ABSTRACT
Nanomaterials and particularly silver nanoparticles (AgNPs) have increased great interest in many fields of science. The element may affect plant growth and development but so far research studies have been scarce and their results disparate. Tulip is one of the most important ornamental plants and its production technology is constantly being improved. The aim of this study was to investigate the effects of AgNPs on growth and yield attributes of tulip cv. ‘Pink Impression’. Before planting, the tulip ‘Pink Impression’ bulbs were soaked in the following concentrations of AgNPs: 25, 50, 100 and 150 mg L-1. Control bulbs were soaked in deionized water. The plants were forced in a greenhouse for two growing seasons. The tulips treated with 100 mg L−1 AgNPs flowered earlier, had longer cut-flower stem, larger petals, greater stem diameter and cut-flower fresh weight. Moreover, at this concentration of AgNPs, they showed increased leaf greenness index (SPAD), stomatal conductance, root fresh weight, root length and produced daughter bulbs of the greatest weight. The study outcomes indicate that AgNPs may be used as plant growth promoters in horticulture.
Introduction
Bulbous plants are an important segment of ornamental plant production, the annual value of which is estimated to be around one billion dollars (Benschop et al. Citation2010). Tulip (Tulipa gesneriana L., Liliaceae) is the third most popular cut flower after roses and chrysanthemums. In 2017, Dutch flower exchanges sold over 1894 million cut tulip flowers for a total of 281 million EUR (Royal FloraHolland Citation2018). High market requirements constantly drive improvements in tulip cut flowers quality (Sochacki and Treder Citation2017) and methods shortening the production cycle under covers (Ramzan et al. Citation2014; Amiri et al. Citation2018).
Recently, numerous research studies have focused on nanotechnology and its achievements in many areas of human activity (Singh Citation2017). Silver nanoparticles (AgNPs) belong to the most common nanomaterials obtained via physical, chemical and biological processes, often with the use of various plant species (Zuverza-Mena et al. Citation2017; Rai et al. Citation2018). A limited number of studies conducted so far on AgNPs in agricultural and horticultural settings demonstrated their effects on seed germination, plant growth and development, flowering, yield and physiological processes (Yin et al. Citation2012; Yasur and Rani Citation2013; Byczyńska Citation2017; Zakharova et al. Citation2017). AgNPs promoted seed germination in Pennisetum glaucum (Parveen and Rao Citation2015), stimulated growth and increased the number of inflorescences in Carthamus tinctorius (Zari et al. Citation2015), and in combination with indoleacetic acid and 6-benzyladenine they increased the number and length of adventitious roots in Hibiscus rosa sinensis (Thangavelu et al. Citation2018). In Trigonella foenum-graecum, the application of AgNPs stimulated the growth of seedlings by enhancing leaf number, root lenght, shoot lenght and wet weight of plants (Jasmin et al. Citation2017). Contrary to that, AgNPs inhibited germination in Brassica nigra (Amooaghaie et al. Citation2015), seedlings growth in Lolium multiflorum (Yin et al. Citation2011), Phaseolus radiatus and Sorgum bicolor (Lee et al. Citation2012). The results are inconclusive and identified AgNPs as both growth stimulators and inhibitors.
To our knowledge, this study is the first one demonstrating biostimulating properties of AgNPs in forcing of ornamental bulbous crop plant. Here, we evaluated the effects of bulb soaking in nano-silver solutions of different concentrations on plant growth, flowering, selected physiological parameters and bulb yield of ‘Pink Impression’ tulips.
Material and methods
The experiment carried out over two growing seasons (2014–2015 and 2015–2016) involved prepared tulip bulbs cv. ‘Pink Impression’ of the Darwin hybrid group, imported from the Netherlands (Mantel, Holland). Their mean circumference was 12.2 cm and mean weight 19.1 g. Directly prior to planting the bulbs were soaked for 60 min in AgNPs (Sigma-Aldrich, Spain) solutions (0, 25, 50, 100 and 150 mg L−1) obtained by dissolving AgNPs in deionized water. Each variant consisted of four repetitions, 20 bulbs per independent repetition. The bulbs were planted individually on 31 October 2014 and 22 October 2015 into plastic pots 12 cm in diameter and 0.7 L capacity filled equally (v:v) with sand and peat substrate TS1 (Kronen, Cerkwica, Poland) containing: N-NO3 – 182 mg L−1, P – 131 mg L−1, K – 402 mg L−1, Ca – 1646 mg L−1, Mg – 172 mg L−1 and Cl – 18 mg L−1. The peat substrate had an initial of pH 6.4 and electrical conductivity of 0.54 mS cm−1. The pots were placed in a room intended for bulb cooling. The tulips were forced using a standard method (De Hertogh Citation1996). After 15 weeks of cooling, the pots were transferred into a greenhouse and kept under natural photoperiod at 18 ± 2°C (day) and 16 ± 2°C (night).
