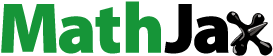
ABSTRACT
Paddy rice (Oryza sativa L.) is easy to suffer from iron (Fe) deficiency in drip-irrigated (DI) calcareous soils, especially at the seedling stage. Low soil temperature and the soil nitrogen (N) is dominated by nitrate () is probably the reason of Fe chlorosis in this stage. The objective of this experiment was to elucidate the causes of Fe deficiency induced by low soil temperature and the mechanism of ammonium (
) alleviate Fe deficiency of DI rice. Applying different N forms (
or
) to two rice cultivars (cv. ‘T43’ and ‘T04’, Fe-efficient and Fe-inefficient genotype, respectively) under different soil temperatures to study the growth and Fe uptake of DI rice. The results showed that low soil temperature reduced the root biomass and root activity of rice significantly, which in turn, resulted in low leaf SPAD value and Fe uptake of rice. The rice fed with
had improved root activity and bigger root surface area, higher rhizosphere soil DTPA-Fe concentration and more Fe uptake than fed with
under low soil temperature. The results indicated
alleviates Fe deficiency of DI rice by improving the availability of rhizosphere soil Fe, increasing the root surface area and root activity. Application of
to DI rice is a promise agronomic measure to alleviate Fe deficiency.
Introduction
The technology of planting paddy rice (Oryza sativa L.) by drip irrigation has a significant water saving potential while achieving higher yields (Guo and Chen Citation2012). Drip-irrigated (DI) rice normally keeps soil water content between soil water holding capacity 80%–100%, but no obvious water layer on the soil surface. It is considered as revolutionary water saving technology for rice to cope with the worldwide water crisis, especially in arid areas (He, Ma, et al. Citation2013; He, Yang, et al. Citation2016). In practice, it was found that DI rice was prone to iron (Fe) deficiency chlorosis at the seedling stage on calcareous soils, after soil temperature rose, rice chlorosis has gradually disappeared.
Ammonium () and nitrate (
) nitrogen (N) are the two main forms of inorganic N in the soil. The soil N in the flooded soil is mainly
and that the N in the upland is mainly
because of the nitrification process (Qian et al. Citation2004). Rice is a typical
-prefer crop with a high
assimilation capacity (Zhu et al. Citation2009), and
is easier be uptake by root than
in low temperature (Clarkson and Warner Citation1979; Bassirirad Citation2000). Numerous studies have shown that plants absorb
to acidify rhizosphere, while the absorption of
can alkalise the rhizosphere (Thomson et al. Citation1993; Mattson et al. Citation2009). So DI rice uptake
have a high rhizosphere pH (Chen et al. Citation2018), this, in turn, may reduce Fe, Zn, and P uptake due to these elements are less effective in high pH environment (Gao et al. Citation2006; Kobayashi et al. Citation2010; Fan et al. Citation2012). In addition, N forms also influence Fe transportation of plant. The general view is that
will increase the pH of the plant apoplasts, thereby reducing the Fe reductase activity of plasma membrane, resulting in limited Fe absorption in plants (Nikolic and Römheld Citation2003; Kosegarten et al. Citation2004). However,
can reduce apoplast pH and hence boost Fe uptake and transportation inside the plant (Zou et al. Citation2002; Zou and Zhang Citation2003). In a previous study, we found that ammonium sulfate can increase the activity of roots and alleviate the Fe deficiency of DI rice (Zhang et al. Citation2015). Furthermore, Zhu et al. (Citation2018) indicated that
improved the Fe reutilisation of rice roots and thus alleviate Fe deficiency in rice. Considering, the rice seedlings in flooding soil seldom suffer from Fe deficiency in the same condition, we then suspect Fe-deficiency-chlorosis of the DI rice is related to N forms. In addition, although the use of increased N input and foliar Fe-containing solution application can improve Fe nutrient of rice, it was not a sustainable and economic agronomic practice for rice production (Zhang, Wu, et al. Citation2008; Zhang, Wang, et al. Citation2009).
The small amount but high-frequency irrigation and fertilisation technique by drip irrigation may allow the aerobic soil to form an dominated environment. Thus, in this study, we try to use this strategy to correct Fe deficiency problems in DI rice. Firstly, the hypothesis we proposed are: (i) low soil temperature restricting root growth and Fe uptake of DI rice, and (ii) the
nutrition can alleviate Fe deficiency of DI rice induced by the low soil temperature compared to the
, because of improved rhizosphere Fe availability by root H+ emission, better root growth and root Fe uptake ability. To evaluate these physiological responses in DI rice, a pot experiment was conducted under simulated drip irrigation in low soil temperature condition. The effects of low soil temperature on the growth and Fe uptake of rice and the effects of
on Fe uptake of rice were examined through supply different N forms to different Fe-deficiency tolerant rice varieties in two different soil temperature conditions.
