Hermida et al. Citation2017), however, N, along with phosphorus and magnesium, is among the macronutrients that growers tend to excessively apply through fertigation, impacting nutrient balance and productivity (Franco-Hermida et al. Citation2013). According to Breś (Citation2009), nitrate and K are the nutrients that are leached in higher amounts in rockwool cultivated roses, up to 83 and 106 kg ha−1 per month, respectively (Breś, Citation2009). The excessive application of fertilisers and leaching of N and K pollutes the soil and groundwater (Thorburn et al. Citation2003; Ju et al. Citation2004; Yu-kui et al. Citation2009), negatively impacting economic revenue; thus, an increase in the efficiency in fertilisation practices is required.
Reduced fertilisation has been demonstrated to negatively affect growth and production/marketability of roses. Chow et al. (Citation2009) reported that reducing nutrient concentration of the fertigation solution to 50% and 33% of the optimum level (10.7 and 3.2 meq L−1 of N and K, respectively) significantly reduced the number of flowering shoots, while increasing the blind shoots, in Rosa hybrida cv Tropicana. Fertigation of roses is usually performed with solutions containing 10.7 to 14.3 meq L−1 of N (Dole and Wilkins Citation2005), while roses in recirculating hydroponic systems have been fed with solutions containing 13.7 and 6.8 meq L−1 of N and K, respectively (Ehret et al. Citation2005). Cabrera (Citation2000) assessed a wide range of N concentrations in the fertigation solution (2.1 to 15.7 meq L−1), concluding that optimum flower and biomass yields were obtained with 6.4 meq L−1. Other reports indicate that roses in recirculating hydroponic systems can be grown with N and K as low as at 3.0 and 1.75 meq L−1 (Cabrera et al. Citation1995a) or 4.3 and 1.7 meq L−1 (Cabrera et al. Citation1995a), respectively.
The objectives of this study were to investigate the biomass, N and K accumulation in roses as well as their dynamics within the plant parts of roses cultivated in a recirculating hydroponic system in order to obtain information useful to delineate fertigation programmes.
Materials and methods
The present study was conducted under greenhouse at the Universidad Autónoma Agraria Antonio Narro, in Buenavista, Saltillo, Coah., México (25° 23′ 42″ N Lat., 100° 59′ 57″ W Long., 1743 m above sea level). The average minimum and maximum temperature recorded during the study was 9.3 and 24.3°C, respectively. The relative humidity ranged between 14.5% and 90.4%. The incident photosynthetically active radiation during the period of highest insolation (12:00 to 14:00 h) was on average 558 μmol m−2 s−1.
Roses plants (Rosa sp.) Cv Freedom, grafted on Natal Briar, were transplanted on July 2 in rigid plastic containers of 30 × 48 × 20 cm (28.8 L). The containers were filled with perlite (33% water retention capacity [v / v], 64% pore space, apparent density of 0.25 g cm−3). A total of 90 containers were placed in an elevated structure to establish a closed hydroponic system with recirculation of the nutrient solution. Four plants were planted in each container.
Plants were irrigated with a modified Steiner nutrient solution prepared with potable water, considering its chemical properties for the supply of nutrients. The pH of the nutrient solution was adjusted daily to 5.9 and ±0.1 with H2SO4 and the electrical conductivity was maintained at 2.0 dS m−1. The nutrient solution was applied through an automated drip irrigation system dispensing 4 L h−1 with four drip stakes installed per container. Plants were fertigated approximately 15 min every 30 min between 7:00 am and 7:00 pm.
The structure of a roses bush is formed by one or several basal breaks, the zone of active leaves and the cutting flowers zone. The basal brakes are those stems developed from the grafting point; in the present study, we allowed the development of only one basal break per plant. The active leaves zone is formed by the stems that develop from the basal break, while the cutting flowers zone corresponds to the stems developed from the active leaves zone. The basal breaks and the stems from the active leaves zones were not allowed to develop flower buds as the buds were removed when they reached 5 mm in diameter (debudding); this practice resulted in the sprouting of axillary shoots from the unifoliate and trifoliate leaves, which were also removed when shorter than 5 cm (deshooting). When the shoot form the last trifoliate leaf was ready to be removed, the bearing stem was pruned, leaving at least two pentafoliate leaves (pinching). The shoots that grow from the pentafoliate leaves formed the cutting-flowers zone and were allowed to develop normally until harvest time.
