ABSTRACT
Grain legumes, including cowpea, are the cheapest sources of minerals and protein to enhance human nutrition. Cultivar development and deployment of cowpea with increased grain mineral content and protein composition rely on selection of genetically unique and complementary breeding lines. The objective of this study was to assess the grain minerals and protein composition of diverse cowpea collections of eastern, southern and western Africa to select promising parents to develop a breeding population. Twenty-two genetically diverse cowpea genotypes were field evaluated using a randomised complete block design with three replications in two locations in South Africa. The dried grain mineral contents were determined using an Atomic Absorption Spectrophotometre, while the protein content was determined by the combustion method. Analyses of variance showed significant (P < 0.05) effects of genotypes, locations and their interactions. The test genotypes showed considerable variation for the following nine mineral contents: calcium (Ca), copper (Cu), iron (Fe), potassium (K), magnesium (Mg), manganese (Mn), sodium (Na), phosphorus (P), and zinc (Zn) varying from 0.07 to 0.12 mg kg−1; 4.46 to 7.81 mg kg−1; 0.06 to 0.11 g; 11.82 to 14.45 g; 1.86 to 2.27 g; 9.24 to 13.30 mg kg−1; 0.26 to 0.80 g; 4.63 to 5.92 g and 0.03.00 to 0.05 g, respectively. Further, the total protein content varied from 23.16 to 28.13%. Significant correlations were detected among some mineral elements and total protein content, suggesting the possibility of simultaneous selection for these traits. The principal component analysis (PCA) identified four principal components (PCs) contributing to 70.93% of the total explained variation amongst genotypes. Overall the following genotypes with desirable grain mineral and protein attributes were selected: IT90K-59 (Ca), 98K-5301 (Ca and protein), ITOOK-1060 (Cu), ITOOK-1217 and IT845-2246 (Fe), Bensogla (K, Na, and P), TVU11424 and ITOOK-1217 (Mg), CH14 (Mn and Na), TVU12637 (Mn) and Glenda and Vuli (Zn). The selected cowpea genotypes are useful genetic resources for population and cultivar development for grain nutrients composition.
Introduction
Cowpea (Vigna unguiculata L. Walp.; 2n = 2x = 20) is a multipurpose grain legume widely cultivated in sub-Saharan Africa (SSA), Asia, South America and part of Southern Europe and United States (Singh et al. Citation1997; Timko and Singh Citation2008). It is one of the most drought tolerant crops thriving under variable and harsh growing conditions in arid and semi-arid tropics globally (Timko et al. Citation2007; Adewale et al. Citation2010). The dried grains, fresh green seeds, immature pods and leaves are used for food (Singh Citation2014).
In SSA, including South Africa, cowpea is a valuable component of the farming systems of the smallholder farmers. The young leaves and succulent immature pods are eaten as leafy vegetables and the dry seeds are valued for snacks and main meal dishes in South Africa. Cowpea is a good source of protein, essential minerals including Ca, Cu, Fe, K, Mg, Mn, Na, P, and Zn vitamins (vitamins A, C, B1, B2, B5, B6 and B9), carbohydrates and antioxidants that are essential for human health, growth, and development. Macro- and micro-nutrients are essential for humans to perform normal physiological functions (Berdanier et al. Citation2016). Nutritionally enhanced plant varieties provide considerable amounts of bioavailable nutrients useful to alleviate nutrient deficiency among rural and urban populations. Plant derived mineral and protein nutrition is the cheapest alternative to circumvent malnutrition that is prevalent in SSA.
Vitamin A and Zn deficiencies are common in South Africa and have become part of the ‘hidden hunger’, causing serious constraints to human health. Unbalanced growth and body weight are some the most common nutritional disorders caused by malnutrition. Improving the nutritional quality of cowpea is, therefore, important to alleviate nutritional deficiency. In South Africa, cereal based foods are mostly supplemented with mineral elements such as Fe, Zn, Mn, and selenium (Se) in order to alleviate micronutrient deficiency in children and pregnant women (Schönfeldt and Gibson Citation2009). It was also reported that most of the smallholder farmers in the country are highly dependent on cowpea leaves, immature pods and grain to meet their mineral and protein requirements (Belane and Dakora Citation2012; Gerrano et al. Citation2015).
The presence of genetic diversity among cowpea genotypes for the different minerals and protein is vital to improve its nutritional quality through breeding (Ng’uni et al. Citation2012; Shegro et al. Citation2012; Gerrano et al. Citation2015; Citation2017) towards contributing to food and nutritional security. Carnovale et al. (Citation1990) reported the nutritional characteristics in cowpea and they found that the mean concentrations for Ca, Zn, P and K contents in grains were 37.00, 4.70, 430.00 and 125.00 mg 100 g−1, respectively. Therefore, cowpea genotypes can be selected with desirable nutritional contents through characterisation of the available genetic resources.
Nutritional analysis of diverse cowpea genotypes on the basis of grain mineral elements and crude protein content is essential in the identification of potential candidate parental genotypes to develop nutritionally enhanced cowpea cultivars. Improvement of nutritional value of cowpea is therefore, important and will contribute to alleviating nutritional deficiency in SSA. Therefore, the objective of the current study was to assess the genetic variability among cowpea genotypes collected from southern and western Africa for mineral and protein content in the grains and to identify unique and complementary parental genotypes with high minerals and protein contents to develop a breeding population.
Material and methods
Plant materials and description of study area
Seeds of 22 cowpea genotypes () were obtained from the genebanks of the Agricultural Research Council (ARC), Vegetable and Ornamental Plants (VOP- Pretoria) and Grain Crops (GC-Potchefstroom), South Africa. The cowpea genotypes were acquired from the International Institute for Tropical Agriculture (IITA) originally assembled from southern and western Africa. The experiments were conducted at the Roodeplaat research farm of VOP-Pretoria (25°604′ S; 28°345′ E) and at Syferkuil, the University of Limpopo’s experimental station situated at 23°50′ S; 29°40′ E. The two sites are located in Gauteng and Limpopo provinces, respectively. The experiments were conducted in the 2014 cropping season. Planting was done during mid-November. The altitude of Roodeplaat research farm is 1168 metre above sea level (masl) and the trial received a total rainfall of 386.56 mm. The average minimum and maximum recorded temperature during the growth period was 14.61°C to 29.58°C, respectively, with a maximum temperature of 36.37°C in January. At this site the average relative humidity during the growth period was 88.44%. The altitude of Syferkuil is 1244 masl. At this location, a total rainfall of 526.30 mm was received during the growing period. The average minimum and maximum temperatures for the site was 13.30°C and 28.01°C, respectively with a maximum temperature of 36.48°C in October and November during the flowering stage. The average relative humidity during the growth period was 88.66%, similar to that of the Roodeplaat site.