We counted the number of days from the beginning of greenhouse forcing to the beginning of flowering that was determined as a fully colored flower bud. At this stage, we randomly selected five plants from each replicate and determined their leaf greenness index (SPAD) with an optical apparatus Chlorophyll Meter SPAD-502 (Minolta, Osaka, Japan) and stomatal conductance with an SC1 porometer (Dekagon Devices, Pullman, WA, USA). The measurements involved three fully developed leaves of each plant and four readings per leaf. The measured parameters included cut-flower length, stem diameter (middle section), petal length, fresh cut-flower weight, fresh weight of roots and root length measured from the basal plate to the end of the longest root. To assess post-harvest longevity in days, cut flowers were placed in individual glass cylinders with a capacity of 0.25 L filled with deionized water. Flower observation was conducted daily in a room with the following controlled conditions: 20 ± 2°C, relative humidity 65 ± 5%, quantum radiation intensity 30 µmol m−2 s−1 applied in a cycle of 10 h of light and 14 h of darkness. Wilting and drying of perianth petals were assumed as a loss of the ornamental value. Three weeks after the end of flowering, we also assessed fresh weight and number of daughter bulbs per plant.
Results from each growing season were analysed with ANOVA for univariate experiments in a complete randomisation arrangement. Calculations were performed using the STATISTICA 13.3 package (StatSoft, Cracov, Poland). Comparison of means and determination of homogeneous groups were based on Tukey’s test for α = 0.05.
Results
Experimental results for both seasons showed that bulb soaking in AgNPs stimulated the growth attributes of tulip (). The use of AgNPs accelerated flowering by on average 4.2 days, depending on concentration and season (). In the first season, the first to bloom were the plants grown from the bulbs soaked in 50 and 100 mg L−1 AgNPs, and in the second season those soaked in 50 mg L−1. All concentrations of AgNPs increased the length of cut flowers, and 100 mg L−1 was the most effective. At this concentration, flowers were by 22.4% longer than the control in the first season and by 28.2% in the second season. Additionally, AgNPs significantly affected the stem diameter that was the highest in the plants grown from the bulbs soaked in 100 mg L−1 AgNPs. Compared to the control, AgNPs treatment increased petal length during both seasons, irrespective of the concentration. The plants obtained from the bulbs treated with 100 mg L−1 AgNPs had the highest fresh weight of cut flowers. At that concentration, the flower fresh weight was 16.9% greater than in the control in the first season and by 24.6% in the second season. Our observations showed no effect of bulb soaking in AgNPs on the post-harvest longevity of cut flowers that usually lasted for six days in both the seasons ().
Figure 1. Visible effects of silver nanoparticles (AgNPs) on tulip growth attributes. Left to right: 0 (control), 25, 50, 100 and 150 mg L−1 AgNPs.
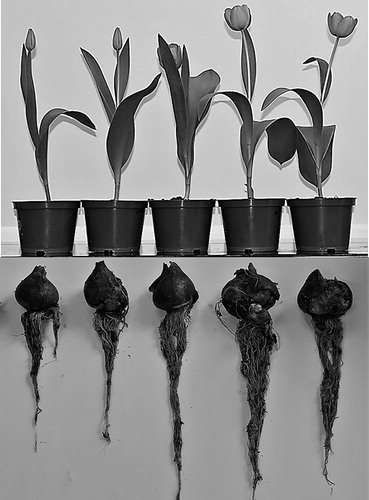
Table 1. Impacts of silver nanoparticles’ (AgNPs) various concentrations on flowering of tulip in two seasons.
To assess physiological changes evoked by AgNPs treatment, we determined the leaf greenness index that correlates with chlorophyll content and stomatal conductance, which is one of the basic parameters of gas exchange ( and ). The leaf greenness index exceeded that of the control in the plants exposed to 100 mg L−1 AgNPs (both seasons) and 150 mg L−1 (the first season). The stomatal conductance was significantly higher in the plants treated with 50 and 100 mg L−1 in both the seasons.