Roses were established during 112 days and pruned to remove the aerial portion, leaving the basal breaks at ∼60 cm. During the study period, seven destructive samplings were conducted, which corresponded to the phenological phases indicated in . At each of the sampling dates, five plants from the 90 containers were randomly selected and carefully removed from their containers. Plants were then rinsed free of excess perlite and carefully divided into root, rootstock, basal break, stems and leaves of the active leaves zone, and stems, leaves and flower buds of the cutting flowers zone. The separated plant parts were placed in a drying oven at 70°C for 72 h, except for the stems, which were maintained between 15 and 20 days. Once the plants parts were dried, the dry weight (DW) was recorded using a digital scale.
Table 1. Destructive samplings performed on rose plants and stage of growth.
Plant N and K was determined by tissue digestion with 2 mL of a mixture of H2SO4 and HClO4, and 1 mL of H2O2. The digest was taken to 25 mL and filtered. In an aliquot of the resulting sample, the concentration of N was determined by the semi-micro Kjeldahl method, while the concentration of K was determined by inductively coupled plasma (ICP-AES 725 Series Agilent, Mulgrave, Victoria, Australia). Total N and K contents were determined in relation to the DW and the concentration of these nutrients in the different organs (leaf, stem, etc.) of the plant, with the sum of the content of the nutrients representing the total accumulation per plant. The average of each sampling was plotted with SigmaPlot 12.5 (Stat Ease, Minneapolis, MN) and a segmented analysis using linear, quadratic or cubic models was used to determine the tendencies.
Results
Growth and N and K dynamics of the zone of active leaves
From the sprouting of new shoots of the active leaves zone to the deshooting of the active leaves zone (once the basal break was pruned). An increase of total plant DW ((a)) associated with a partial gain in the DW of roots ((a)), rootstock ((a)), and that of the developing active leaves zone ((a)) was observed, however, it was the basal break that presented the highest DW increase ((a)). The accumulation of total DW was associated with an increase in total N ((b)) and K ((c)), which was observed in all the plant parts but occurred mainly in the basal break ((b–c)). The zone of active leaves accumulated N ((b)), especially in the leaves, and K, especially in the stem ((c)).
Figure 1. Total dry weight (a), N (b) and K (c) accumulated in roses during the development of the zone of active leaves and zone of cutting flowers, including the harvest. DD = debudding of the active leaves zone, DS = deshooting of the active leaves zone, P = pinch of the active leaves zone, S10 cm = shoots of the cutting flowers zone with 10 cm length, S15 cm = shoots of the cutting flowers zone with 15 cm length, FBV = flower buds visible in the cutting flowers zone, FSE = flower stem elongation in the cutting flowers zone, H = harvest of flower. Bars represent the standard error of the mean (n = 5).
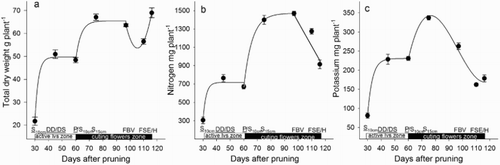
Figure 2. Dry weight (a), N (b) and K (c) accumulated in the roots of roses during the development of the zone of active leaves and zone of cutting flowers, including the harvest. DD = debudding of the active leaves zone, DS = deshooting of the active leaves zone, P = pinch of the active leaves zone, S10 cm = shoots of the cutting flowers zone with 10 cm length, S15 cm = shoots of the cutting flowers zone with 15 cm length, FBV = flower buds visible in the cutting flowers zone, FSE = flower stem elongation in the cutting flowers zone, H = harvest of flower. Bars represent the standard error of the mean (n = 5).