Table 1. Description of cowpea genotypes tested in the study.
Experimental design and trial establishment
Field trails were established in both sites using a randomised complete block design with three replications. The experimental plots consisted of four rows of 4 m long separated by 1 m spacing. The spacing within the rows was 0.40 m and spacing between replications was 1.5 m. Two seeds were sown in each hole and the seedlings were thinned to one at two weeks after planting. Standard cowpea agronomic management practices were followed at both locations.
Analysis of minerals and protein contents
The mature cowpea seeds were harvested from each genotype at 12.5% moisture contents. The seeds were manually sorted and cleaned to remove any extraneous materials. The seeds were then oven dried at 80°C for 24 hours to reduce the moisture content. The dried seeds were ground using a laboratory seed grinder and then sieved using a 0.5 mm sieve. From this 0.5 g flour was sampled for analysis. Mineral content and protein analysis was conducted at the ARC Soil, Water and Climate analytical laboratory, Pretoria, South Africa. The concentration of nine mineral elements, including Ca, Cu, Fe, K, Mg, Mn, Na, P and Zn were determined using an Atomic Absorption Spectrophotometre (Spectra AA 300). Crude protein content (N × 6.25) was determined by the combustion method (Leco ®model, FP-528, St. Joseph, Ml).
Data analysis
The data set was subjected to analysis of variance (ANOVA) using GenStat for Windows 17th edition (VSN, International Hempstead, UK) (Payne et al. Citation2016) statistical software. Correlation and principal component analysis for mineral elements and protein contents was done using GenStat for Windows 17th edition (VSN, International Hempstead, UK) (Payne et al. Citation2016). Shannon-Weaver (H’) index as a measure of diversity was computed for mineral elements and protein. The data set was further subjected to principal component analysis (PCA) using GenStat for Windows 17th edition (VSN, International Hempstead, UK) (Payne et al. Citation2016). Furthermore, cluster analysis using the mean data combined over the two experiments was performed based on Jaccard’s dissimilarity matrix distance measures. A dendrogram was then generated based on the Jaccard’s dissimilarity matrix using DARwin 5.0 software (Perrier and Jacquemoud-collet Citation2006). The dendrogram was generated based on the neighbor-joining algorithm using the unweighted pair group method (UPGM). Bootstrap analysis was performed for node construction using 10 000 bootstrap values.
Results
Genetic variation and association of traits
ANOVA per test location revealed highly significant differences (p < 0.001) among genotypes for all measured characteristics, suggesting the existence of considerable genetic diversity (). The genotype IT90K-59 showed the highest concentration of Ca (0.18 mg kg−1, and 0.13 mg kg−1) at Syferkuil and Roodeplaat, respectively. Genotype 98K-5301 (0.13 mg kg−1), similarly revealed the highest concentration at the Roodeplaat site, which was also the second genotype in Ca concentration at Syferkuil. The genotype Encore and PAN311 recorded Ca content of 0.12 mg kg−1 at both sites, while Glenda had 0.12 mg kg−1 and 0.11 mg kg−1 at Roodeplaat and Syferkuil, respectively. Genotype TVU11424 expressed Ca content of 0.11 mg kg−1 at Roodeplaat and 0.10 mg kg−1 at Syferkuil. These genotypes showed consistent and above average values for Ca across locations and can be selected as candidate parents for breeding. Genotype IT90K-76 showed the highest concentration of Cu (7.05 mg kg−1) and Mn (13.49 mg kg−1); while Vuli showed the highest concentration of P (5968.00 mg kg−1), Zn (64.57 mg kg−1) and protein (27.17%) and Bensogla had the highest concentration of K (14832.00 mg kg−1) and Na (1072.70 mg kg−1) at Syferkuil. Similarly, at Roodeplaat, genotype Mamlaka displayed the highest concentration of Mn (16.70 mg kg−1) and protein (31.17%); TVU13953 showed the highest concentration of Fe (86.51 mg kg−1) and Zn (48.10 mg kg−1) and Bensogla showed the highest concentration of K (14072 mg kg−1), Na (523.50 mg kg−1) and P (6160.00 mg kg−1) (data not presented). These genotypes showed consistent and above average values for across locations and can be selected as candidate parents for breeding for their respective sites. In general, the mean concentration of all the minerals were higher at Syferkuil, than at Roodeplaat except for Cu, Mn and protein (data not presented).
Table 2. Partial analysis of variance showing mean square values and significant tests for nine grain mineral and protein parameters among 22 cowpea genotypes evaluated at two locations in South Africa.
Some of the mineral elements and total protein content were significantly influenced by the genotype, location and the interaction effects (). All the characteristics revealed highly significant variations (p < 0.001) among the cowpea genotypes, signifying the influence of the genetic factor on the expression of the characteristics. Location effects were highly significant for all minerals except K, Mg and P. Highly significant genotype by location interactions (p < 0.001) were observed for all the characteristics except K and total protein content (). The average performance of the cowpea genotypes combined over locations for nutritional characteristics are shown in . The total protein content ranged from 23.16% in genotype Bechuana white to 28.13% in Vuli, with a mean value of 26.07%. The genotypes Vuli, Mamlaka, IT90K-59, Ngoji, TVU13953 and 98K-5301 had the highest total protein content over locations ().
Table 3. Mean responses for nine grain mineral elements and protein content in 22 cowpea genotypes evaluated in two locations.