Materials and methods
Soil properties
This study was conducted at the Shihezi University Agricultural Experiment Station, Shihezi City, Xinjiang Uyghur Autonomous Region, China (44◦ 18’ N, 86◦ 02’ E) in 2017. The soil was collected from the 0 to 20 cm depth of arable land at the experimental station. The soil at the experiment, which has a sandy loam texture, is a Calcareous Fluvisol according to the FAO-UNESCO classification system (FAO Citation1998). Some physical and chemical properties of the soil are as follows: pH (1: 2.5 soil: water), 7.48; EC (1: 5 soil: water), 0.20 dS m−1; organic matter, 9.50 g kg−1; total N, 0.88 g kg−1; available P, 14.0 mg kg−1; and available K, 224 mg kg−1; DTPA-Fe, 6.89 mg kg−1; DTPA-Zn, 0.77 mg kg−1; DTPA-Mn, 5.62 mg kg−1, and CaCO3, 150.8 g kg−1. Soil organic matter (Walkley-Black wet oxidation method), total N (Kjeldhal method), available P (Resin-P), and rapidly available K (NH4OAC-K) were measured as described by Page (Citation1982). Fresh soil samples were extracted with DTPA-TEA (0.005 M diethylene triamine pentaacetic acid + 0.1 M triethanolamine + 0.01 M CaCl2, adjusted to pH 7.3) and DTPA-Fe, DTPA-Zn and DTPA-Mn were determined by atomic absorption spectrophotometer (Hitachi Z-2000, Japan) as described by Lindsay and Norvell (Citation1978). Air-dried soil samples were measured soil CaCO3 with the gas evolution method as described by Horton and Newsom (Citation1953).
Experimental design
The experiment consisted of a 2 × 2 × 2 factorial design. There were two cultivars of rice [Fe-efficient genotype rice (O. sativa L. cv. T43) and Fe-inefficient genotype rice (O. sativa L. cv. T04) according to Li et al. (Citation2017), abbreviated Fe-efficient and Fe-inefficient, respectively], two N forms [ ((NH4)2SO4 + 2.0% dicyandiamide (DCD)) and
(Ca(NO3)2 + 2.0% dicyandiamide (DCD)), abbreviated NAM and NNI, respectively], and two soil temperatures in the rice root zone (low soil temperature of 18 ± 2°C and optimal soil temperature of 24 ± 2°C according to the experience of local farmers in planting DI rice and other researchers’ report (Shimono et al. Citation2004; Arai-Sanoh et al. Citation2010), abbreviated T18 and T24, respectively). The eight treatments were arranged in a completely randomised block design with four replicates for each treatment, resulting in a total of 32 pots.
The soil was air-dried, passed through a 4-mm sieve, mixed with P (triple superphosphate, 0.15 g P2O5 kg−1 soil) and K (potassium sulfate, 0.2 g K2O kg−1 soil) fertiliser, and then packed into plastic pots (diameter 20 cm height 20 cm). Each pot contained 4.0 kg soil. In order to obtained a complete rice root system, each pot contains three root bags (diameter 6 cm height 10 cm) in advance. After preparing the pots, 1.2 L water was applied to saturate the soil until the water leaked sufficiently. The soil was allowed to dry naturally for 2 d and then the rice was sown.
Rice (O. sativa L. cv. Fe-efficient genotype and Fe-inefficient genotype) seeds were pre-germinated by soaking in water for 24 h and sown in root bags (six seeds per root bag). The stand was thinned to three plants per hill after emergence. Water was added to the soil using an intravenous drip apparatus. The water content in the pot was maintained at >80% of the soil water holding capacity but never flooded. The soil water content in all the treatments was monitored by weighing the pots. Irrigation was applied as needed, usually once every 2 d.
Soil temperature and different N treatments were initiated when the rice seedlings grew to four leaves (35 days after emergence of seed). The different N forms fertiliser (0.2 g N kg−1 soil) application was added to the rice seedlings once by irrigation. Then, each N form treatments were placed in two different temperature-controlled water tank on average. Soil temperature was controlled by circulating water in each tank. The temperature control system was designed as described by Bai et al. (Citation2017). After 15 days of the N application, the contents of and
content in the soil were 272.6 mg kg−1 (
), 80.8 mg kg−1 (
) in the NAM treatments and 71.2 mg kg−1 (
), 241.2 mg kg−1 (
) in the NNI treatments. After the 40th day of the soil temperature treatment, the rice root bag was taken out from the soil in combination with the rice roots. Two hills of rice were sampled for the rhizosphere soil pH and DTPA-Fe concentration, rice biomass and rice Fe absorption. Another hill of rice was immersed in water to obtain the complete roots system to measure the root surface area and root activity of rice. The entire growth stage of the rice was conducted outdoors.
Sampling and measurement methods
Leaf SPAD value of rice
A SPAD-502 chlorophyll metre (Konica Minolta SPAD-502, Japan) was used to measure the greenness of the 10 highest leaves in each pot on the sampling date.
Rhizosphere soil pH and rhizosphere soil DTPA-Fe
The soil adhering to the roots was harvested as the rhizosphere soil. The rhizosphere soil pH was measured with a combined electrode pH metre (Hanna HI 98185, Italy) after shaking a 1.0: 2.5 soil: water suspension for 3 min. The rhizosphere soil DTPA-extractable Fe was extracted with DTPA-TEA (0.005 M diethylene triamine pentaacetic acid + 0.1 M triethanolamine + 0.01 M CaCl2, adjusted to pH 7.3) after shaking a 2: 1 extractant: soil suspension at 25°C for 2 h (Lindsay and Norvell Citation1978). The filtrate was then immediately analysed with an atomic absorption spectrophotometer (Hitachi Z-2000, Japan).
Root ferric chelate reductase activity (FC-R)
Roots collected from root bag were rinsed with tap water to remove soil particles in its surface, then rinsed 3 times with deionised water. Then, the roots were immersed in a saturated CaSO4 solution for 5 min and washed with distilled water 3 times to remove contaminating Fe present on the root surface. The roots were transferred to 20 mL of a nutrient solution containing 100 µmol L−1 Fe(III)-EDTA and 400 µmol L−1 2,2-bipyridyl, 10 mM MES buffer (pH 5.5). After 2 h, an aliquot of the assay solution was removed, and the FC-R was determined by measuring the concentration of a Fe2+-bipyridyl complex formed at A523 in a spectrophotometer (Shimadzu UV-2201) (Li et al. Citation2000).