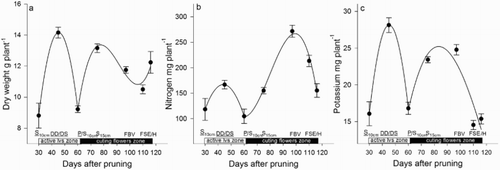
Figure 3. Dry weight (a), N (b) and K (c) accumulated in the rootstock of roses during the development of the zone of active leaves and zone of cutting flowers, including the harvest. DD = debudding of the active leaves zone, DS = deshooting of the active leaves zone, P = pinch of the active leaves zone, S10 cm = shoots of the cutting flowers zone with 10 cm length, S15 cm = shoots of the cutting flowers zone with 15 cm length, FBV = flower buds visible in the cutting flowers zone, FSE = flower stem elongation in the cutting flowers zone, H = harvest of flower. Bars represent the standard error of the mean (n = 5).
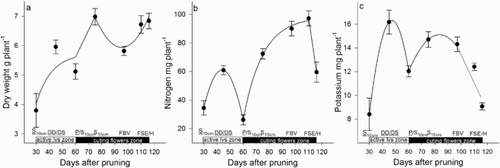
Figure 4. Dry weight (a), N (b) and K (c) accumulated in the stem and leaves of the active leaves zone (ALZ) of roses during the development of the zone of active leaves and zone of cutting flowers, including the harvest. DD = debudding of the active leaves zone, DS = deshooting of the active leaves zone, P = pinch of the active leaves zone, S10 cm = shoots of the cutting flowers zone with 10 cm length, S15 cm = shoots of the cutting flowers zone with 15 cm length, FBV = flower buds visible in the cutting flowers zone, FSE = flower stem elongation in the cutting flowers zone, H = harvest of flower. Bars represent the standard error of the mean (n = 5).
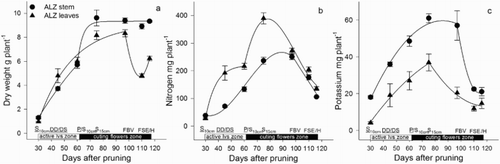
Figure 5. Dry weight (a), N (b) and K (c) accumulated in the basal break of roses during the development of the zone of active leaves and zone of cutting flowers, including the harvest. DD = debudding of the active leaves zone, DS = deshooting of the active leaves zone, P = pinch of the active leaves zone, S10 cm = shoots of the cutting flowers zone with 10 cm length, S15 cm = shoots of the cutting flowers zone with 15 cm length, FBV = flower buds visible in the cutting flowers zone, FSE = flower stem elongation in the cutting flowers zone, H = harvest of flower. Bars represent the standard error of the mean (n = 5).
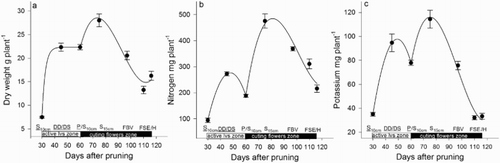
From the deshooting to the pinching of the active leaves zone. On a whole plant basis, DW did not show a significant difference between the deshooting and pinching in the active leaves zone ((a)). However, the deshooting was associated with a loss of 35% and 14% in the DW of roots ((a)) and rootstock ((a)), respectively. In spite of this, the DW accumulation in the stems and leaves of the zone of active leaves maintained the total DW of the plant ((a)). The unaffected total DW was related to unaffected total N ((b)) and K ((c)), however, there was a strong decrease in the content of both nutrients in all the plants parts (), except for the zone of active leaves as they exhibited an increase in N ((b)) and K ((c)).
Growth and N and K dynamics of the zone of cutting flowers
From the pinching of the zone of active leaves to the sprouting of cutting-flowers zone. Total plant DW increased by 39% ((a)), which was associated with an increase in the DW in all plant parts ((a)). The increase in total DW resulted in a 109% increase of total N ((b)) and 46% of total K ((c)) in relation to the previous sampling, which occurred especially in the rootstock ((b–c)), in the basal break ((b–c)), and in the leaves of the active leaves zone ((b–c)).