The concentration of the mineral elements significantly varied between the two sites due to the variation in the genotypic make up as well as environmental factors. The concentration of Ca was significantly influenced by genotype, location and their interaction and varied from 0.07 to 0.16 mg kg−1 with an average value of 0.11 mg kg−1 ( and ). The highest concentration of Ca was found in genotype IT90K-59 followed by 98K-5301 and Bensogla. The highest concentration of Cu was found in the genotype ITOOK-1060 (7.81 mg kg−1). The Fe concentration varied from 60.64 in Bechuana white to 105.94 mg kg−1 in genotype ITOOK-1217, with an average mean value of 74.64 mg kg−1. The Fe concentration recorded for ITOOK-1217 was almost double that recorded for Fahari which had the lowest concentration, indicating the wider variation exhibited among the genotypes ().
The tested cowpea genotypes are rich in K, which varied from 11824 to 14452 mg kg−1 with the highest density found in genotype Bensogla. This genotype also displayed the highest concentration of other mineral elements such as Na (798.10 mg kg−1) and P (5923.93 mg kg−1) with average mean values for crude protein. The highest concentration of Mg (2273.70 mg.kg−1) was recorded in genotype TVU11424, with a mean value of 2064.40 mg kg−1. The concentration of Zn ranged from 32.63 to 51.08 mg kg−1 with a mean value of 40.16 mg kg−1. The highest density of Zn was found in genotype Vuli followed by genotypes Glenda, IT90K-76 and TVU11424. Bensogla had the highest concentration for P, K and Na; while IT90K-59 showed the highest concentration for Ca, Mn and total protein contents at both locations, suggesting this genotype could be selected as an ideal genotype for improvement of concentration of these specific nutrients. Genotypes IT845-2246 and ITOOK-1217 showed the highest concentration for Fe across locations ().
Pairwise trait associations between different cowpea grain minerals and protein contents are presented in . Total grain protein content showed highly significant positive association (P < 0.001) with Ca, but highly significant and negative correlation with Cu, Fe, K and Mg. This suggested that an increase in Cu, Fe, K and Mg concentration would result in a decrease in total protein content in the grains of cowpea. Similarly, K was found to be positively and significantly (P < 0.001) correlated with P, Na and Mg. Fe was positively and significantly correlated with Cu (r = 0.47, p < 0.001) and Mg (r = 0.38, p = 0.01), but negatively correlated with Mn (r = −0.24, P = 0.05) and protein (r = −0.42, p = 0.01). Mn and Mg revealed significant negative correlation (r = −30, P = 0.01); while P and Na showed positive significant correlation (r = 37, P = 0.01).
Table 4. Pair-wise correlation coefficients indicating the association of nine mineral elements and total protein content in 22 cowpea genotypes evaluated in two locations.
Diversity indices and principal component analysis
summarises diversity index, eigenvalues, percentage of total variance, percentage of cumulative variance and eigenvectors for the mineral composition and total protein in grain of cowpea genotypes. Overall, the studied cowpea genotypes showed considerable variability with diversity index scores ranging from 0.86 to 0.91 with respect to characteristics evaluated. The Shannon-Weaver diversity (H’) index varied from 0.86–0.91, with an average value of 0.88, suggesting the presence of high genetic diversity due to these measured characteristics. The genotypes showed high diversity for Cu and Na. However, total grain protein showed relatively lower levels of variation. All the individual mineral elements and protein characteristics showed a different pattern of variation and were highly polymorphic. Thus, the diversity among cowpea genotypes varied depending on these nutritional characteristics. PCA was performed to assess the relative importance of each characteristic towards explaining the prevalent genetic variance in the studied genotypes (). Considering a minimum threshold eigenvalue of one, the 10 measured characteristics were reduced to four PCs that explained 70.93% of the total variation observed in the cowpea genotypes (). Hence, the first four PCs, each with eigenvalues ≥ 1.00 explained 70.93% of the total variation among the studied cowpea genotypes for mineral composition and total grain protein (). Most of the genetic variation (26.43%) observed was explained by the first principal components (PC1), followed by PC2 (18.74%). PC1 was due to the contrasting effect of total protein content with high negative loadings and Mg with high positive loadings. The mineral elements Cu and Fe also contributed high variances in this PC. In PC2, K and P made significant contributions with high positive loadings; however, Fe also contributed significant variance to this PC with a negative loading. The PC3 that accounted about 14% of the total variance among the genotypes constituted mainly of the mean effects of Ca and Na that revealed strong positive loadings and associations. The crude protein also had a high variance contribution to PC3 with positive loadings. PC4 (11.76%) was found to be strongly and positively associated with Zn. Na played a significant role in PC3 and PC4 with equal values of positive and negative eigenvectors loading, respectively ().
Figure 1. Principal components 1 (PC1) and 2 (PC2) showing the spatial distribution of the 22 cowpea genotype based on nine seed mineral elements and protein content; Pro = protein; Ca = calcium; Cu = copper; Fe = iron; K = potassium; Mg = magnesium; Mn = manganese; Na = sodium; P = phosphorus; Pro = protein; Zn = zinc.
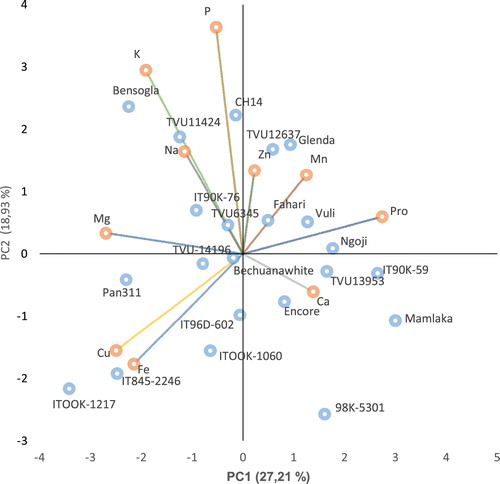
Table 5. Diversity index and eigenvectors within principal components involving nine mineral seed elements and total protein content in 22 cowpea genotypes evaluated in two locations.