Figure 2. Impacts of silver nanoparticles’ (AgNPs) various concentrations on the leaf greenness index of tulip in seasons 2014–2015 (A) and 2015–2016 (B). Vertical bars indicate the mean ± standard deviation (n = 4). A different letter above each bar indicates a significant difference between treatments at P ≤ 0.05.
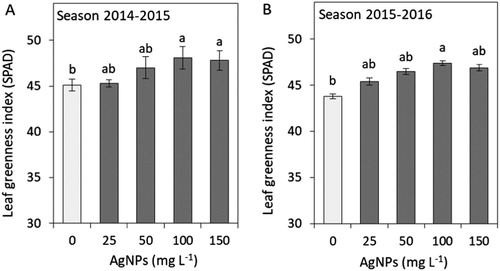
Figure 3. Impacts of silver nanoparticles’ (AgNPs) various concentrations on stomatal conductance of tulip in seasons 2014–2015 (A) and 2015–2016 (B). Vertical bars indicate the mean ± standard deviation (n = 4). A different letter above each bar indicates a significant difference between treatments at P ≤ 0.05.
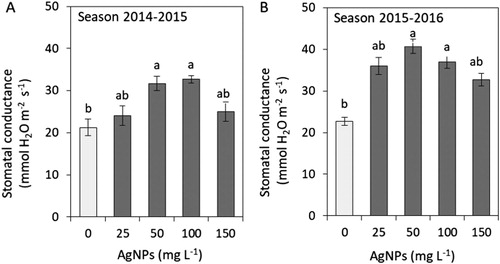
Root system size affects plant growth, which is why we determined the root fresh weight and the length of adventitious roots. In both seasons, the plants grown from the bulbs soaked in 100 mg L−1 AgNPs produced roots of higher fresh weight (). The roots were the longest in the plants treated with 50 and 100 mg L−1 AgNPs, irrespective of the season ().
Figure 4. Impacts of silver nanoparticles’ (AgNPs) various concentrations on the root fresh weight of tulip in seasons 2014–2015 (A) and 2015–2016 (B). Vertical bars indicate the mean ± standard deviation (n = 4). A different letter above each bar indicates a significant difference between treatments at P ≤ 0.05.
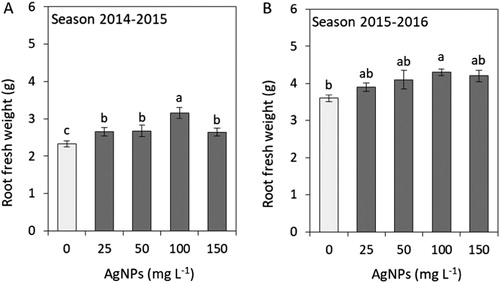
Figure 5. Impacts of silver nanoparticles’ (AgNPs) various concentrations on root length of tulip in seasons 2014–2015 (A) and 2015–2016 (B). Vertical bars indicate the mean ± standard deviation (n = 4). A different letter above each bar indicates a significant difference between treatments at P ≤ 0.05.
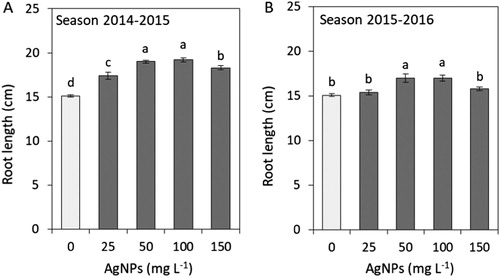
The number and weight of daughter bulbs are important metrics of tulip yield. In both seasons, AgNPs treatment enhanced the fresh weight of daughter bulbs and the effect was the strongest for AgNPs at 100 mg L−1. In the first season, a higher number of daughter bulbs were formed on the plants treated with 50 mg L−1 AgNPs, and in the second with 100 mg L−1 AgNPs ().
Table 2. Impacts of silver nanoparticles’ (AgNPs) various concentrations on bulbs yield of tulip in two seasons.