From the sprouting of the cutting flower zone to the flower bud with size shorter than 5 mm. In contrast to the total plant DW ((a)), both the leaves and the stem of the cutting flowers zone ((a)) and the active leaves zone ((a)) increased their DW, which was associated to a decrease in the DW of the root ((a)), the rootstock ((a)), as well as in the basal break ((a)). Total N accumulated decreased after the flower bud became visible ((b)) while the reduction of K was detected when the shoot of the cutting flowers zone was longer than 15 cm ((c)). In contrast, the content of N ((a)) and K ((b)) increased in stem and leaves of the cutting flowers zone, so that the decrease at a whole plant level was due to a reduction of these nutrients in the root after the flower bud became visible ((b–c)), and in the basal break ((b–c)) and in leaves of the active leaves zone ((b–c)) after the sprouting shoots of the cutting flowers zone ((b–c)). In the rootstock there was a contrasting result as the concentration of both nutrients increased ((b-c)).
Figure 6. Dry weight (a), N (b) and K (c) accumulated in the stem, leaves and flower bud during the development of the zone of cutting flowers (CFZ) of roses, including the harvest. DD = debudding of the active leaves zone, DS = deshooting of the active leaves zone, P = pinch of the active leaves zone, S10 cm = shoots of the cutting flowers zone with 10 cm length, S15 cm = shoots of the cutting flowers zone with 15 cm length, FBV = flower buds visible in the cutting flowers zone, FSE = flower stem elongation in the cutting flowers zone, H = harvest of flower. Bars represent the standard error of the mean (n = 5).
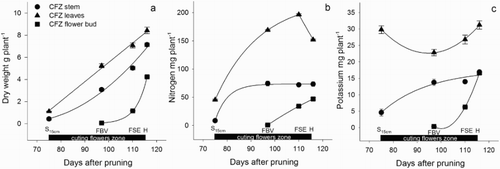
From the flower bud shorter than 5 mm until flower harvest. There was an increase in the leaf DW and the stem of the cutting flower zone ((a)), so that there was an increase in the total DW accumulated when the flower reached the harvesting point ((a)), which was associated with an increase in mass in all plant parts. During the last part of the development of the flowering stem, N and K decreased markedly ((b–c)), which was due to a reduction in the content of these nutrients in all parts of the plant, except in the organs of the cutting flowers zone ((b–c)).
Discussion
Roses started the accumulation of N and K concurrently with the initial development of the shoots of the active leaves and cutting flowers zones (); however, after the debudding, deshooting and pinching of this shoot in the active leaves zone, the accumulation halted. The uptake and accumulation of N and K was resumed when the shoot that developed after pinching started to grow, although it was halted again once the shoot of the cutting flowers zone reached 15 cm in length and the flower bud became visible (). This cyclic pattern of nutrient uptake and biomass accumulation has been previously reported for roses by Cabrera et al. (Citation1995a) and Mattson and Lieth (Citation2005).
The cyclic pattern has been associated with a reduction in root growth during the development of new leaves and shoots, as demonstrated in white oak (Quercus alba L.) (Reich et al. Citation1980). In the present study, we did observe a decline in root growth (loss of biomass as a result of root turnover) ((a)). According to Silberbush and Lieth (Citation2004), in mature rose plants there is a continuous formation of new roots, while the older roots decay. In our study, the loss of root DW occurred 40 days after the development of the cutting flowers zone started, and coincided with: (1) the arrest in the accumulation of N and K ((b–c)), and (2) the debudding of the shoot in the active leaves zone and the active growth of the shoot from the cutting flowers zone ((a)). Other reports indicate a reduction in root growth or nutrient uptake during the maximum elongation rate of the flowering stems (Cabrera et al. Citation1995a; Kim and Lieth Citation2012). In the present study we are also reporting a reduction in growth or nutrient uptake, however, in addition to this reduced growth and uptake rate there was also a reduction in the total DW ((a)) and total content of N and K ((b–c)), which were associated with the development of the flower buds and the rapid elongation of the flower stem. These losses occurred after the flower bud became visible, and coincided with a rapid elongation of the flowering stem.