Cluster analysis
presents the clustering pattern of the 22 cowpea genotypes. Cluster analysis constructed based on Jaccard’s index in DARwin classified the 22 cowpea genotypes into three distinct groups . The distinctiveness of the clustering patterns of the genotypes was confirmed by the high cophenetic correlation coefficient (r = 0.84) for the measured characteristics. The first cluster consisted of 5 (22%) of the genotypes and was characterised by high concentrations of Cu, K, Mg, Na, P and Zn, the second cluster consisted of 7 (31%) and displayed high Ca concentration and high total grain protein content but were low in concentration of other mineral elements, and the third cluster consists of 10 (45%) of the genotypes characterised by higher concentrations of Fe and Mn compared to the other clusters.
Figure 2. Dendrogram based on UPGMA depicting the genetic relationship among 22 cowpea genotypes using seed mineral elements and protein content; Pro = protein; Ca = calcium; Cu = copper; Fe = iron; K = potassium; Mg = magnesium; Mn = manganese; Na = sodium; P = phosphorus; Pro = protein; Zn = zinc.
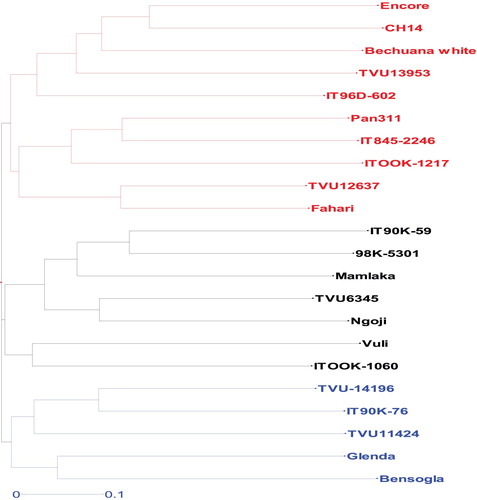
Discussion
Genetic variability of cowpea for mineral elements and protein content
Cowpea is an important but underutilised legume crop, which is contributing to food and nutritional security in SSA. Highly significant genotype by location interactions (p < 0.001) were observed for all the characteristics except potassium and total protein content (). Ye et al. (Citation2006) reported genotype by location interactions and suggested the existence of different patterns of genetic associations across environments. Similarly, Allard and Bradshaw (Citation1964) reported the complex nature of mineral and protein content which was affected by genotype by location interactions. Moreover, Vandemark et al. (Citation2018) reported the effect of genotype by location interactions on the concentration of mineral elements in chickpea and lentils grown in Washington and Idaho, USA.
The present study assessed genetic variability of 22 cowpea genotypes for mineral elements and grain crude protein content under two environmental conditions. The cowpea genotypes showed a wide range of genetic variability for the mineral elements and protein content. This genetic variability would contribute to achieving food security and improved nutrition towards sustainable production and conservation of biodiversity of cowpea. Significant genetic diversity was observed among the genotypes in their genetic potential to absorb the mineral elements available from the soil and translocate them into the plant system for different physiological processes and functions (Singh et al. Citation1997; Liu et al. Citation2003; Jamali et al. Citation2008; Badigannavar et al. Citation2016) and finally to the grain. The diversity present among the genotypes for the mineral elements and protein content suggested their importance in cowpea breeding. Similarly, Owolabi et al. (Citation2012) reported significant differences among five cowpea genotypes evaluated in Nigeria for mineral element and crude protein. The genotypes showed highly significant variations in the concentration of Cu, Mn, Na, Zn and Ca as well as crude protein content between the two locations, indicating the genotypes reacted differently in different environments (). At Syferkuil, genotype PAN311 was superior in K and Cu, while Vuli was found to be high in Zn, P and crude protein (data not presented). Similarly, genotype IT90K-76 had high concentrations of Mn, and Zn, above average P concentration (5428 mg kg−1) and TVU12637 displayed high concentrations of Mn, Mg and P. At Roodeplaat, on the other hand, genotype Mamlake was found to be higher in Mn and crude grain protein content, Encore in Mn and Zn, while genotype TVU13953 was higher in Fe and Zn (data not presented). The highest average absorption and translocation of mineral concentration and protein content into the plant system was observed at Syferkuil site with the total amount of 2127.14 compared to that of Roodeplaat with 2097.40 () indicating the quick dilution of mineral elements in the soil and their availability in usable forms for absorption by the root system and translocation into the grain through the plant body (Seetharama et al. Citation1987; Johnson and Larry Citation2008; Shegro et al. Citation2012; Badigannavar et al. Citation2016; Phuke et al. Citation2017).
The grain of genotype Bensogal displayed higher concentrations of Ca, K, P, Na and Mg across locations (), suggesting this genotype is a suitable genotype to improve the nutrient concentration in cowpea through biofortifcation. The variation observed among the genotypes was partly due to genetic potential of the genotypes and due to the growing environment. These results agree with the finding by Owolabi et al. (Citation2012). The mean nutrient concentration of the grain grown at Syferkuil were higher for all nutrients except for Cu, Mn and crude protein than at the Roodeplaat site (), indicating this location was favourable for obtaining high concentrations of the studied mineral nutrients.
Okareh et al. (Citation2015) reported that protein is an essential nutritional component of the diet of humans. The protein content in cowpea grain is rich in amino acids such as lysine and tryptophan, compared to other cereal grains such as sorghum, maize, rice and other (Timko et al. Citation2007). In the present study, the protein content of cowpea grain ranged from 23.16% to 28.13% with the highest values found in genotypes Mamlaka, IT90K-59, Vuli, Ngoji, and TVU13953 (). These genotypes can also be selected for formulating infant feeds (Owolabi et al. Citation2012). Mamiro et al. (Citation2011) reported that the protein content of cowpea genotypes evaluated in Tanzania ranged from 22.01 to 26.00%, while in Nigeria it ranged from 19.84 to 26.61% (Owolabi et al. Citation2012). The crude protein content in this study was much lower (46.51%) than that reported by Adeyemi et al. (Citation2012). The values of the crude protein content obtained in this study was in agreement with other studies (Ghaly and Alkoaik Citation2010; Vasconcelos et al. Citation2010; Boukar et al. Citation2011; Tchiagam et al. Citation2011).