Discussion
The commercial value of cut tulip flowers depends on early flowering, stem length and diameter, and flower size (De Hertogh Citation1996). Our study showed considerable shortening of the greenhouse forcing period and effective growth stimulation in the plants exposed to AgNPs, particularly at 100 mg L−1 (). The cut flowers obtained from the bulbs treated with 100 mg L−1 AgNPs were longer, had thicker stems, longer petals and higher fresh weights than controls. Our results match the findings of other authors. Pallavi et al. (Citation2016) found that Vigna sinensis plants sprayed with 50 mg L−1 AgNPs produced longer and heavier shoots. Nejatzadeh-Barondozi et al. (Citation2014) noticed a stimulating effect of 60 ppm AgNPs on growth and biomass of Ocimum basilicum. Positive effect of AgNPs on plant growth occurred also in Cucumis sativus (Shams et al., Citation2013), Chrysanthemum morifolium (Tung et al. Citation2018) and Phaseolus vulgaris (Das et al. Citation2018). The effect of AgNPs on growth and development of plants may be attributed to the fact that nanoparticles may enhance metabolic processes that lead to plant growth stimulation or inhibition in general (Feregrino et al. Citation2018). According to Krishnaraj et al. (Citation2012) silver ions may interact with the proteins, starch synthesising machinery and carbohydrate translocation in the plant. Das et al. (Citation2018) propose that nano-silver intensifies plant growth by improving the accumulation of essential macronutrients uptake by roots. It is important to mention that AgNPs may stimulate plant growth by regulating gene expression. For example, AgNPs induced Arabidopsis gene expression related to cell division, metabolism and hormone signalling pathways (Syu et al. Citation2014). The mechanism of the AgNPs’ action in plants is not sufficiently recognised and requires further studies.
Leaf greenness evaluated by SPAD is an important quality criterion in the assessment of ornamental crops (Zawadzińska and Salachna Citation2018). A high SPAD value may indicate increased leaf content of chlorophyll (Dunn et al. Citation2018). In our study, the most vividly green leaves indicated the high quality of tulips grown from the bulbs soaked in 100 and 150 mg L−1 AgNPs (). Moreover, the leaves of tulips treated with 50 and 100 mg L−1 AgNPs had higher stomatal conductance (), which together with increased SPAD suggest enhanced efficiency of photosynthesis. Literature reports confirm these assumptions. For example, AgNPs increased the chlorophyll content in the leaves of P. vulgaris (Das et al. Citation2018), Brassica juncea (Sharma et al. Citation2012) and Solanum tuberosum (Homaee and Ehsanpour Citation2015), and improved net productivity of photosynthesis in Solanum lycopersicum (Zakharowa et al. Citation2017).
In our study soaking tulip bulbs in 100 mg L−1 AgNPs significantly increased both fresh weight and length of the adventitious roots ( and ). Other species also showed differences in root length depending on the AgNPs’ concentration. Seedlings of S. lycopersicum produced markedly longer shoots when grown from seeds soaked in 1.5 mg L−1 AgNPs (Almutairi Citation2016). Soaking seeds in AgNPs positively affected root length in B. juncea (Sharma et al. Citation2012) and Pisum sativum (Barabanov et al. Citation2018). In the first plant AgNPs concentration was below 200 ppm, and in the second AgNPs solution was stabilised with quaternary ammonium salt at 0.005% and 0.001%. Stimulation of root system growth by AgNPs may improve water and nutrient uptake, as reported for P. vulgaris grown on a substrate containing biological concentrations of AgNPs reaching 25–50 mg kg−1 (Das et al. Citation2018).
Some tulip species and cultivars achieve poor bulb propagation rate (Podwyszyńska et al. Citation2015), which is why new solutions increasing bulb yield are constantly being looked for. AgNPs used in our study allowed us to obtain a higher number of daughter bulbs that had also higher fresh weight than those produced by the control. Improved quality and quantity parameters of tulip yield may be due to the stimulating effects of AgNPs on the growth of roots and above ground organs. The potential application of AgNPs in the propagation of other ornamental geophytes is worth investigating.
In conclusion, our study demonstrated that soaking tulip bulbs in 50 and 100 mg L−1 AgNPs shortens the production cycle and improves the quality of cut flowers. Moreover, silver in the form of AgNPs enhanced bulb yield and stimulated root system development. These findings can be applied in forcing tulips in future. However, considering the poorly known impact of AgNPs on the environment, further detailed studies are necessary.
Disclosure statement
No potential conflict of interest was reported by the authors.
Notes on contributors
Andżelika Byczyńska is a PhD Student in the West Pomeranian University of Technology in Szczecin, Poland. Her studies mainly focused on the use of nano-silver in ornamental geophytes.
Agnieszka Zawadzińska has obtained a Ph.D. degree in the subject of horticulture. Presently, she is an academic staff in the Department of Horticulture, the West Pomeranian University of Technology in Szczecin, Poland. His study mainly focused on alternative growing media, salinity and plant growth regulators in ornamentals crops.