In our study the roses plants reached harvest stage 60 days after the pruning of the shoot from the active leaves zone ((a)). During the first 40 days, DW, N and K accumulation closely resembled growth and nutrient accumulation reported for roses by Kim and Lieth (Citation2012), however, the authors did not report any biomass or nutrient loss. We suggest that these differences between our current study and that of Kim and Lieth (Citation2012) reflect a decrease in N and K tissue concentration at the whole plant level (data not shown).
We proposed that the reduction in DW and N and K content at a whole plant level may be a result of: (1) the loss of DW of roots (), the rootstock () and the basal break (), (2) the limitation of carbohydrates supply to the roots to provide reserves for the developing flowering shoot (Cabrera et al. Citation1995a), which in turn causes root death, (3) a potential energy depletion due to translocation of reserves from the aerial plant parts to the roots to enhance new roots formation, as suggested by the increased demand of sucrose in roses grown in vitro (Hyndman et al. Citation1981), (4) signalling from the aerial plant parts to the roots to enhance the formation of new roots once they die, as suggested by the increased demand of N in roses grown in vitro (Hyndman et al. Citation1981), (5) the transport of nitrogenous substances, such as polyamines, from the aerial part to the roots, which may be later lost to the growing medium; these substances are reported to stimulate the formation of new roots in rose plants (Yeo et al. Citation2010), and (6) the rootstock used in the present study, which reportedly develops more roots than other commonly used rootstocks for roses (Cabrera et al. Citation1995b), demanding high levels of carbohydrate reserves and N and K for signalling.
The accumulation of biomass in the root occurred in tandem with the initial shoot growth following pinching ((a)), while root DW declined as the shoot started to rapidly expand. In spite of the decrease in biomass and nutrient content at a whole plant level (), in the developing flowering shoot (cutting flowers zone), the stem, leaves and flower bud exhibited an increase in DW, N and K content (). We suggest that N and K were redistributed from the root ((b–c)), the rootstock ((b–c)) and the basal break ((b–c)) to meet and sustain the needs of the developing flowering shoot (Cabrera et al. Citation1995b).
Mor and Halevy (Citation1979) reported that the development of shoots in roses represents a strong demand for photoassimilates until the flower buds become visible. Just after the appearance of flower buds, most of the leaves in this new shoot become a source of assimilates. These results support our premise that the N and K absorption rates in roses are at least in part governed by the availability of assimilates in the roots. The absorption rate of nutrients such as K is parallel to the changes in N absorption, which is consistent with the absorption rates reported for Euonymus japonica (Hershey and Paul Citation1983).
According to our observations, there is a period in which roses plants need to be fed with N and K, as these nutrients are actively uptaken. This occurs, for both the active leaves ((b–c)) and the cutting flowers zones ((b–c)), from the pinching up to when the shoots that develop after the pinch reach 10 to 15 cm in length (slow growth and biomass accumulation). During the remaining development, roses plants do not take up more N or K, and the needs to complete shoot growth were met by nutrient mobilisation from other plant parts ().
In our study, roses plants with one single flowering stem and one single basal break, exhibited a high N demand after pinching. The total demand for N was 411 mg per plant for the initial shoot development of the active leaf zone ((b)) and 799 mg per plant for the initial shoot development of the cutting flowers zone ((b)). The lower N demand for the development of the shoot from the active leaves zone may be because it was not allowed to complete its cycle as it was pruned when the flower buds became visible. The demand for K was 149 and 106 mg per plant for both shoots, respectively ( and ). According to Cabrera et al. (Citation1995a), for the developing of the flowering stem, roses plants demand 30 mg N per plant day−1 during the autumn-winter season; as the uptake on N in our study took approximately 30 days for the shoot of the cutting flowers zone, it would imply a total demand of N of 900 mg per plant, closely resembling that we are reporting (799 mg per plant).