Total protein was highly influenced by location that affected the vegetative and physiological growth of the crop, whereas the interaction of genotype and location had no influence on the characteristics (Adeyemi et al. Citation2012). This indicated that the protein content of cowpea grain grown under limited soil moisture is lower than that of cowpea grain produced with plentiful soil moisture. This could explain the difference in mean protein values for Syferkuil (24.47%) and Roodeplaat (27.66%). Similarly, Owolabi et al. (Citation2012) observed the differences among the tested cowpea genotypes for the crude protein in Nigeria.
Most smallholder famers in Africa cannot afford to obtain protein from meat, therefore additional protein sources are needed to supplement their diets. Protein deficiency has been reported as one of the main causes of malnutrition in children and causes marasmus and kwashiorkor (Ghaly and Alkoaik Citation2010). Most of the cowpea varieties being grown contain between 22 and 25% crude protein in their grains (Carnovale et al. Citation1990; Diouf Citation2011). Hence, cowpea can be a cheap source of protein in the people’s diets and the protein levels can be further enhanced. However, only about 80% of the protein is digestible (Marconi et al. Citation1990). Breeding high yielding cowpea varieties with improved nutritional quality such as high protein content and high protein digestibility through biofortification is vital in order to meet the protein requirements of Africa’s populations who depend on this important grain.
The highest density of Ca was obtained in genotypes IT90K-59 (0.16 mg kg−1), 98K-5301 (0.14 mg kg−1) and Bensogla (0.13 mg kg−1) (). Stanley (Citation1995) reported that the mineral elements, particularly Ca, plays a major physiological role in the growth and development of plant meristems, root hairs and root tips. It is also plays a vital role in human bone growth, development, health and strength (Samia et al. Citation2005). Shegro et al. (Citation2012) and Seetharama et al. (Citation1987) reported that variation in the ability of crops to absorb mineral elements varied due to the genetic differences in their physiological functions. The Ca values recorded in the present study were lower than those previously reported by Belane and Dakora (Citation2012) and Mamiro et al. (Citation2011) in cowpea genotypes in Ghana and Tanzania, respectively. This might be due to the genotype and environmental variations that prevailed during the growth and development period of the plants. However, the same genotypes (Bensogla, CH14, Fahari, Glenda, IT90K-76, Mamlaka, Ngoji, PAN311 and TVU11424) were tested in Ghana and showed higher concentrations of Ca as a result of probably conducive environmental conditions that favoured the high absorption and translocation of this mineral element into the plant system for different functions.
It was reported that Cu is essential to all living organisms as a trace dietary mineral element. It is a fundamental constituent of the respiratory enzyme complex which helps biochemical reactions in the cell, involved in the absorption, translocation, storage and metabolism of iron (Johnson and Larry Citation2008). It is an essential micro-nutrient which is necessary for the haematologic and neurologic systems (Tan et al. Citation2006). In the present study, significant genetic variability was observed among the cowpea genotypes and the highest Cu density was obtained in genotype IT00K-1060 and PAN311 (). The values recorded were similar to those reported earlier by Jaryum et al. (Citation2013), but higher than the values reported by Belane and Dakora (Citation2012).
The concentration of Fe varied from 62.16 mg kg−1 in Fahari to 105.94 mg kg−1 in ITOOK-1217 () which was higher than the values reported by Belane and Dakora (Citation2012) and Mamiro et al. (Citation2011). The present study indicated that cowpea genotypes ITOOK-1217 and IT845-2246 showed the highest Fe concentration. Genotypes CH14, Mamlaka, IT90K-59, IT96D-602 and TVU6345 had a similar concentration of iron. This indicated that they had some common genetic characters in the uptake, translocation and the biological processes of this mineral element (Seetharama et al. Citation1987; Shegro et al. Citation2012). Genotypes with high concentrations of micro-nutrients such as Fe, Zn and Mn in grain could be selected as potential parents for biofortification through breeding (Cakmak Citation2008) to meet the micronutrient requirements for health, growth and development. Furthermore, the genotypes with high concentration of Fe can be formulated for baby foods for growth and development (Owolabi et al. Citation2012; Okareh et al. Citation2015) and could be used for functional foods towards improving food and nutritional quality (Badigannavar et al. Citation2016).
In the current study, significant genetic variability was observed in the concentration of K, Na and P among the genotypes and the highest concentration was recorded in genotype Bensogla (). The values obtained in this study for K, Na and P were within the range of values reported by Boukar et al. (Citation2011). P is an essential mineral element in human nutrition and plays an important role in the structure and function of the human body. The concentration of P and Mn varied significantly among the genotypes evaluated across locations (). The highest values observed for Mn were in genotype TVU12637 (13.78 mg kg−1) and CH14 (13.08 mg kg−1). Seetharama et al. (Citation1987) reported that the highest concentration of mineral elements in the gain have come from genotype’s ability to absorb and distribute in the grain.
Correlations among mineral elements and protein content in cowpea
Positive and significant association was observed between Ca and crude protein (). Ca played an important role in protein synthesis in plants. Therefore, selection of correlated traits directly influences each other allowing the breeder for simultaneous selection in the breeding programme (Rukundo et al. Citation2013). Breeding for high crude protein content will therefore result in increased concentration of Ca. However, significant negative correlations were observed between crude protein content and Cu, Fe, K and Mg indicating selection for crude protein content decreases the concentration of Cu, Fe, K and Mg. Mg was strongly and positively associated with Cu, K and Fe content but Mg had strong negative association with Mn. Significant positive correlations were observed between K and Na or P, between Na and P and between P and Zn. This may be due to the fact that these elements have similar chemical properties and they compete for the site of absorption, transport, and function in plant tissues (Walker et al. Citation1977; Robson and Pitman Citation1983). Hence, the selection of any one of these mineral elements will lead to increased concentration of the other mineral elements, thereby enhancing nutritional values in cowpea.