Piotr Salachna is a lecturer of horticulture at the West Pomeranian University of Technology in Szczecin, Poland. He was born in Debica and earned his MS (2001) and PhD (2006) from the University of Agriculture in Szczecin. His main areas of expertise include flower bulbs, with special interest Eucomis and Ornithogalum species. He has specialized on the use of natural polysaccharides as biostimulators.
ORCID
Agnieszka Zawadzińska http://orcid.org/0000-0002-0882-9919
Piotr Salachna http://orcid.org/0000-0003-0403-8519
Additional information
Funding
References
- Almutairi ZM. 2016. Influence of silver nano-particles on the salt resistance of tomato (Solanum lycopersicum) during germination. Int J Agric Biol. 18(2):449–457.
- Amiri A, Kafi M, Kalate-Jari S, Matinizadeh M, Karaj I. 2018. Tulip response to different light sources. J Anim Plant Sci. 28(2):539–545.
- Amooaghaie R, Tabatabaei F, Ahadi AM. 2015. Role of hematin and sodium nitroprusside in regulating Brassica nigra seed germination under nanosilver and silver nitrate stresses. Ecotoxicol Environ Saf. 113:259–270.
- Barabanov PV, Gerasimov AV, Blinov AV, Kravtsov AA, Kravtsov VA. 2018. Influence of nanosilver on the efficiency of Pisum sativum crops germination. Ecotoxicol Environ Saf. 147:715–719.
- Benschop M, Kamenetsky R, Nard ML, Okubo H, De Hertogh A. 2010. The global flower bulb industry: production, utilisation, research. Hortic Rev. 36(1):1–115.
- Byczyńska A. 2017. Nano-silver as a potential biostimulant for plant – a review. World Sci News. 86(3):180–192.
- Das P, Barua S, Sarkar S, Karak N, Bhattacharyya P, Raza N, Kim KH, Bhattacharya SS. 2018. Plant extract–mediated green silver nanoparticles: efficacy as soil conditioner and plant growth promoter. J Hazard Mater. 346:62–72.
- De Hertogh AA. 1996. Holland bulb forcer's guide. Hillegom, Netherlands: International Flower Bulb Centre and Dutch Bulb Exporters Association.
- Dunn BL, Singh H, Goad C. 2018. Relationship between chlorophyll meter readings and nitrogen in poinsettia leaves. J Plant Nutr. 41(12):1566–1575.
- Feregrino-Perez AA, Magaña-López E, Guzmán C, Esquivel K. 2018. A general overview of the benefits and possible negative effects of the nanotechnology in horticulture. Sci Hortic. 238:126–137.
- Homaee MB, Ehsanpour AA. 2015. Physiological and biochemical responses of potato (Solanum tuberosum) to silver nanoparticles and silver nitrate treatments under in vitro conditions. Indian J Plant Physiol. 20(4):353–359.
- Jasim B, Thomas R, Mathew J, Radhakrishnan EK. 2017. Plant growth and diosgenin enhancement effect of silver nanoparticles in Fenugreek (Trigonella foenum-graecum L.). Saudi Pharm J. 25(3):443–447.
- Krishnaraj C, Jagan EG, Ramachandran R, Abirami SM, Mohan N, Kalaichelvan PT. 2012. Effect of biologically synthesized silver nanoparticles on Bacopa monnieri (Linn.) Wettst. plant growth metabolism. Process Biochem. 47(4):651–658.
- Lee WM, Kwak JI, An YJ. 2012. Effect of silver nanoparticles in crop plants Phaseolus radiatus and Sorghum bicolor: media effect on phytotoxicity. Chemosphere. 86(5):491–499.
- Nejatzadeh-Barandozi F, Darvishzadeh F, Aminkhani A. 2014. Effect of nano silver and silver nitrate on seed yield of (Ocimum basilicum L.). Org Med Chem Lett. 4(1):11.
- Pallavi MC, Srivastava R, Arora S, Sharma AK. 2016. Impact assessment of silver nanoparticles on plant growth and soil bacterial diversity. 3 Biotech. 6(2):254.
- Parveen A, Rao S. 2015. Effect of nanosilver on seed germination and seedling growth in Pennisetum glaucum. J Clust Sci. 26:693–701.
