ABSTRACT
Soil properties and agricultural practices take a joint effect on the communities of soil bacteria. The aim of the present study was to survey Alphaproteobacterial communities as possible indicators of soil quality considering clay, loamy and sandy soils under conventional and organic farming. Alphaproteobacteria community composition were analysed by 16S rRNA gene with nested-PCR (polymerase chain reaction) and denaturing gradient gelelectrophoresis (DGGE). Sequencing of partial 16S rRNA gene from the DGGE bands were performed. Conventional and organic farming resulted in significant differences in chemical properties of soils. According to the results community fingerprints were separated into groups depending on soil types and farming systems. This separation can be attributed mostly to soil pH, AL-P2O5,-K2O. The analysed sequences were identified as soil bacteria which could play the main role in nitrogen fixing, mineralisation and denitrification. The highest diversity index was revealed from the organic farming at sandy texture site, where mainly Mesorhizobium sp. and Rhizobium sp. were detected. The soil type and actual crop could have a stronger impact on the soil bacterial composition than the management.
Introduction
The composition of the soil microbial communities is influenced by the land cultivation methods and other biotic and abiotic factors (Marinari et al. Citation2006). According to Diepeningen et al. (Citation2006) the different agricultural land management methods and soil types have effects on the abundance, activity and diversity of soil microbial communities. The activity of healthy soil microbial communities can contribute to sustainable agro-ecosystems.
The impact of the land management method on the soil bacteria has been studied intensively but there are still many unanswered questions in this topic (Edesi et al. Citation2012; Ding et al. Citation2013).
Soil microbes especially those promoting plant nutrition especially important in infertile and low-nutrient supplied soils (van der Heijden et al. Citation2008; Wang and Cheng Citation2017). Phosphorus and nitrogen are the two most limiting elements for plant nutrition, therefore N2-fixing and P-mobilising bacterial taxa are essential in plant growth. Bissett et al. (Citation2011) detected growing rates of nitrification and higher levels of nitrogen with high microbial biomass in soils under organic land management compared to conventional farming systems.
The bacterial activity can be influenced by soil physical clay, loam and sand content), chemical (pH value, nitrate and ammonium content, phosphate and organic carbon levels) and biological parameters (Diepeningen et al. Citation2006; Williamson et al. Citation2011). Positive correlation between soil pH and the abundance of soil bacteria was also observed (Lauber et al. Citation2009).
In this study soil microbial communities at three sites Karcag (K), Martonvásár (M) and Nyíregyháza (Ny) in Hungary having distinct soil texture, (clay, loam and sand, respectively) with conventional (C) and organic (O) land managements were compared.
The objectives of this paper were to determine the impact of different soil types (clay, loamy, sandy), the main soil physical–chemical characteristics, the cropping systems on the soil microbial community composition focusing on the Alphaproteobacteria.
Alphaproteobacteria show a fine capability to conform to diverse environmental conditions and the association between bacteria and plants runs from non-symbiotic to symbiotic relationships. Alphaproteobacteria is a significant group in relation to research of the environmental and anthropogenic impacts on functional and genetic diversity of soil microorganisms and also in the rhizosphere (Orr et al. Citation2012).
DNA based molecular microbiological methods can provide great insight to the diversity and variability of soil microorganisms at different organisation levels from species to communities (McCaig et al. Citation1999; Lynch et al. Citation2004). Nested-PCR (polimerase chain reaction) and denaturing gradient gel electrophoresis (DGGE) methods were used to amplify the 16S ribosomal RNA gene of Alphaproteobacteria and study their community structural diversity (Plassart et al. Citation2012; Ding et al. Citation2013). The method discloses the molecular fingerprints of soil bacterial communities and by sequencing bands it could also provide taxonomic-phylogenetic information about the community members (Székely et al. Citation2009; Bárány et al. Citation2014). We typified the soil Alphaproteobacteria community structural diversity and dominant species from the three soil types with different land management systems.