Biomass and Fe uptake analysis
The plant samples, which were used to collect the rhizosphere soil, were divided into the shoot and root. Before the Fe uptake analysis, the roots were carefully washed with water followed by a 0.5 mM CaSO4 solution to remove the soil particles. The shoots were washed with water followed by a 0.1 M HCl solution. The root and shoot samples were then washed several times with deionised water. The shoot and root samples were then dried in an oven at 80°C and weighed before analysis the shoot and root Fe concentrations.
Oven-dried samples were ground with a stainless-steel mill to pass through a 0.5-mm sieve. The samples were digested in HNO3-HClO4 (v: v, 2: 8) at 120°C until the solution became transparent (Zhu et al. Citation2018). The filtrate was analysed with an atomic absorption spectrophotometer (Hitachi Z-2000, Japan).
Root activity and root surface area of rice
One-hill plants were removed from the plastic pots with the root bag. The roots were carefully washed with water to remove the soil particles. The total absorption area and active adsorption area of fresh root samples were determined by methylene blue dyeing to characterise the root activity parameters as previously described by Yang et al. (Citation2004). After that, the rice roots were immersed in water, and the fine roots were separated gently with a toothpick to avoid injury before imaging. The roots were scanned using a flatbed image scanner (Epson Expression/STD 1600 scanner). The images were analysed using WinRhizo commercial software (Regent Instruments, 2001) to determine the root surface area of the rice plants.
Statistical analysis
All statistical analyses were conducted with SPSS 17.0 statistical software using three-way ANOVA followed by Duncan’s multiple range test at P < 0.05. All the data were analysed from each treatment with four replicates.
Results
Effects of N forms on the rhizosphere soil pH and rhizosphere soil DTPA-Fe in different soil temperatures
The N forms and the soil temperature significantly affected the rhizosphere soil pH ((A)). Under the identical soil temperature (T18 or T24), the rhizosphere soil pH in the NAM was significantly lower than that in the NNI. For Fe-efficient genotype, compared with the NNI, the rhizosphere soil pH in the NAM was reduced by 0.21 units under the T24 treatments, while under the T18 treatments, the rhizosphere soil pH in the NAM was only reduced by 0.07 units. For Fe-inefficient genotype, compared with the NNI, the rhizosphere soil pH in the NAM was reduced by 0.22 units under the T24 treatments, while under the T18, the rhizosphere soil pH in the NAM was only reduced by 0.09 units ((A)).
Figure 1. Effect of nitrogen forms (N) on rhizosphere soil pH (A) and rhizosphere soil DTPA-Fe (B) of rice cultivars (G) in different soil temperatures (T). Error bars represent SE (n = 4). Columns within each cultivar the same letter are not significantly different at 5% according to Duncan’s multiple range test. Abbreviations: Fe-efficient and Fe-inefficient, Fe-efficient genotype rice (O. sativa L. cv. T43) and Fe-inefficient genotype rice (O. sativa L. cv. T04). NAM and NNI, ammonium nitrogen was (NH4)2SO4 + 2.0% DCD and nitrate nitrogen was Ca(NO3)2 + 2.0% DCD, respectively. T24 and T18, soil optimum temperature was 24 ± 2°C and soil low temperature was 18 ± 2°C, respectively. *** and ns indicate significant differences at p < 0.1% and p ≥ 5%.
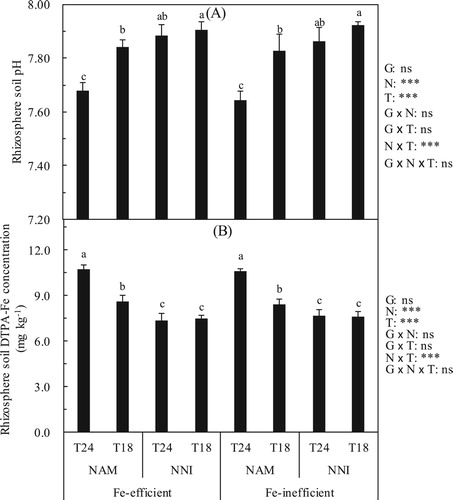
The N forms and the soil temperature significantly affected the rhizosphere soil DTPA-Fe concentration ((B)). Under the identical soil temperature (T24 or T18), the rhizosphere soil DTPA-Fe concentration in the NAM was significantly higher than that in the NNI. The rhizosphere soil DTPA-Fe of Fe-efficient genotype under the T18 displayed an increase of 1.11 mg kg−1 in the NAM compared to that in the NNI, however, the rhizosphere soil DTPA-Fe in the NAM increased by 3.36 mg kg−1 under the T24 compared with that in the NNI. The rhizosphere soil DTPA-Fe of Fe-inefficient genotype under the T18 displayed an increase of 0.85 mg kg−1 in the NAM compared to that in the NNI, while, under the T24, the rhizosphere soil DTPA-Fe in the NAM increased by 2.92 mg kg−1 compared with the NNI ((B)).
Overall, showed significant rhizosphere soil acidification and soil Fe activation ability compared with
, this acidification effect and increased of the DTPA-Fe of rhizosphere soil were influenced by soil temperature. Low soil temperature weakens the acidification effect and Fe activation ability in the rhizosphere of rice.