These figures for N and K demands may be useful to delineate fertilisation programmes as long as the flowering flushes in roses plants are manipulated by pruning practices (Cabrera et al. Citation1995a), since non-coordinated flushes within a roses bush would imply flowering stems with different phenological phases and thus, different nutrient demands. However, these figures do not allow for the N and K lost that we are reporting. In that, these nutrients may enter the N and K cycles in the soil and may become available for re-uptake by the new emerging roots, thus reducing the amount of fertilisers input needed. Our data indicate that as much as 555 and 167 mg plant−1 of N and K, respectively, are lost to the root environment after the flower bud in the flowering stem becomes visible (for N), or after the shoot of the flowering stem reaches 15 cm in height (for K), which makes them potentially available for reuse by plants.
In the present study, we observed a detention in growth and N or K accumulation and a loss of total biomass and N and K. In spite of this, the developing shoot that produced the flower exhibited an increase in biomass and accumulation of these nutrients, which was due to the redistribution of N and K from the roots, rootstock and basal break. Our observations allowed estimating that for the growth of a single flowering stem, rose plants demand 799 and 106 mg per plant of N and K, respectively; however, the loss of N and K from roses plants to the root environment implies that lower fertiliser inputs may be needed as these nutrient may be re-uptaken by new emerging roots.
Disclosure statement
No potential conflict of interest was reported by the authors.
Notes on contributors
Dr Daniela Alvarado-Camarillo has a postdoctoral position at Facultad de Ciencias Agrícolas, Universidad Autónoma del Estado de México, Toluca, Estado de México, México. She received her Doctor in Science degree in protected agriculture at Universidad Autónoma Agraria Antonio Narro in 2017. Currently, she is working on hydroponic cultivation of ornamental plants, floriculture and plant nutrition.
Dr Luis A. Valdez-Aguilar is a senior full time researcher and professor at Universidad Autónoma Agraria Antonio Narro. His research is focused mainly on the hydroponic cultivation of greenhouse ornamental and vegetable species, nutrient interactions, design of fertilization programs for ornamentals and hydroponic systems. He received his PhD degree in horticulture at Texas A&M University in 2004 and has published 78 papers.
Dr Ana María Castillo-González is a senior full time researcher and professor at Universidad Autónoma Chapingo. Her research is focused on determining the optimum nutrition of horticultural plants such as ornamentals, fruit trees and berries. She received her Doctor in Science degree in fruticulture at Colegio de Postgraduados, México, in 1997 and has published 36 papers, one book, and four book chapters.
Dr Libia I. Trejo-Téllez is a senior full time researcher in the Departamento of Edafologia at Colegio de Posgraduados, México. In 2004 she got the degree of Dr. rer. nat. by the Free University of Berlin (Germany) and does research in soil science, horticulture and agricultural plant science. She has over 15 books and book chapters and 150 papers published.
Dr Silvia Y. Martínez-Amador is a senior full time researcher and professor at Departamento de Botánica at Universidad Autónoma Agraria Antonio Narro. Her research interest is related to the use of degraded waters and treatments for irrigation of greenhouse crops. She received his PhD degree in biotechnology at Universidad Autónoma de Coahuila in 2007 and has published 35 papers.
ORCID
Luis A. Valdez-Aguilar http://orcid.org/0000-0002-2510-1962
References
- Breś W. 2009. Estimation of nutrient losses from open fertigation systems to soil during horticultural plant cultivation. Pol J Environ Stud. 18:341–345.