Materials and methods
Soil samples
Three soil types representing three different textures were examined having different soil physical characteristics. The clay soils were collected from the University of Debrecen Research Institute of Karcag from conventional (5.67 ha) and from organic (5.67 ha) land management systems. The loamy soils were collected in Martonvásár from a 15 years long-term field experiment in the Centre for Agricultural Research. The conventional and organic soil samples originated from the sites of 0.5 ha. Sandy soil samples were collected from University of Debrecen Institutes for Agricultural Research and Educational Farm, Research Institute of Nyíregyháza, from the Westsik crop rotation experiment (conventional) (6.7 ha) and from the organic sites (14.19 ha) ().
Table 1. Crop rotation land management and manuring of arable farming types in conventional (C) and organic (O) systems at the three sites of Karcag (K), Martonvásár (M) and Nyíregyháza (Ny) four years before sampling.
Karcag soil type is a Luvic Phaeosem, Aquic Hapludoll with clay texture (USDA) (FAO Citation1998). Martonvásár is a calcic chernozem soil (FAO Citation1998), soil texture is a clay loam (USDA). Nyíregyháza soil type is Mollic Fluvisol (USDA) (FAO Citation1998) with sand texture.
Soil samples were collected from three sites of Hungary under (C) and (O) land management methods in May 2012 along the diagonal of a field from the rizosphere soil 0–20 cm below the soil surface using a borer. 12–12 samples were taken from each site both from (C) and (O) farming systems. Soil samples were homogenised, air-dried, ground, and sieved through a 2 mm mesh for physico-chemical analysis. Another part of soil samples were kept at −20°C until laboratory processing.
Physical and chemical properties of soils
The following soil physico-chemical analyses were performed based on the Hungarian soil standards: the texture of soils was determined by the USA method – clay and loamy soil by pipetting with Sartorius type analytic scale - sandy soil by sifting - according to the following categories: sand (0.05–2 mm), silt (0.002–0.05 mm), clay (<0.002 mm). The total salt content was measured based on the electric conductivity (EC 2.5) of soil: water (1:2.5) suspension (mS cm−1). The pHH2O and pHCaCl2 (0.01N) values were recorded by using an ABU 93 TRIBURETTE radiometer Copenhagen and TIM 900 titration manager TitraLab Radiometer analytical. The (mg kg−1),
(mg kg−1) analysis and the total N (%), C (%), the humus content (%) (was calculated from soil organic C (%) × 1.725), the ammonium-lactate (AL)-soluble nutrient content: Ca (m/m%), K2O (mg kg−1), Na (mg kg−1), P2O5 (mg kg−1) were determined as described by Buzás (Citation1988, Citation1993).
Molecular analysis
DNA extractions were performed from 0.25 g of homogenised soil using the Zymo Research Soil Microbe DNA MiniPrep Kit according to the manufacturers’ instructions. Twelve community DNA isolates were obtained from each area. Total soil nucleic acid concentration was quantified using ND 1000 Nanodrop spectrophotometer (Thermo Scientific). The average total DNA yield was calculated from the absorbance (A) at 260 nm. The purity of nucleic acid was estimated by the average ratio between the A260/A280 (Yeates et al. Citation1998; Lakay et al. Citation2006; Técher et al. Citation2010). The extracted total DNA was stored at −20°C until use.
From the community, DNA extracts four soil DNA samples per each site were chosen to investigate by DGGE.
Prior to DGGE partial 16S rRNA gene was amplified by a nested-PCR method in two steps. The first was carried out using R1494: (5′-CTACGGTTACCTTGTTACGAC-3′) and F203α: (5′-CCGCATACGCCCTACGGGGGAAAGATTTAT-3′) Alphaproteobacteria specific primer pair, followed by a second PCR with R1378 (5′-CGGTGTGTACAAGGCCCGGGAACG-3′) and F984GC (5′-AACGCGAAGAACCTTAC-3′) universal bacterial primer pair. A GC-clamp (5′-CGCCCGGGGCGCGCCCCGGGCGGGGCGGGGGCACGGGGGG-3′) was attached to primer F984GC to avoid the complete denaturation of the DNA strands (Tsushima and Matsushita Citation2010; Gomes et al. Citation2011). DNA amplification was performed in a total volume of 50 µl, containing 10 µM of each primer, 25 µl Maxima Hot Start PCR Master Mix (2x) (Thermo Scientific), 16 µl nuclease free water (Thermo Scientific), and contained 5 µl of the DNA extract. PCR amplifications were performed in a thermal cycler (ICYCLER, BIO-RAD). Temperature profile of the first PCR included: initial denaturation (95°C, 4 min) followed by 30 cycles of denaturation (95°C, 30 s), annealing (56°C, 30 s) and extension (72°C, 1 min), with a single final extension (72°C, 10 min). The second PCR was carried out with almost the same temperature profile as the first one, except that the annealing temperature was at 53°C (Singh et al. Citation2011). Each PCR reaction was checked by electrophoresis in a 1.5% agarose gel (SeaKem LE Agarose (Lonza) containing 1xTAE (Tris acetate-EDTA) buffer (AccuGENE 50xTAE Buffer (Lonza)) and nucleic acid staining solution 20.000x (ECO Safe, Pacific Image Electronics). PCR product was visualised under UV light.