Principal component and cluster analyses of mineral elements and protein content in cowpea
PCA was used to visualise this data set in a two dimensional biplot (). In the current study, the PCA showed the first four latent vectors had latent roots higher than one, indicating that significant variability existed among the cowpea genotypes evaluated for the mineral elements and crude protein. Chatfield and Collin (Citation1980) and Hair et al. (Citation1998) suggested that eigenvalues (latent roots) higher than one, could be considered as significant and component loadings (latent vectors) higher than ±0.30 were considered to meaningfully explain variation. The positive and negative sign of the loading showed the direction of the relationship between the components and the variables in the four quadrant (Johnson Citation1998). In the present study, the four PCs (eigenvalue >1) are important and cumulatively account for 70.93% of the total variation among the cowpea genotypes ().
The average H’ value of 0.88 () obtained in the present study suggests the presence of high genetic diversity (Eticha et al. Citation2005) among the genotypes.
In the PCA biplot (), the genotypes were scattered in four quadrants indicating that there was high genetic diversity among them due to the measured nutritional traits. The biplot showed the association of the mineral elements, protein and the cowpea genotypes, with the principal components. Mwadzingeni et al. (Citation2016) reported the presence of a high association of traits in discriminating genotypes that have small angles between dimensions vectors in the same direction. In this study, a very close association was observed between Na and K, and between Cu and Fe indicating their positive interaction in the plant system (, ). The genotypes located at the top right quadrant were characterised and associated with high concentration of Zn, Mn, and crude protein content ( and ). The cowpea genotypes found at the left top quadrant contain high values for P, K, Mg and P. The cowpea genotypes clustered at the bottom left quadrant were characterised based on the high concentration of Cu and Fe with positive and significant association. These groups of genotypes were identified as potential and candidate parental genotypes for improvement of these two mineral elements in the development of population in the breeding programme. The last quadrant, located at the right bottom quadrant consisted of genotypes associated only with high concentration of mineral element, Ca. In this quadrant, the genotype Mamlake had average mean concentration of this mineral element (of South Africa origin), with the highest concentration in IT90K-59 from West Africa originally. Similarly, Gerrano et al. (Citation2015, Citation2017) found the same clustering patterns of cowpea genotypes using biplots for the mineral elements and protein content in the leaves and immature pods of cowpea in South Africa.
Cluster analysis using a neighbor-joining algorithm using the unweighted pair group method (UWPGM) revealed different clustering patterns of genotypes (). The clustering using UWPGM based on ten measured characteristics grouped the 22 cowpea genotypes into three distinct clusters, indicating the cowpea genotypes exhibited notable genetic divergence in terms of measured traits. The members of the first cluster consisted of five genotypes: TVU-14196, IT90K-76, TVU11424, Glenda and Bensogla, which were characterised by high concentrations of Cu, K, Mg, Na, P and Zn and were mostly from Southern and Western Africa. The second cluster was composed of seven cowpea genotypes that displayed high Ca concentration and high total grain protein content but were low in concentration of other mineral elements. This cluster comprised the cowpea genotypes from southern, western and eastern regions. The third cluster consisted of ten genotypes and was characterised by higher concentration of Fe and Mn compared to other clusters. Forty one percent of the genotypes in this cluster were from the western part of the country followed by Southern African genotypes. The members of this cluster showed average concentrations in most of the characteristics such as Cu, K, Mg, Na, P and Zn. These genotypes could be used as a source of genes to improve the concentration of mineral elements in cowpea breeding programmes. Parents belonging to genetically different clusters can be used to identify heterotic groups towards development of breeding populations with favourable nutritional characteristics (Souza and Sorrells Citation1991; Hasanuzzaman et al. Citation2002; Gerrano et al. Citation2017; Mhlaba et al. Citation2018).
The present study found a wide range of genetic diversity among the 22 cowpea genotypes that were evaluated for concentration of selected mineral elements and crude protein content. Of the mineral elements studied P, K, Mg, and Na were the most abundant in the cowpea grain, while Ca, Cu, Fe, Mn, and Zn were found in smaller quantities. The following genotypes were identified with desirable grain mineral and protein attributes: IT90K-59 for increased Ca content, 98K-5301 (Ca and protein), ITOOK-1060 (Cu), ITOOK-1217 and IT845-2246 (Fe), Bensogla (K, Na, and P), TVU11424 and ITOOK-1217 (Mg), CH14 (Mn and Na), TVU12637 (Mn) and Glenda and Vuli (Zn). The identified cowpea genotypes are useful genetic resources for breeding population development and cultivar release emphasising enhanced grain nutrients composition.
Disclosure statement
No potential conflict of interest was reported by the authors.
Notes on contributors
Abe Shegro Gerrano is a researcher at the Agricultural Research Council. Currently, he is developing superior cowpea genotypes for biofortification.
Willem Jansen van Rensburg a researcher at the Agricultural Research Council. He is a co-author for the current study.
Sonja Venter a Senior Manager for the Vegetable and Ornamental Plants at the Agricultural Research Council. She is a co-author for the current study.
Nemera Shargie is a senior researcher at the Agricultural Research Council. He is a co-author for the current study.
Amelework Beyene is post-doctoral scholar in plant breeding African Center for Crop Improvement (ACCI), University of KwaZulu-Natal, Pietermaritzburg, South Africa. She is a co-author for the current study.
Hussein Shimelis is a Professor of Plant Breeding at the University of KwaZulu-Natal in South Africa. He is a co-author for the current study.
Maryke Labuschagne is a Professor of Plant Breeding and Genetics at the University of the Free State in South Africa. She is a co-author for the current study.
ORCID
Abe Shegro Gerrano http://orcid.org/0000-0001-7472-8246
Willem S. Jansen van Rensburg http://orcid.org/0000-0002-5684-6174
Nemera G. Shargie http://orcid.org/0000-0003-2590-0999
Maryke T. Labuschagne http://orcid.org/0000-0003-0593-2678
Additional information
Funding
References
- Adewale B, Okonji C, Oyekanmi A, Akintobi D, Aremu C. 2010. Genotypic variability and stability of some grain yield components of cowpea. Afr J Agric Res. 5:874–880.