Effects of N forms on the FC-R of rice root and SPAD value of rice leaf in different soil temperatures
The rice genotype, the N forms and the soil temperature significantly affected the FC-R of rice root ((A)). Under the identical N forms (NAM or NNI), the root FC-R in the T18 were significantly higher than those in the T24. Under the identical soil temperature (T24 or T18), the root FC-R in the NNI were significantly higher than those in the NAM. Under the NAM treatments, Fe-efficient genotype displayed an increase of 0.35 units in the FC-R in the T18 compared with the T24, while under the NNI treatments, the root FC-R in the T18 increased by 0.59 units compared with those in the T24. Under the NAM, Fe-inefficient genotype displayed an increase in FC-R in the T18 by 0.57 units compared with the T24, while under the NNI, the root FC-R in the T18 increased by 0.62 units compared with those in the T24 ((A)).
Figure 2. Effect of nitrogen forms (N) on root ferric chelate reductase activity (A) and leaf SPAD value (B) of rice cultivars (G) in different soil temperatures (T). Error bars represent SE (n = 4). Columns within each cultivar the same letter are not significantly different at 5% according to Duncan’s multiple range test. Abbreviations: Fe-efficient and Fe-inefficient, Fe-efficient genotype rice (O. sativa L. cv. T43) and Fe-inefficient genotype rice (O. sativa L. cv. T04). NAM and NNI, ammonium nitrogen was (NH4)2SO4 + 2.0% DCD and nitrate nitrogen was Ca(NO3)2 + 2.0% DCD, respectively. T24 and T18, soil optimum temperature was 24 ± 2°C and soil low temperature was 18 ± 2°C, respectively. *, **, *** and ns indicate significant differences at P < 5%, P < 1%, p < 0.1% and p ≥ 5%.
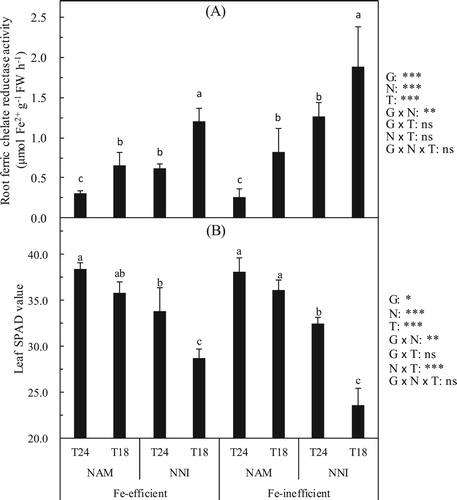
The rice genotype, the N forms and the soil temperature significantly affected the leaf SPAD values of rice ((B)). Under the NAM treatments, the SPAD value of Fe-efficient or Fe-inefficient genotype did not differ significantly between the T24 and the T18. Under the NNI, the SPAD values of Fe-efficient or Fe-inefficient genotype in the T18 were significantly lower than that in the T24. Under the identical soil temperature (T24 or T18), the SPAD values of Fe-efficient or Fe-inefficient genotype in the NAM were significantly higher than those in the NNI. Under the NAM treatments, the leaf SPAD value of Fe-efficient genotype displayed a reduction of 4% in the T18 compared with that in the T24, while under the NNI treatments, the SPAD value decreased by 15% in the T18 compared with that in the T24. Under the NAM treatments, Fe-inefficient genotype displayed a reduction in the leaf SPAD value of 5% in the T18 compared with that in the T24, while under the NNI treatments, the SPAD value decreased by 27% in the T18 compared with that in the T24 ((B)).
In summary, the low soil temperature induced lower SPAD value in leaves of rice compared with the optimal soil temperature. The root Fe deficiency response magnitude caused by the low soil temperature was less in than in
. Correspondingly,
can alleviate the decrease of the leaves SPAD value due to the low soil temperature compared with
, and the magnitude of the SPAD value decreased by the low soil temperature was greater in Fe-inefficient genotype than that in Fe-efficient genotype.
Effects of N forms on rice growth in different soil temperatures
The N forms and the soil temperature significantly affected the rice biomass (). Under the identical N (NAM or NNI), the shoot dry weight, root dry weight and number of tillers were significantly lower in the T18 than in the T24, this is true both in Fe-efficient and Fe-inefficient genotype. A comparison of the rice variety Fe-efficient genotype with the T24 indicated that the shoot dry weight, root dry weight and number of tillers reduction rates in the T18 were 40%, 29% and 33% under the NAM treatments, respectively. However, under the NNI treatments, compared with the T24, the reduction rates of the shoot dry weight, root dry weight and the number of tiller in the T18 were 43%, 36% and 40%, respectively. A comparison of the rice variety Fe-inefficient genotype with the T24 indicated that the shoot dry weight, root dry weight and number of tiller reduction rates in the T18 were 25%, 20% and 43% under the NAM treatments, respectively. However, under the NNI treatments, compared with the T24, the shoot dry weight, root dry weight and number of tiller reduction rates in the T18 were 55%, 51% and 46%, respectively.