DGGE was performed in a 7% polyacrylamide (PAA) gel containing 40–60% gradient of denaturants (urea and formamide) at 60°C for 13.5 h at a constant voltage (120 V) using INGENYphorU-2 electrophoresis system (Ingeny International BV, Netherlands). The gel was stained in EtBr solution for 20 min followed by two washing steps for 15 min each in 1xTAE buffer. Dominant, well separated and intense bands were excised, and DNA was reextracted from the gel in 30 µl nuclease free water during an overnight incubation at 4°C. Reamplification was carried out using the same PCR protocol as used by the second PCR prior to DGGE (Ding et al. Citation2012). Sanger sequencing of the DNA was performed with 3500 Series Genetic Analyzer (Life Technologies) in the Biological Research Centre, Szeged (Hungary).
Statistical analyses
Differences in soil chemical and physical properties between the organic and conventional land management types were tested by the two sample t-tests, n = 12. For the molecular analyses, the soil samples were n = 4 which represent the above area. Similarity percentages test (SIMPER) was used to identify which physical–chemical parametres had the largest contribution to the average dissimilarity among the two different land use of the three sites. Principal component analysis (PCA) was used to visualise the differences between the dissimilar soil managements on soil chemical properties, n = 12, except KC, MC, MO, NYC where we used n = 11. The above statistical tests were performed using Past3 (Hammer et al. Citation2001). The UPGMA dendrogram based on the DGGE fingerprints was made with TotalLab TL120. v 2006. software. Dominant community members were identified by partial 16S rRNA gene sequence analysis. Chromas Lite 2.1.1 (Technelysium, Australia) software was used to evaluate sequences and the nearest cultivated relative of each strain and clone was found by EzTaxon (Yoon et al. Citation2017) and NCBI database (Altschul et al. Citation1997). The soil bacterial diversity was measured with Shannon-Wiener diversity index (H’), Evenness (E) and richness (R) (Hill et al. Citation2000).
Results
Characterisation of physical and chemical soil properties
Based on the PCA () along PC2 axis the samples from clay soil (Karcag) principally dominated by AL-Na. Near PC1 axis the samples from loamy soil (Martonvásár) represented mainly the EC, pH(H2O) and pH(CaCl2). The two different land management methods for clay (Karcag) and sandy (Nyíregyháza) soil sites () separated into groups in contrast to the MC and MO sites were partially divided. According to the SIMPER test analysis, the largest contribution to the dissimilarities was detected at AL-P2O, AL-K2O, ,
, AL-Na, AL-Ca parameters for Martonvásár (Bray–Curtis distance), AL-P2O, AL-Na, AL-K2O,
,
for Karcag and AL-K2O, AL-P2O, AL-Na,
,
for Nyíregyháza ().
Figure 1. Principal components (PCA) biplot analysis among the soil samples (Karcag, Martonvásár and Nyíregyháza) from conventional and organic farming systems. (Legends: C = conventional, O = organic systems, K = Karcag, M = Martonvásár, Ny = Nyíregyháza, n = 12, except KC, MC, MO, NYC n = 11 were used, filled circle = KC, circle = KO, filled triangle = MC, triangle = MO, filled square = NyC, square = NyO).