- Adeyemi SA, Lewu, FB, Adebola PO, Bradley G, Okoh AI. 2012. Protein content variation in cowpea genotypes (Vigna unguiculata L. Walp.) grown in the Eastern Cape province of South Africa as affected by mineralised goat manure. Afr J Agric Res. 7:4943–4947.
- Allard RW, Bradshaw AD. 1964. Implications of genotype-environmental interactions in applied plant breeding1. Crop Sci. 4:503–508. doi: 10.2135/cropsci1964.0011183X000400050021x
- Badigannavar A, Girish G, Ramachandran V, Ganapathi TR. 2016. Genotypic variation for seed protein and mineral content among post-rainy season-grown sorghum genotypes. Crop J. 4:61–67. doi: 10.1016/j.cj.2015.07.002
- Belane AK, Dakora FD. 2012. Elevated concentrations of dietarily-important trace elements and macronutrients in edible leaves and grain of 27 cowpea (Vigna unguiculata L. Walp.) genotypes: implications for human nutrition and health. Food Nutr Sci. 3:377–386.
- Berdanier CD, Dwyer JT, Heber D. 2016. Handbook of nutrition and food. 3rd ed. CRC Press; p. 211–224. ISBN 978-1-4665-0572-8.
- Boukar O, Massawe F, muranaka S, Franco J, Maziya-Dixon B, Singh B, Fatokun C. 2011. Evaluation of cowpea germplasm lines for protein and mineral concentrations in grains. Plant Genet Resour. 9:515–522. doi: 10.1017/S1479262111000815
- Cakmak I. 2008. Enrichment of cereal grains with zinc: agronomic or genetic biofortification?. Plant Soil. 302:1–17. doi: 10.1007/s11104-007-9466-3
- Carnovale E, Marletta L, Marconi E, Brosio E. 1990. Nutritional and hydration properties in cowpea. In: Ng NQ, Monti LM, editors. Cowpea genetic resources. Ibadan: IITA; p. 111–118.
- Chatfield C, Collin AJ. 1980. Introduction to multivariate analysis. Published in the USA by Chapman and Hall in Association with Methuen, Inc., 733 Third Avenue, New York NY. 10017.
- Diouf D. 2011. Recent advances in cowpea (Vigna unguiculata (L.) Walp.) “omics” research for genetic improvement. Afr J Biotechnol. 10:2803–2819. doi: 10.5897/AJBx10.015
- Eticha F, Bekele E, Belay G, Börner A. 2005. Phenotypic diversity in tetraploid wheats collected from Bale and Wello regions of Ethiopia. Plant Genet Resour. 3:35–43. doi: 10.1079/PGR200457
- Gerrano AS, Adebola PO, Jansen van rensburg WS, Venter SL. 2015. Genetic variability and heritability estimates of nutritional composition in the leaves of selected cowpea genotypes [Vigna unguiculata (L.) Walp.]. HortScience. 50:1435–1440.
- Gerrano AS, Jansen van Rensburg WS, Adebola PO. 2017. Nutritional composition of immature pods in selected cowpea [Vigna unguiculata (L.) Walp.] genotypes in South Africa. Aust J Crop Sci. 11:134–141. doi: 10.21475/ajcs.17.11.02.p72
- Ghaly AE, Alkoaik FN. 2010. Extraction of protein from common plant leaves for use as human food. Am J Appl Sci. 7:323–334. doi: 10.3844/ajassp.2010.323.330
- Hair JF, Andrson JR, Tatham RE, Black WC. 1998. Multivariate data analysis. 5th ed. London: Prentice-Hall international, Inc..
- Hasanuzzaman M, Biswas BK, Alam MS, El-Taj HF, Amin MR. 2002. Multivariate analysis in sorghum. Pak J Bio Sci. 5:529–530. doi: 10.3923/pjbs.2002.529.530
- Jamali MK, Kazi TG, Arain MB, Afridi HI, Jalbani N, Sarfraz RA, Baig JA. 2008. A multivariatestudy: variation in uptake of trace and toxic elements by various varieties of sorghum bicolor L. Hazard Material J. 158:644–651. doi: 10.1016/j.jhazmat.2008.02.007
- Jaryum KH, Okoye ZSC, Stoecker B. 2013. Copper content of staple seeds and grains grown in kanam local government area, Nigeria. SpringerPlus. 2:1–5. doi: 10.1186/2193-1801-2-373
- Johnson DE, 1998. Applied multivariate method for data analysis. Pacific Grove (CA): Duxbury press.
- Johnson MD, Larry E. 2008. Copper. Merck manual home health handbook. Merck Sharp and Dohme Corp., a subsidiary of Merck & Co., Inc.
- Liu JG, Liang JS, Li KQ, Zhang ZJ, Yu, BY, Lu XL, Yang JC, Zhu QS. 2003. Correlations between cadmium and mineral nutrients in absorption and accumulation in various genotypes of rice under cadmium stress. Chemosphere. 52:1467–1473. doi: 10.1016/S0045-6535(03)00484-3
- Mamiro PS, Mbwaga AM, Mamiro DP, Mwanri AW, Kinabo JL. 2011. Nutritional qualityand utilization of local and improved cowpea varieties in some regions in Tanzania. Afr J Food Agric Nutr Dev. 11:4490–4506.
- Marconi E, lombardi-boccia G, Carnovoale E, Ng Q. 1990. Nutritional evaluation of wild and cultivated species of cowpea. In: Ng NQ, Monti LM, editors. Cowpea genetic resources. Ibadan: IITA; p. 101–110.
- Mhlaba ZB, Amelework B, Shimelis H, Modi AT, Mashilo J. 2018. Genetic differentiation among selected tepary bean collections revealed by morphological traits and simple sequence repeat markers. Acta Agric Scand B Soil Plant Sci. doi:10.1080/09064710.2018.1450440.
- Mwadzingeni L, Shimelis H, Tesfay S, Tsilo TJ. 2016. Screening of bread wheat genotypes for drought tolerance using phenotypic and proline analyses. Front Plant Sci. 7:1–12. doi: 10.3389/fpls.2016.01276
- Ng’uni D, Geleta M, Hofvander P, Fatih M, Bryngelsson T. 2012. Comparative genetic diversity and nutritional quality variation among some important southern African sorghum accessions [Sorghum bicolor (L.) Moench]. Aust J Crop Sci. 6:56–64.