Figure 3. Effect of nitrogen forms (N) on shoot dry weight (A), root dry weight (B) and number of tillers (C) of rice cultivars (G) in different soil temperatures (T). Error bars represent SE (n = 4). Columns within each cultivar the same letter are not significantly different at 5% according to Duncan’s multiple range test. Abbreviations: Fe-efficient and Fe-inefficient, Fe-efficient genotype rice (O. sativa L. cv. T43) and Fe-inefficient genotype rice (O. sativa L. cv. T04). NAM and NNI, ammonium nitrogen was (NH4)2SO4 + 2.0% DCD and nitrate nitrogen was Ca(NO3)2 + 2.0% DCD, respectively. T24 and T18, soil optimum temperature was 24 ± 2°C and soil low temperature was 18 ± 2°C, respectively. *, **, *** and ns indicate significant differences at P < 5%, P < 1%, p < 0.1% and p ≥ 5%.
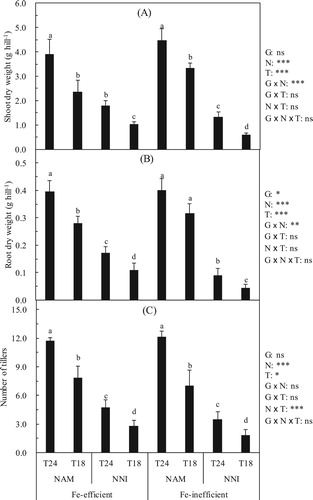
Overall, the low soil temperature significantly inhibited the growth of the rice (e.g. the shoot, root dry weight and number of tillers). can alleviate the inhibitory effect of the low soil temperature on rice growth compared with
. This inhibition of the Fe-inefficient genotype was stronger than that of the Fe-efficient genotype.
Effects of N forms on the root surface area and root activity of rice in different soil temperatures
The N forms and the soil temperature significantly affected the root surface area, root total surface area and active adsorption area of rice root, and the root surface area and active adsorption area were also significantly affected by the rice genotype (). Under the identical N treatments (NAM or NNI), the root surface area, total absorption area and active absorption areas of these two rice varieties were all significantly lower in the T18 than those in the T24. Under the identical soil temperature (T24 or T18), the root surface area, total root absorption area and active absorption area of these two rice varieties were all significantly higher in the NAM than those in the NNI.
Table 1. Effects of different nitrogen forms (N) on root surface area and root activity of rice cultivars (G) in different soil temperatures (T).
In the Fe-efficient genotype, under the NAM treatments, the root surface area, total absorption area and active area of the rice roots decreased by 28%, 22% and 23%, respectively, in the T18 compared with those in the T24, while under the NNI treatments, in the T18 compared with the T24, the root surface area, total absorption area and active area of the rice roots decreased by 50%, 26% and 31%, respectively. In Fe-inefficient genotype, under the NAM treatments, in the T18 compared with the T24, the root surface area, total absorption area and active area of the rice roots decreased by 16%, 26% and 29%, respectively, while under the NNI treatments, in the T18 compared with the T24, the root surface area, total absorption area and active area of the rice roots decreased by 44%, 34% and 52%, respectively ().
In summary, the low soil temperature reduced the rice root surface area and root activity significantly, and this reduction was stronger in Fe-inefficient genotype than in Fe-efficient genotype. can alleviate the reduction in root activity and root surface area of rice under low soil temperature.
Effects of N forms on Fe uptake of rice in different soil temperatures
The N forms and the soil temperature significantly affected the shoot and root Fe concentrations of rice (). Under the NAM treatments, there were no significant differences in the shoot Fe concentrations of these two rice variety between the T24 and the T18, but the Fe concentration in rice roots was significantly higher in the T18 than that in the T24. Under the NNI treatments, both the shoot and the root Fe concentrations of these two rice varieties were all significantly higher in the T18 than that in the T24.
Table 2. Effects of different nitrogen forms (N) on Fe uptake of rice cultivars (G) in different soil temperatures (T).
The N forms and the soil temperature significantly affected the shoot Fe uptake and root Fe uptake of rice (). Under the identical soil temperature (T18 or T24), both the shoot and the root Fe uptake of these two rice cultivars were all significantly higher in the NAM than in the NNI. Under the NAM treatments, the shoot Fe uptake of these two rice cultivars were significantly lower in the T18 than in the T24, but the root Fe uptake of these two rice cultivars were all significantly higher in the T18 than in the T24. Under the NNI treatments, the shoot Fe uptake of Fe-efficient genotype was significantly higher in the T18 than in the T24, and the shoot Fe uptake of Fe-inefficient genotype had no significant difference between the T18 and the T24. There was also no significant difference in the root Fe uptake of these two rice cultivars between the T18 and the T24 under the NNI treatments.
Overall, compared to ,
can increase Fe uptake in the different parts of rice whether or not the rice root was subjected to low soil temperature. In addition, both
and low soil temperature caused Fe to deposition in the roots of rice.
Discussion
In this study, (NAM) shows significant rhizosphere soil acidification and soil Fe activation ability compared with
(NNI), however, the low soil temperature (T18) weaken these effect in rhizosphere of rice (), the reason may because root N uptake ability of rice was significantly reduced due to the reduction of root area and root activity by low root temperature (Feng et al. Citation2011; Nagasuga et al. Citation2011). The previous studies have indicated that a strong root system is an important guarantee for plant nutrient uptake (Li et al. Citation2016). In this study, the root dry weight, root surface area, root total absorption area and active absorption area of rice root were all decreased in the T18 compared to the T24 ((B); ), this in return, restrict Fe uptake of rice. Such as, in these rice varieties with the identical NAM treatments, the Fe uptake of the shoot in the T18 were significantly less than that in the T24. However, (B) and 3 show that under the identical NNI treatment, although the rice had the lowest leaves SPAD values and the strongest growth restriction in the T18, the root Fe concentration, shoot Fe concentrations and Fe uptake were still significantly higher than those in the T24 (). These results parallel those ascribed to the so called ‘chlorosis paradox’ as described by Mengel (Citation1994), whereby severe inhibition of plant growth results in an increased concentration of Fe in chlorotic leaves (Römheld Citation2000; Gruber and Kosegarten Citation2002), because the growth of rice is severely limited by low soil temperature in this study (), and
induce rice to suffer from Fe deficiency which led to the restriction of rice normal growth also (Chen et al. Citation2018). Similar results have been reported by Díaz et al. (Citation2009). In addition, the increase of root FC-R indicates that plants are suffering from Fe deficiency stress (Marschner et al. Citation1986; Poonnachit and Darnell Citation2004), and the result of this study showed that the T18 treatments had lower leaf SPAD values and higher root FC-R compared to the T24 (). Therefore, the low soil temperature restricts growth and decrease Fe absorption ability of rice root, which aggravates Fe absorption barrier of rice.