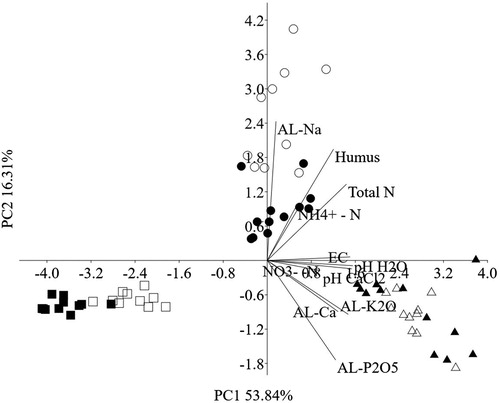
Table 2. The main soil physical properties from conventional and organic farming systems in the three sampling sites.
Table 3. The main soil chemical parameters originated from three different soil types with conventional and organic farming systems.
Analysis of alphaproteobacteria community
Alphaproteobacteria community structures of the three soil types formed separate groups according to the DGGE patterns (). The first distinct group (at 40% similarity) comprised the community structures of loamy soil samples. The second branching (at 47% similarity) separated the community fingerprints of the clay and the sandy soils. The smallest spatial heterogenity was typical for loamy soil and the largest was revealed in the sandy soil samples (NyO). Separation of the community patterns of conventional and organic soil samples was observed at 60–65% similarity in almost every case. According to the number of DGGE bands, samples from conventional land management sites had higher soil bacterial diversity than those from organic management. The most diverse soil type was the sandy (NyC) farming samples (H’ = 2.82; E = 0.89; R = 23), (for organic (H’ = 2.78; E = 0.86; R = 25)). The lowest diversity was observed in the clay soil of (KO) farming system (H’ = 2.46; E = 0.87; R = 17), however, Alphaproteobacteria diversity of conventional farming of this site was higher (H’ = 2.65; E = 0.87; R = 20). The loamy soil showed medium diversity indices for MC (H’ = 2.68; E = 0.89; R = 19) and (H’ = 2.59; E = 0.87; R = 19) for MO farming systems. The bacterial diversity indices were not significantly different from each other except for the species richness of the bacterial communities of clay soil (KC) which was significantly higher than KO farming land. The excision and sequence analysis of 14 separate intensely stained bands were carried out. The obtained 16S rRNA sequences showed high similarity to known soil bacteria or uncultured environmental sequences (≥ 96% identity) (). Two isolates were identified to species rank using the EzTaxon-e database. Three isolated samples were showed genus similarity with soil bacteria. Nine isolates showed the highest sequences similarity to uncultured bacteria originated from soil samples these were revealed in (C) sandy and (O) clay and sandy soils. Alphaproteobacteria class and the Bacteria domain was revealed in the investigated samples. Despite the differing soil types, there were overlapping taxon composition observed between the studied sites and farming systems.
Figure 2. The banding pattern of the DGGE UPGMA dendrogram of bacterial communities. (Legends: C = conventional, O = organic systems, K = Karcag, M = Martonvásár, Ny = Nyíregyháza, the codes of farming systems with numbering 1–4 indicated the soil samples (n = 4) which best represent the above area. Numbers in the banding patterns 1–14 indicated the isolated and sequenced bands (thick lines)).
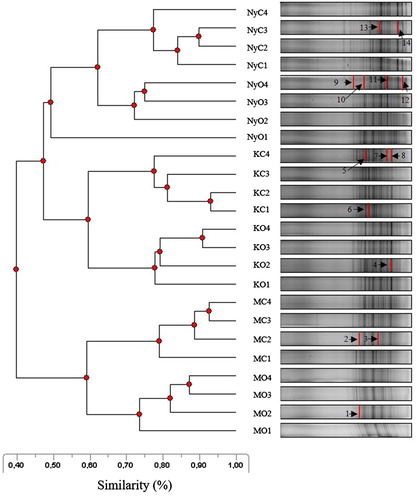
Table 4. The closest phylogenetic relatives of 16S rRNA sequences isolated from DGGE banding patterns.