- Cabrera RI. 2000. Evaluating yield and quality of roses with respect to nitrogen fertilization and leaf nitrogen status. Acta Hortic. 511:133–142. doi: 10.17660/ActaHortic.2000.511.15
- Cabrera RI, Evans RY, Paul JL. 1995a. Cyclic nitrogen uptake by greenhouse roses. Sci Hortic. 63:57–66. doi: 10.1016/0304-4238(95)00789-V
- Cabrera RI, Evans RY, Paul JL. 1995b. Nitrogen partitioning in rose plants over a flowering cycle. Sci Hortic. 63:67–76. doi: 10.1016/0304-4238(95)00790-Z
- Chow A, Chau A, Heinz KM. 2009. Reducing fertilization for cut roses: effect on crop productivity and twospotted spider mite abundance, distribution, and management. J Econ Entomol. 102:1896–1907. doi: 10.1603/029.102.0521
- Dole JM, Wilkins HF. 2005. Floriculture. Principles and species. Upper Saddle River (NJ): Pearson Prentice Hall.
- Ehret DL, Menzies JG, Helmer T. 2005. Production and quality of greenhouse roses in recirculating nutrient systems. Sci Hortic. 106:103–113. doi: 10.1016/j.scienta.2005.03.002
- Franco-Hermida JJ, Henao-Toro MC, Guzmán M, Cabrera RI. 2013. Determining nutrient diagnostic norms for greenhouse roses. HortScience. 48:1403–1410.
- Franco-Hermida JJ, Quintero MF, Cabrera RI, Guzman JM. 2017. Determination of diagnostic standards on saturated soil extracts for cut roses grown in greenhouses. PLoS ONE. 12:e0178500. https://doi.org/10.1371/journal.pone.0178500.
- Hershey DR, Paul JL. 1983. Ion absorption by a woody plant with episodic growth. HortScience. 18:357–359.
- Hyndman SE, Hasegawa PM, Bressan RA. 1981. The role of sucrose and nitrogen in adventitious root formation on cultured rose shoots. Plant Cell, Tissue Organ Cult. 1:229–238. doi: 10.1007/BF02318919
- Joiner JN, Poole RT, Conover CA. 1983. Nutrition and fertilization of ornamental greenhouse crops. In: Janick J, editor. Horticultural reviews. Volume 5. Westport (CT): The AVI Publishing Company Inc; p. 317–403.
- Ju X, Liu X, Zhang F, Roelcke M. 2004. Nitrogen fertilization, soil nitrate accumulation, and policy recommendations in several agricultural regions of China. AMBIO: a Journal of the Human Environment. 33:300–305. doi: 10.1579/0044-7447-33.6.300
- Kim WS, Lieth JH. 2012. Simulation of year-round plant growth and nutrient uptake in Rosa hybrida over flowering cycles. Horticulture, Environment, and Biotechnology. 53:193–203. doi: 10.1007/s13580-012-0054-y
- Mattson NS, Lieth JH. 2005. Modeling macronutrient absorption of hydroponically-grown cut flower roses. Acta Hortic. 751:129–135.
- Mor Y, Halevy AH. 1979. Translocation of 14C-assimilates in roses. I. The effect of the age of the shoot and the location of the source leaf. Physiol Plant. 45:177–182. doi: 10.1111/j.1399-3054.1979.tb01683.x
- Reich PB, Teskey RO, Johnson PS, Hinckley TM. 1980. Periodic root and shoot growth in oak. For Sci. 26:590–598.
- Silberbush M, Lieth JH. 2004. Nitrate and potassium uptake by greenhouse roses (Rosa hybrida) along successive flower-cut cycles: a model and its calibration. Sci Hortic. 101:127–141. doi: 10.1016/j.scienta.2003.10.009
- Thorburn PJ, Biggs JS, Weier KL, Keating BA. 2003. Nitrate in groundwaters of intensive agricultural areas in coastal Northeastern Australia. Agric Ecosyst Environ. 94:49–58. doi: 10.1016/S0167-8809(02)00018-X
- Yeo KH, Cho YY, Lee YB. 2010. Estimation of shoot development for a single-stemmed rose ‘vital’ based on thermal units in a plant factory system. Korean J Horticult Sci Technol. 28:768–776.
- Yu-kui R, Shi-ling J, Fu-suo Z, Jian-bo S. 2009. Effects of nitrogen fertilizer input on the composition of mineral elements in corn grain. Agrociencia. 43:21–27.