- Okareh OT, Adeolu AT, Adepoju OT. 2015. Proximate and mineral composition of plantain (Musa paradisiaca) wastes flour; a potential nutrients source in the formulation of animal feeds. Afr J Food Sci Technol. 6:53–57. doi: 10.14303/ajfst.2015.015
- Owolabi AO, Ndidi US, James BD, Amune FA. 2012. Proximate, anti nutrient and mineral composition of five varieties (improved and local) cowpea seed, commonly consume in samaru community, Zaria, Nigeria. Asian J Food Sci Technol. 4:70–72.
- Payne RW, Murray DA, Harding SA, Baird DB, Soutar DM. 2016. Genstat for windows. 18th ed. Hemel Hempstead: VSN International.
- Perrier X, Jacquemoud-collet JP. 2006. DARwin software: dissimilarity analysis and representation for windows. Available from http://www.darwin.cirad.fr/darwin.html.
- Phuke RM, Anuradha K, Radhika K, Jabeen F, Anuradha G, Ramesh T, Hariprasanna K, Mehtre SP, Deshpande SP, Anil G, et al. 2017. Genetic variability, genotype × environment interaction, correlation, and GGE biplot analysis for grain iron and zinc concentration and other agronomic traits in RIL population of sorghum (Sorghum bicolor L. Moench). Front Plant Sci. 8:503. doi:10.3389/fpls.2017.00712.
- Robson AD, Pitman JB. 1983. Interactions between nutrients in higher plants. In: Läuchli A, Bieleski RL, editors. Inorganic plant nutrition. New York: Springer; p. 147–180.
- Rukundo P, Shimelis H, Laing M, Gahakwa D. 2013. Storage root formation, dry matter synthesis, accumulation and genetics in sweet potato. Aust J Crop Sci. 7:2054–2061.
- Samia MA, Hagir BE, Wisal IH, Elfadil EB, Abdullahi HE. 2005. Proximate composition, antinutritional factors and mineral content and availability of selected legumes and cereals grown in Sudan. J Food Technol. 3:511–515.
- Schönfeldt HC, Gibson N. 2009. Healthy eating guide-lines in the South African context. J Food Compos Anal. 22:S68–S73. doi: 10.1016/j.jfca.2009.01.005
- Seetharam N, Clark RB, Marranville JW. 1987. Sorghum genotype differences in uptake and use efficiency of mineral elements. In: Gabelman WH, Loughman BC, editors. Genetic aspects of plant mineral nutrition. Dordrecht/Boston/Lancaster: University of Wisconsin, Madison, Martinus Nijhoff publisher; p. 437–443.
- Shegro A, Shargie NG, Van biljon A, Labuschagne MT. 2012. Diversity in starch, protein and mineral composition of sorghum landrace accessions from Ethiopia. J Crop Sci Biotech. 15:275–280. doi: 10.1007/s12892-012-0008-z
- Singh BB. 2014. Cowpea: the food legume of the 21st century. Crop Science Society of America, Inc., 5585 Guilford Road, Madison, WI 53711-5801 USA.
- Singh BB, Chambliss OL, Sharma B. 1997. Recent advances in cowpea breeding. In: Singh BB, Mohan DR, Dashiell KE, Jackai LEN, editors. Advances in cowpea research. Tsukuba, Ibaraki: International Institute of Tropical Agriculture, Ibadan, Nigeria and Japan International research center for Agricultural Sciences; p. 30–49.
- Souza E, Sorrells ME. 1991. Relationships among 70 North American oat germplasms: I. Cluster analysis using quantitative characters. Crop Sci. 31:599–605. doi: 10.2135/cropsci1991.0011183X003100030010x
- Stanley AB. 1995. Soil nutrient bioavailability. New York: Purdue University; p. 72–180.
- Tan JC, Burns DL, Jones HR. 2006. Severe ataxia, myelopathy, and peripheral neuropathy due to acquired copper deficiency in a patient with history of gastrectomy. J Parenter Enteral Nutr. 30:446–450. doi: 10.1177/0148607106030005446
- Tchiagam JBN, Bell JM, Nassourou AM, Njintang NY, Youmbi E. 2011. Genetic analysis of seed proteins contents in cowpea (Vigna unguiculata L. Walp.). Afri J Biotechnol. 10:3077–3086. doi: 10.5897/AJB10.2469
- Timko MP, Ehlers JD, Roberts PA. 2007. Cowpea. In: Kole C, editor. Genome mapping and molecular breeding in plants, pulses, sugar and tuber crops. Vol. 3. Berlin Heidelberg: Springer Verlag; p. 49–68.
- Timko MP, Singh BB. 2008. Cowpea, a multifunctional legume. In: Moore PH, Ming R, editors. Genomics of tropical crop plants. New York (NY): Springer Science Business Media, LLC; p. 227–258.
- Vandemarka GJ, Grusakb MA, McGee RJ. 2018. Mineral concentrations of chickpea and lentil cultivars and breeding lines grown in the U.S. Pacific Northwest. The Crop J. 2018:253–262. doi: 10.1016/j.cj.2017.12.003
- Vasconcelos IM, Maia FMM, Farias DF, Campello CC, Carvalho AFO, de Azevedo Moreira R, Abreu De Oliveira JT. 2010. Protein fractions, amino acid composition and antinutritional constituents of high-yielding cowpea cultivars. J Food Compos Anal. 23:54–60. doi: 10.1016/j.jfca.2009.05.008
- Walker WM, Miller JE, HAssett JJ. 1977. Effect of lead and cadmium upon the calcium, magnesium, potassium, and phosphorus concentration in young corn plants. Soil Sci. 124:145–151. doi: 10.1097/00010694-197709000-00004
- Ye X, Avendano S, Dekkers JCM, Lamont SJ. 2006. Association of twelve immune-related genes with performance of three broiler lines in two different hygiene environments. Poult Sci. 85:1555–1569. doi: 10.1093/ps/85.7.1165