Rice is a typical -prefer crop with a high
assimilation capacity (Zhu et al. Citation2009), and
is easier uptake by root than
in low temperature (Clarkson and Warner Citation1979; Bassirirad Citation2000). Combined with these two reasons, both the Fe-efficient and Fe-inefficient genotype had the lowest biomass in NNI with the T18 treatment, and biomass declining ratio of rice in the NAM due to low soil temperature was also smaller than that in the NNI can well explanation (). The data showed that in the Fe-efficient genotype with the identical NAM, compared with the T24, the root total absorption area and active absorption area of rice in the T18 decreased by 22% and 23%, while in the identical NNI treatments, which decreased by 26% and 31%. In the Fe-inefficient genotype with the identical
supply, compared with the T24, the root total absorption area and active absorption area of rice in the T18 decreased by 26% and 29%, while in
supply, which decreased by 34% and 52% (). Obviously, no matter in which N forms, the decrease of root activity by low soil temperature in the Fe-inefficient genotype was stronger than that in the Fe-efficient genotype, and compared with
,
can release this decrease effect. This opinion can be confirmed by the shoot Fe uptake of rice. For example, under the identical soil temperature (T18 or T24), both the shoot and the root Fe uptake of these rice cultivars were all significantly higher in the NAM than in the NNI (). In addition, in these rice varieties with the identical NAM treatments, there was no significant difference in the leaf SPAD value under different soil temperature treatments, however, in the NNI treatments, the leaf SPAD values were decreased significantly by the low soil temperature, and this decrease was stronger in the Fe-inefficient genotype than in the Fe-efficient genotype ((B)). Similar results were also reported by Cao et al. (Citation2017), they reported that the total chlorophyll content in rice leaves in
group was significantly higher than that in
group under cold stress. Fe-deficiency sensitive genotypes tend to have higher root FC-R than Fe-deficiency tolerant genotypes under Fe stress (Bityutskii et al. Citation2017), and the increase in FC-R indicates Fe demand in plants in Fe stress (Marschner et al. Citation1986; Poonnachit and Darnell Citation2004). From these results, we concluded that
can ensure a relatively high Fe absorption capacity of rice root to alleviate Fe deficiency of rice in low soil temperature. Our previous research also verified this result from different aspects, such as Zhang et al. (Citation2015) and Zhang et al. (Citation2017) indicated that delayed sowing date and add plant growth regulators can improve the root physiological activity of drip-irrigated rice to alleviate rice seedling chlorosis, respectively.
In conclusion, the results indicated that low soil temperature severely inhibits root growth would aggravate Fe deficiency of DI rice at the seedling stage, however, -supply can alleviate Fe deficiency of DI rice in low soil temperature. The mechanism of
alleviates Fe deficiency of DI rice in low temperature has primarily two aspects: (i) improve the availability of rhizosphere Fe, (ii) ensure that the rice root system has an effective absorptive capacity for Fe nutrients by increasing the root surface area and root activity. Application of
to rice is a promise agronomic measure to alleviate Fe deficiency of DI rice.
Disclosure statement
No potential conflict of interest was reported by the authors.
Notes on contributors
Xinjiang Zhang is a PhD candidate in plant nutrition at Agronomy College, Shihezi University, Xinjiang, China. He published 3 research articles on plant nutrition, mainly focus on Fe deficiency in drip-irrigated rice.
Hui Liu is a PhD candidate in plant nutrition at Life Sciences College, Shihezi University, Xinjiang, China. She published 2 research articles on plant nutrition, mainly focus on nitrogen regulation in rice.
Chaoran Meng is a Postgraduate in plant nutrition at Agronomy College, Shihezi University, Xinjiang, China. He is involved in research on plant nutrition, mainly focus on Fe deficiency in drip-irrigated rice.
Zhiyang Zhang is an Undergraduate in plant nutrition at Agronomy College, Shihezi University, Xinjiang, China. He is involved in research on plant nutrition, mainly focus on Fe deficiency in drip-irrigated rice.
Mengmeng Wang is an Undergraduate in plant nutrition at Agronomy College, Shihezi University, Xinjiang, China. She is involved in research on plant nutrition, mainly focus on Fe deficiency in drip-irrigated rice.
Changzhou Wei has obtained PhD degree in the subject of plant nutrition. Presently he is a professor in Agronomy College, Shihezi University in Xinjiang, China. He has published 8 research papers in international journals, mainly focus on nutrition disorder in drip-irrigated rice and soil nutrition dynamic when soil convert from flooding to aerobic (upland soil). Presently he is involved in research in Fe deficiency mechanism in drip-irrigated rice system.