Discussion
Most of the investigated soil chemical parameters showed significant differences between the different soil types with conventional and organic farming systems (). Physical () -chemical soil characteristics (pH, EC, humus, nutrient availability, etc.) can affect soil bacterial community composition (Jeffery et al. Citation2010; Li et al. Citation2012). Most measured pH values from the soil types of (O) sites were significantly higher compared to those measured pH from conventional systems (), however, soil alkalinity of both farming systems were within the optimum range for bacteria (Jeffery et al. Citation2010). Humus contents, total nitrogen contents and concentrations were significantly higher in clay and loamy soils with (O) sites except the sandy soil (Ny) (n.s.). However, the
content was significantly higher in loamy and sandy soils between the two farming systems. Alphalpha crops grown on the clay and sandy soil may explain this differences. Clay soil (KO) had significantly higher humus and total nitrogen content than the KC owing probably to the green manure provided by sweet clover (). The result of the PCA () indicates three well-separated groups of variables according to the different soil types. As can be seen, relationships were occurred among the (C) and (O) sites. Based on the results of PCA soil
content was also a remarkable parameter there, which could indicate the shift in the rate of N mineralisation or nitrification between the (C) and (O) managed soils. The humus content from the loamy soil (MO) was significantly higher compared to the (MC) sites which could be due to the green manure of pea amendment and the preservation of organics in the high clay and loam content of this soil. In all cases, the soil nitrogen forms were higher at conventional farming sites than at (O) systems, due probably to the effect of the applied fertiliser. However fertiliser was not used at sandy soil sites (NyC) which resulted lower nitrate levels (Lauber et al. Citation2009) compared to other sites. Our results indicated that the concentrations of the AL-soluble nutrients showed significant differences between the different soil types with (C) and (O) sites except for AL-Ca. Based on the results of SIMPER we found that the following environmental factors predict best the difference between clay (KC, KO) and sandy (NyC, NyO) sites: AL-P2O5, AL-K2O and AL-Na. While in loamy soil (MC and MO), AL-Na and the
were the most differentiating.
During molecular analysis, we obtained appropriate yield and purity of soil DNA (Niemi et al. Citation2001). From the PAA gel, only the intensely stained and separated bands were isolated from the different soil types and land managements methods, which provides an information about the detected Gram-negative soil bacteria (). Lopes et al. (Citation2011) investigated soil microbial composition (the 16S rRNA gene with bacterial primer pair) from bulk soil, paddy field under (C) and (O) land management and found distinct groups by the banding patterns. Our results showed that the soil bacterial diversity indices per sites were not significantly different between the loamy and sandy soil with two farming systems only in richness at clay soil (KC). Matsushita et al. (Citation2015) found that in an apple orchard the diversity of Alphaproteobacteria community evenness were significantly higher in (O) comparing to the (C) management. Li et al. (Citation2012) investigated Alphaproteobacteria from chernozem soil with clay texture. Their results indicated that the amount of Alphaproteobacteria were higher – but not significant – in (O) compare to (C) farming systems.
The most diverse bacterial community (H’, E) was found at sandy soil (NyC) where Rhizobium sp. was identified () which lives in symbiosis with the alfalfa crop (). The least diverse bacterial community (H’) was observed at clay soil (KO), where uncultured bacterial clones were identified belonging to the Rhizobiaceae family: Mesorhizobium sp. (Arora Citation2013) from sandy soil (NyO) is also a nitrogen-fixing soil bacteria. The other isolated samples 98–100% of similarity were shown with an uncultured bacterium clone (from the family of Rhizobiaceae, Acetobacteraceae, Rhodobiaceae and the phylum of Alphaproteobacterium). These members can play the main role in the decomposition of the organic matter and nitrogen mineralisation. The further isolated samples had medium level diversity indices. We detected Pseudovibrio denitrificans (Shieh et al. Citation2004) from loamy soil (MO), which is a facultatively anaerobic bacterium with rod-shaped cells capable for fermentation with a complete denitrification. Devosia sp. (Yoon et al. Citation2007) from loamy soil (MC) which is anaerobic, rod-shaped nitrogen-fixing soil bacteria and Ancylobacter rudongensis sp. nov. (Xin et al. Citation2004). Species-level classification was only obtained for loamy and sandy soil in both (C) and (O) land managements.