- Podwyszyńska M, Kosson R, Treder J. 2015. Polyamines and methyl jasmonate in bulb formation of in vitro propagated tulips. Plant Cell Tiss Organ Cult. 123(3):591–605.
- Rai PK, Kumar V, Lee S, Raza N, Kim KH, Ok YS, Tsang DC. 2018. Nanoparticle-plant interaction: implications in energy, environment, and agriculture. Environ Int. 119:1–19.
- Ramzan F, Younis A, Riaz A, Ali S, Siddique MI, Lim KB. 2014. Pre-planting exogenous application of gibberellic acid influences sprouting, vegetative growth, flowering, and subsequent bulb characteristics of ‘Ad-Rem’ tulip. Hortic Environ Biote. 55(6):479–488.
- Royal FloraHolland. 2018. Royal FloraHolland Annual Report 2017.https://annualreport.royalfloraholland.com/?_ga=2.239128835.1893662484.1533650048-1004016660.1533650048#/feiten-en-cijfers/import-export?_k=52jegh [17 August 2018].
- Shams G, Ranjbar M, Amiri A. 2013. Effect of silver nanoparticles on concentration of silver heavy element and growth indexes in cucumber (Cucumis sativus L. negeen). J Nanopart Res. 15(5):1630.
- Sharma P, Bhatt D, Zaidi MGH, Saradhi PP, Khanna PK, Arora S. 2012. Silver nanoparticle-mediated enhancement in growth and antioxidant status of Brassica juncea. Appl Biochem Biotechnol. 167(8):2225–2233.
- Singh NA. 2017. Nanotechnology innovations, industrial applications and patents. Environ Chem Lett. 15(2):185–191.
- Sochacki D, Treder J. 2017. A survey of viruses’ occurrence in Polish and imported tulip bulbs. Acta Sci Pol-Hortoru. 16(1):105–112.
- Syu YY, Hung JH, Chen JC, Chuang HW. 2014. Impacts of size and shape of silver nanoparticles on Arabidopsis plant growth and gene expression. Plant Physiol Biochem. 83:57–64.
- Thangavelu RM, Gunasekaran D, Jesse MI, Su MR, Sundarajan D, Krishnan K. 2018. Nanobiotechnology approach using plant rooting hormone synthesized silver nanoparticle as “nanobullets” for the dynamic applications in horticulture – an in vitro and ex vitro study. Arab J Chem. 11(1):48–61.
- Tung HT, Nam NB, Huy NP, Luan VQ, Hien VT, Phuong TTB, Le DT, Loc NH, Nhut DT. 2018. A system for large scale production of chrysanthemum using microponics with the supplement of silver nanoparticles under light-emitting diodes. Sci Hortic. 232:153–161.
- Yasur J, Rani PU. 2013. Environmental effects of nanosilver: impact on castor seed germination, seedling growth, and plant physiology. Environ Sci Pollut Res. 20(12):8636–8648.
- Yin L, Cheng Y, Espinasse B, Colman BP, Auffan M, Wiesner M, Bernhardt ES. 2011. More than the ions: the effects of silver nanoparticles on Lolium multiflorum. Environ Sci Technol. 45:2360–2367.
- Yin L, Colman BP, McGill BM, Wright JP, Bernhardt ES. 2012. Effects of silver nanoparticle exposure on germination and early growth of eleven wetland plants. PLoS One. 7(10):e47674.
- Zakharova OV, Gusev AA, Zherebin PM, Skripnikova EV, Skripnikova MK, Ryzhikh VE, Lisichkin GV, Shapoval OA, Bukovskii ME, Krutyakov YA. 2017. Sodium tallow amphopolycarboxyglycinate-stabilized silver nanoparticles suppress early and late blight of Solanum lycopersicum and stimulate the growth of tomato plants. BioNanoScience. 7(4):692–702.
- Zari H, Babak P, Asad R. 2015. The effect of priming with nano-sliver on agronomic traits of safflower cultivars. J Essent Oil Bear Pl. 18(5):1148–1156.
- Zawadzińska A, Salachna P. 2018. Effects of some peat-alternative substrate on the growth and chemical composition of pelargonium. Acta Hortic. 1201:641–647.
- Zuverza-Mena N, Martínez-Fernández D, Du W, Hernandez-Viezcas JA, Bonilla-Bird N, López-Moreno ML, Peralta-Videa JR, Gardea-Torresdey JL. 2017. Exposure of engineered nanomaterials to plants: insights into the physiological and biochemical responses-A review. Plant Physiol Biochem. 110:236–264.