ORCID
Xinjiang Zhang http://orcid.org/0000-0001-6522-4548
Changzhou Wei http://orcid.org/0000-0003-1677-0678
Additional information
Funding
References
- Arai-Sanoh Y, Ishimaru T, Ohsumi A, Kondo M. 2010. Effects of soil temperature on growth and root function in rice. Plant Prod Sci. 13:235–242. doi: 10.1626/pps.13.235
- Bai RX, Chen L, Zhang XJ, Wei GH, Wei CZ. 2017. Effect of salinity and soil temperature on the growth and physiology of drip-irrigated rice seedlings. Arch Agron Soil Sci. 63:513–524. doi: 10.1080/03650340.2016.1223842
- Bassirirad H. 2000. Kinetics of nutrient uptake by roots: responses to global change. New Phytol. 147:155–169. doi: 10.1046/j.1469-8137.2000.00682.x
- Bityutskii NP, Yakkonen KL, Petrova AI, Shavarda AL. 2017. Interactions between aluminium, iron and silicon in Cucumber sativus L. grown under acidic conditions. J Plant Physiol. 218:100–108. doi: 10.1016/j.jplph.2017.08.003
- Cao XC, Zhong C, Zhu LF, Zhang JH, Hussain S, Wu LH, Jin QY. 2017. Glycine increases cold tolerance in rice via the regulation of N uptake, physiological characteristics, and photosynthesis. Plant Physiol Bioch. 112:251–260. doi: 10.1016/j.plaphy.2017.01.008
- Chen HF, Zhang Q, Cai HM, Zhou W, Xu FS. 2018. H2o2 mediates nitrate-induced iron chlorosis by regulating iron homeostasis in rice. Plant Cell Environ. 41:767–781. doi: 10.1111/pce.13145
- Clarkson DT, Warner AJ. 1979. Relationships between root temperature and the transport of ammonium and nitrate ions by Italian and perennial ryegrass (Lolium multiflorum and Lolium perenne). Plant Physiol. 64:557–561. doi: 10.1104/pp.64.4.557
- Díaz I, Del Campillo MC, Cantos M, Torrent J. 2009. Iron deficiency symptoms in grapevine as affected by the iron oxide and carbonate contents of model substrates. Plant Soil. 322:293–302. doi: 10.1007/s11104-009-9916-1
- Fan XY, Karim MR, Chen XP, Zhang YQ, Gao XP, Zhang FS, Zou CQ. 2012. Growth and iron uptake of lowland and aerobic rice genotypes under flooded and aerobic cultivation. Commun Soil Sci Plant. 43:1811–1822. doi: 10.1080/00103624.2012.684826
- FAO. 1998. World reference base for soil resources. Rome Italy: FAO. 84 World Soil Resources Reports.
- Feng HM, Yan M, Fan XR, Li BZ, Shen QR, Miller AJ, Xu GH. 2011. Spatial expression and regulation of rice high-affinity nitrate transporters by nitrogen and carbon status. J Exp Bot. 62:2319–2332. doi: 10.1093/jxb/erq403
- Gao XP, Zou CQ, Fan XY, Zhang FS, Hoffland E. 2006. From flooded to aerobic conditions in rice cultivation: consequences for zinc uptake. Plant Soil. 280:41–47. doi: 10.1007/s11104-004-7652-0
- Gruber B, Kosegarten H. 2002. Depressed growth of nonchlorotic vine grown in calcareous soil is an iron deficiency symptom prior to leaf chlorosis. J Plant Nutr Soil Sci. 165:111–117. doi: 10.1002/1522-2624(200202)165:1<111::AID-JPLN111>3.0.CO;2-B
- Guo QR, Chen L. 2012. Analysis of advantage and prospects for rice drip irrigation under plastic film cultivation development in China. China Rice. 18:36–39. (in Chinese).
- He HB, Ma FY, Yang R, Chen L, Jia B, Cui J, Fan H, Wang X, Li L. 2013. Rice performance and water use efficiency under plastic mulching with drip irrigation. Plos One. 8:e83103. doi:ARTNe8310310.1371/journal.pone.0083103.
- He HB, Yang R, Wu LQ, Jia B, Ma FY. 2016. The growth characteristics and yield potential of rice (Oryza sativa) under non-flooded irrigation in arid region. Ann Appl Biol. 168:337–356. doi: 10.1111/aab.12267
- Horton J, Newsom D. 1953. A rapid gas evolution method for calcium carbonate equivalent in liming materials. Soil Sci Soc Am J. 17:414–415. doi: 10.2136/sssaj1953.03615995001700040029x
- Kobayashi O, Higuchi K, Miwa E, Tadano T. 2010. Growth injury induced by high pH in rice and tomato. Soil Sci Plant Nutr. 56:407–411. doi: 10.1111/j.1747-0765.2010.00470.x
- Kosegarten H, Hoffmann B, Rroco E, Grolig F, Glüsenkamp KH, Mengel K. 2004. Apoplastic pH and FeIII reduction in young sunflower (Helianthus annuus) roots. Physiol Plantarum. 122:95–106. doi: 10.1111/j.1399-3054.2004.00377.x
- Li CJ, Zhu XP, Zhang FS. 2000. Role of shoot in regulation of iron deficiency responses in cucumber and bean plants. J Plant Nutr. 23:1809–1818. doi: 10.1080/01904160009382144
- Li XX, Zeng RS, Liao H. 2016. Improving crop nutrient efficiency through root architecture modifications. J Integr Plant Biol. 58:193–202. doi: 10.1111/jipb.12434
- Li YY, Bai RX, Zhang XJ, Yang YZ, Huang ZH, Hou JW, Wei CZ. 2017. Evaluating of rice genotypes tolerant to Fe deficiency with drip irrigation under the film mulch. J Plant Nutr Fertilizer. 23:781–788. (in Chinese).