We came to the conclusion that in most cases the soil type and the crops should have a stronger impact on the soil bacterial composition than the farming system because of the loamy and clay soils in Martonvásár and Karcag are more fertile with greater humus content and better water management which provide favourable conditions for soil microbes. At sandy soil (Nyíregyháza) where the conditions for soil microbes are less favourable, due to the soil’s poorer water management, less nutrients availability and humus content and acidic conditions (Demeter et al. Citation2016), these factors consequently can result in less humic acid ratio in the isolated soil DNA. Despite their less favourable soil chemical parameters () we detected the most bands with the largest bacterial diversity in sandy soil (). Lopes et al. (Citation2011) reported also that sandy soil resulted in the highest richness (R = 25) during the DGGE analysis compared to other soil types. In this area detection of Mesorhizobium sp. and Rhizobium sp. were probably related to the cropping of leguminous plants. Loamy soil (M) was the least heterogeneous (), although the detected bacterial species - living with wheat and maize – capable nitrification (OF) and nitrogen fixation (CF). Despite a large number of isolated bands from clay soil (K) only uncultured bacterial clones could be identified. In addition to the soil type, the crop also influenced the bacterial diversity of the rizosphere.
Acknowledgements
Thanks are due to István Parádi, Ágota Horel and Mariann Mózes for their assistance. The data service of Mariann Makádi, Péter Mikó, Róbert Czimbalmos was highly appreciated.
Disclosure statement
No potential conflict of interest was reported by the authors.
Notes on contributors
Orsolya Gazdag is an assistant research fellow, in the Institute for Soil Sciences and Agricultural Chemistry, Centre for Agricultural Research, Hungarian Academy of Sciences, Department of Soil Biology. Her research focuses on the soil microbes system, specifically the N-fixing bacteria.
Tünde Takács is a senior research fellow, working for the Institute for Soil Sciences and Agricultural Chemistry, Centre for Agricultural Research, Hungarian Academy of Sciences, Department of Soil Biology.
László Ködöböcz is a research fellow, in the Institute for Soil Sciences and Agricultural Chemistry, Centre for Agricultural Research, Hungarian Academy of Sciences, Department of Soil Biology.
Gergely Krett is a research fellow, working for the Danube Research Institute, Centre for Ecological Research, Hungarian Academy of Sciences.
Tibor Szili-Kovács is a senior research fellow, working for the Institute for Soil Sciences and Agricultural Chemistry, Centre for Agricultural Research, Hungarian Academy of Sciences, Department of Soil Biology.
ORCID
Orsolya Gazdag http://orcid.org/0000-0003-2768-3430
Additional information
Funding
References
- Altschul SF, Madden TL, Schaffer AA, Zhang J, Zhang Z, Miller W, Lipman DJ. 1997. Gapped BLAST and PSI-BLAST: A new generation of protein database search programs. Nucleic Acids Res. 25:3389–3402. doi: 10.1093/nar/25.17.3389
- Arora NK. 2013. Plant microbe symbiosis: fundamentals and advances. 320. New Delhi: Springer.
- Bárány Á, Szili-Kovács T, Krett G, Füzy A, Márialigeti K, Borsodi AK. 2014. Metabolic activity and genetic diversity of microbial communities inhabiting the rhizosphere of halophyton plants. Acta Microbiol Imm H. 61(3):347–361. doi: 10.1556/AMicr.61.2014.3.8
- Bissett A, Richardson A, Baker G, Thrall P. 2011. Long-term land use effects on soil microbial community structure and function. Appl Soil Ecol. 51:66–78. doi: 10.1016/j.apsoil.2011.08.010
- Buzás I. 1988. Manual for soil and agrochemical analyses 2:physico-chemical and chemical analysis of soils. Budapest: Mezőgazdasági publisher (in Hungarian).
- Buzás I. 1993. Manual for soil and agrochemical analyses 1:physico, water management and mineral analysis of soils. Budapest: Inda publisher. (in Hungarian).
- Demeter I, Makádi M, Tomócsik A, Aranyos T, Posta K. 2016. Microbial community profiles in response to different soil managements in sandy soils. Agric For. 62(4):11–17.
- Diepeningen AD, de Vos OJ, Korthals GW, van Bruggen AHC. 2006. Effects of organic versus conventional management on chemical and biological parameters in agricultural soils. Appl Soil Ecol. 31:120–135. doi: 10.1016/j.apsoil.2005.03.003
- Ding GC, Heuer H, Smalla K. 2012. Dynamics of bacterial communities in two unpolluted soils after spiking with phenanthrene: soil type specific and common responders. Front Microbiol. 3(290):1–16.
- Ding GC, Piceno YM, Heuer H, Weinert N, Dohrmann AB, Carrillo A, Andersen GL, Castellanos T, Tebbe CC, Smalla K. 2013. Changes of soil bacterial diversity as a consequence of agricultural land use in a semi-arid ecosystem. PLoS One. 8(3):1–10.
- Edesi L, Järvan M, Noormets M, Lauringson E, Adamson A, Akk E. 2012. The importance of solid cattle manure application on soil microorganisms in organic and conventional cultivation. Acta Agriculturae Scandinavica, Section B – Soil & Plant Science. 62(7):583–594.
- FAO. 1998. World reference base for soil resources. World Soil Resources Report.
- Gomes NCM, Heuer H, Schönfeld J, Costa R, Mendonça-Hagler L, Smalla K. 2001. Bacterial diversity of the rhizosphere of maize (Zea mays) grown in tropical soil studied by temperature gradient gel electrophoresis. Plant Soil. 232:167–180. doi: 10.1023/A:1010350406708
- Hammer Ø, Harper DAT, Ryan PD. 2001. PAST: paleontological statistics software package for education and data analysis. Palaeo Electronica. 4(1):1–9.
- Hill GT, Mitkowskia NA, Aldrich-Wolfe L, Emelea LR, Jurkonie DD, Ficke A, Maldonado-Ramirez S, Lyncha ST, Nelsona EB. 2000. Methods for assessing the composition and diversity of soil microbial communities. Appl Soil Ecol. 15:25–36. doi: 10.1016/S0929-1393(00)00069-X
- Jeffery S, Gardi C, Jones A, Montanarella L, Marmo L, Miko L, Ritz K, Peres G, Römbke J, van der Putten WH. 2010. European Atlas of soil biodiversity. Luxembourg: European Commission, Publications Office of the European Union.
- Lakay FM, Botha A, Prior BA. 2006. Comparative analysis of environmental DNA extraction and purification methods from different humic acid-rich soils. J Microbiol. 102:265–273.
- Lauber CL, Hamady M, Knight R, Fierer N. 2009. Pyrosequencing-based assessment of soil pH as a predictor of soil bacterial community structure at the continental scale. Appl Environ Microbiol. 75(15):5111–5120. doi: 10.1128/AEM.00335-09
- Li R, Khafipour E, Krause DO, Entz MH, de Kievit TR, Fernando WD. 2012. Pyrosequencing reveals the influence of organic and conventional farming systems on bacterial communities. PloS one. 7(12):e51897. doi: 10.1371/journal.pone.0051897
- Lopes AR, Faria C, Prieto-Fernández Á, Trasar-Cepeda C, Manaia CM, Nunes OC. 2011. Comparative study of the microbial diversity of bulk paddy soil of two rice fields subjected to organic and conventional farming. Soil Biol Biochem. 43:115–125. doi: 10.1016/j.soilbio.2010.09.021
- Lynch JM, Benedetti A, Insam H, Nuti MP, Smalla K, Torsvik V, Nannipieri P. 2004. Microbial diversity in soil: ecological theories, the contribution of molecular techniques and the impact of transgenic plants and transgenic microorganisms. Biol Fertil Soils. 40(6):363–385. doi: 10.1007/s00374-004-0784-9
- Marinari S, Mancinelli R, Campiglia E, Grego S. 2006. Chemical and biological indicators of soil quality in organic and conventional farming systems in central Italy. Ecol Indic. 6:701–711. doi: 10.1016/j.ecolind.2005.08.029
- Matsushita Y, Bao Z, Kurose D, Okada H, Takemoto S, Sawada A, Nagase H, Takano M, Murakami H, Koitabashi M, et al. 2015. Community structure, diversity, and species dominance of bacteria, fungi, and nematodes from naturally and conventionally farmed soil: a case study on Japanese apple orchards. Org Agr. 5:11–28. doi: 10.1007/s13165-015-0096-4
- McCaig AE, Glover LA, Prosser JI. 1999. Molecular analysis of bacterial community structure and diversity in unimproved and improved upland grass pastures. Appl Environ Microbiol. 65(4):1721–1730.