- Lindsay W, Norvell WA. 1978. Development of a DTPA soil test for zinc, iron, manganese, and copper. Soil Sci Soc Am J. 42:421–428. doi: 10.2136/sssaj1978.03615995004200030009x
- Marschner H, Römheld V, Kissel M. 1986. Different strategies in higher plants in mobilization and uptake of iron. J Plant Nutr. 9:695–713. doi: 10.1080/01904168609363475
- Mattson N, Leatherwood R, Peters C. 2009. Nitrogen: All forms are not equal. Cari Peters JR Peters Inc:61.
- Mengel K. 1994. Iron availability in plant tissues-iron chlorosis on calcareous soils. Plant Soil. 165:275–283. doi: 10.1007/BF00008070
- Nagasuga K, Murai-Hatano M, Kuwagata T. 2011. Effects of low root temperature on dry matter production and root water uptake in rice plants. Plant Prod Sci. 14:22–29. doi: 10.1626/pps.14.22
- Nikolic M, Römheld V. 2003. Nitrate does not result in iron inactivation in the apoplast of sunflower leaves. Plant Physiol. 132:1303–1314. doi: 10.1104/pp.102.017889
- Page AL. 1982. Methods of soil analysis. Part 2. chemical and microbiological properties. Madison, WI, USA: ASA, CSSA, SSSA.
- Poonnachit U, Darnell R. 2004. Effect of ammonium and nitrate on ferric chelate reductase and nitrate reductase in Vaccinium species. Ann Bot-London. 93:399–405. doi: 10.1093/aob/mch053
- Qian XQ, Shen QR, Xu GH, Wang JJ, Zhou MY. 2004. Nitrogen form effects on yield and nitrogen uptake of rice crop grown in aerobic soil. J Plant Nutr. 27:1061–1076. doi: 10.1081/PLN-120037536
- Römheld V. 2000. The chlorosis paradox: Fe inactivation as a secondary event in chlorotic leaves of grapevine. J Plant Nutr. 23:1629–1643. doi: 10.1080/01904160009382129
- Shimono H, Hasegawa T, Fujimura S, Iwama K. 2004. Responses of leaf photosynthesis and plant water status in rice to low water temperature at different growth stages. Field Crop Res. 89:71–83. doi: 10.1016/j.fcr.2004.01.025
- Thomson C, Marschner H, Römheld V. 1993. Effect of nitrogen fertilizer form on pH of the bulk soil and rhizosphere, and on the growth, phosphorus, and micronutrient uptake of bean. J Plant Nutr. 16:493–506. doi: 10.1080/01904169309364548
- Yang CM, Yang LZ, Yang YX, Ouyang Z. 2004. Rice root growth and nutrient uptake as influenced by organic manure in continuously and alternately flooded paddy soils. Agr Water Manage. 70:67–81. doi: 10.1016/j.agwat.2004.05.003
- Zhang J, Wang MY, Wu LH. 2009. Can foliar iron-containing solutions be a potential strategy to enrich iron concentration of rice grains (Oryza sativa L.)? Acta Agr Scand Section B-SP. 59:389–394.
- Zhang J, Wu LH, Wang MY. 2008. Iron and zinc biofortification in polished rice and accumulation in rice plant (Oryza sativa L.) as affected by nitrogen fertilization. Acta Agr Scand Section B-SP. 58:267–272.
- Zhang J, Zhang SJ, Chen L, Wei CZ. 2017. Alleviation of seeding chlorosis by plant growth regulators in drip-irrigated rice. Acta Agr Scand Section B-SP. 67:184–190.
- Zhang SJ, Zhang XJ, Wang J, Huang QN, Bai RX, Wei CZ. 2015. Applying soil acidification agents and adjusting sowing date to deal with rice chlorosis in seedling stage caused by iron deficiency under drip irrigation with plastic film mulching. China J Rice Sci. 29:519–527. (in Chinese).
- Zhu CQ, Zhang JH, Zhu LF, Abliz B, Zhong C, Bai ZG, Hu WJ, Hussain S, Allen BJ, Cao XC, et al. 2018. NH4+ facilitates iron reutilization in the cell walls of rice (Oryza sativa) roots under iron-deficiency conditions. Environ Exp Bot. 151:21–31. doi: 10.1016/j.envexpbot.2018.03.018
- Zhu YY, Di TJ, Xu GH, Chen X, Zeng HQ, Yan F, Shen QR. 2009. Adaptation of plasma membrane H+ATPase of rice roots to low pH as related to ammonium nutrition. Plant Cell Environ. 32:1428–1440. doi: 10.1111/j.1365-3040.2009.02009.x
- Zou CQ, Zhang FS. 2003. Ammonium improves iron nutrition by decreasing leaf apoplastic pH of sunflower plants (Helianthus annuus L. cv. Frankasol). Chinese Sci Bull. 48:2216–2221. doi: 10.1007/BF03182855
- Zou CQ, Zhang FS, Goldbach H. 2002. Iron fractions in the apoplast of intact root tips of Zea mays L. seedlings affected by nitrogen form. Chinese Sci Bull. 47:727–731. doi: 10.1360/02tb9164