- Niemi RM, Heiskanen I, Wallenius K, Lindström K. 2001. Extraction and purification of DNA in rhizosphere soil samples for PCR-DGGE analysis of bacterial consortia. J Microbiol Meth. 45:155–165. doi: 10.1016/S0167-7012(01)00253-6
- Orr CH, Leifert C, Cumming ST, Cooper JM. 2012. Impacts of organic and conventional crop management. PLOS ONE. 7(12):1–13. doi: 10.1371/journal.pone.0052891
- Plassart P, Terrat S, Thomson B, Griffiths R, Dequiedt S, Lelievre M, Regnier T, Nowak V, Bailey M, Lemanceau P, et al. 2012. Evaluation of the ISO Standard 11063 DNA extraction procedure for assessing soil microbial abundance and community structure. PLoS One. 7(9):e44279. doi:1371/journal.pone.0044279 doi: 10.1371/journal.pone.0044279
- Shieh WY, Lin YT, Jean WD. 2004. Pesudovibrio denitrificans gen. nov., sp. nov., a marine, facultatively anaerobic, fermentative bacterium capable of denitrification. Int J Syst Evol Microbiol. 54:2307–2312. doi: 10.1099/ijs.0.63107-0
- Singh SS, Schloter M, Tiwari SC, Dkhar MS. 2011. Diversity of community soil DNA and bacteria in degraded and undegraded tropical forest soils of North-Eastern India as measured by ERIC–PCR fingerprints and 16S rDNA-DGGE profiles. J Biol Environ Sci. 5(15):183–194.
- Székely AJ, Sipos R, Berta B, Vajna B, Hajdú C, Márialigeti K. 2009. DGGE and T-RFLP analysis of bacterial succession during mushroom compost production and sequence-aided T-RFLP profile of mature compost. Microb Ecol. 57:522–533. doi: 10.1007/s00248-008-9424-5
- Técher D, Martinez-Chois C, D’Innocenzo M, Laval-Gilly P, Bennasroune A, Foucaud L, Falla J. 2010. Novel perspectives to purify genomic DNA from high humic acid content and contaminated soils. Sep Purif Technol. 75:81–86. doi: 10.1016/j.seppur.2010.07.014
- Tsushima S, Matsushita Y. 2010. Technical report on the PCR-DGGE analysis of bacterial and fungal soil communities Ver. 3.3. Tsukuba: National Institute for Agro- Environmental Sciences.
- van der Heijden MGA, Bardgett RD, van Straalen NM. 2008. The unseen majority: soil microbes as drivers of plant diversity and productivity in terrestrial ecosystems. Ecol Lett. 11:296–310. doi: 10.1111/j.1461-0248.2007.01139.x
- Wang S, Cheng X. 2017. Changes in proteolytic bacteria in paddy soils in response to organic management. Acta Agriculturae Scandinavica, Section B – Soil & Plant Science. 67(7):583–589.
- Williamson KE, Kan J, Polson SW, Williamson SJ. 2011. Optimising the direct extraction of procariotic DNA from soils. Soil Biol Biochem. 43(4):736–748. doi: 10.1016/j.soilbio.2010.04.017
- Xin YH, Zhou YG, Zhou HL, Chen WX. 2004. Ancylobacter rudongensis sp. nov., isolated from roots of Spartina anglica. Int J Syst Evol Microbiol. 54:385–388. doi: 10.1099/ijs.0.02466-0
- Yeates C, Gillings MR, Davison AD, Altavilla N, Veal DA. 1998. Methods for microbial DNA extraction from soil for PCR amplification. Biol Proc Online. 1:40–47. doi: 10.1251/bpo6
- Yoon JH, Kang SJ, Park S, Oh TK. 2007. Devosia insulae sp. nov., isolated from soil, and emended description of the genus Devosia. Int J Syst Evol Microbiol. 57(6):1310–1314. doi: 10.1099/ijs.0.65028-0
- Yoon SH, Ha SM, Kwon S, Lim J, Kim Y, Seo H, Chun J. 2017. Introducing EzBioCloud: a taxonomically united database of 16S rRNA gene sequences and whole-genome assemblies. Int J Syst Evol Microbiol. 67:1613–1617. doi: 10.1099/ijsem.0.002404