ABSTRACT
Cassia alata (Caesalpiniaceae) is a large perennial shrub native to tropical America. It is an important medicinal and ornamental plant and has been reported to possess several pharmacological properties. However, phytotoxic substances from C. alata have not yet been documented in the literature. Therefore, this study seeks to evaluate the phytotoxic potential of C. alata leaves and to identify inhibitory substances for the purpose of eco-friendly weed management. The effect of aqueous methanol extracts of C. alata on the seedling growth of alfalfa, cress, lettuce, rapeseed, barnyard grass, foxtail fescue, Italian ryegrass, and timothy was examined. The level of inhibition of the extracts corresponded to concentration. Several chromatographic steps were performed to separate the extracts, and through spectroscopic analysis, two active substances were isolated and characterised as rutin and syringone. These two active substances significantly inhibited the seedling growth of cress and foxtail fescue. The range of I50 values (required concentration for 50% growth inhibition) of rutin and syringone for the seedling growth of cress and foxtail fescue were 129.5–417.8 and 160.1–466.5 µM, respectively. These results indicate that the two identified active phytotoxic substances from C. alata may be responsible for its growth inhibitory properties.
Introduction
Weeds are an impediment to crop production, and farmers mostly rely on synthetic herbicides as the most effective approach to control them (Varshney et al. Citation2012). However, the extensive and uneven application of synthetic herbicides has caused severe environmental pollution, human health problems, and destruction of biodiversity (Pimentel and Burgess Citation2014; Mahmood et al. Citation2016). Therefore, alternative methods of weed control based on natural products is now a pressing issue. Natural herbicides from allelochemicals or phytotoxic compounds have more benefits over synthetic compounds as they have less or non-toxic effect and short half-life, considered as safe weed management way for environment (Duke et al. Citation2002; Nebo et al. Citation2014). Therefore, natural product based herbicide can be applicable as a component of sustainable weed management to ensure satisfaction of human needs for present and future generations. Consequently, phytotoxicity may be an important consideration in replacing synthetic herbicides to control weeds (Ullah et al. Citation2015). It has been suggested that plant materials possessing phytotoxic potential can be used in agriculture as natural herbicides (Kato-Noguchi et al. Citation2016; Benvenuti et al. Citation2017). Many reports have documented the successful use of plants having phytotoxic potential (their extracts/residues or isolated substances) to control weeds in crop fields in place of using synthetic herbicides (Xuan et al. Citation2001; Hong et al. Citation2004; Mushtaq et al. Citation2010). Moreover, phytotoxic substances have no residual toxic effects on the environment (Amb and Ahluwalia Citation2016). In this regard, there has been much attention on finding potential phytotoxic substances from medicinal plants (Kuddus et al. Citation2011; Islam et al. Citation2014).
Cassia alata (Caesalpiniaceae) is a large perennial shrub native to tropical America but is now found in a wide range of habitats in the tropics, such as in Bangladesh, Indonesia, Africa, Philippines, and Jamaica (Awal et al. Citation2004; Ajose Citation2007). This plant grows to about 3–4 m tall with leaves 50–80 cm long and is commonly known as candle bush because the inflorescence of this plant resembles a yellow candle. The fruit of this plant is straight and pod-like, up to 25 cm long, and the seeds are spread by animals or water (Hirt et al. Citation2008). Cassia alata has been reported to have several pharmacological properties (Villaseñor et al. Citation2002; Awal et al. Citation2004; Levy and Lewis Citation2011). The plant has been effectively used to treat ringworm infections, and thus, it is also known as ringworm shrub (Owoyale et al. Citation2005). Numerous studies have reported that C. alata is broadly used for treating skin diseases, gastrointestinal conditions, allergic reactions, and internal and external infections (Ghani Citation1998; Hennebelle et al. Citation2009) and is well known in Africa and India as a natural remedy for diabetes (Abo et al. Citation2008; Khan and Yadava Citation2010). Plants in the Cassia genus generally produce a variety of secondary metabolites including flavonoids, anthraquinones, triterpenes, steroids, alkaloids, pyrrolizine and pyrrolizidine alkaloids, and tannins (Hemlata and Kalidhar Citation1993; Idu et al. Citation2007), some of which possess biological and pharmacological activities such as antioxidative, antimicrobial, anti-inflammatory, antitumor, and cytotoxic (Panichayupakaranant and Kaewsuwan Citation2004; Fernand et al. Citation2008). Cassia species have gained attention as important sources of compounds used for medicinal treatment because they possess several secondary metabolites. Moreover, thirteen known anthraquinones and flavonoids have been isolated from C. alata after phytochemical investigation, of which aloe emodin, emodin, diosmetin, and kaempferol were found in the leaves of C. alata (Promgool et al. Citation2014). However, to the best of our knowledge, to date there has been no report on the phytotoxic substances in C. alata leaves except for a few preliminary phytotoxicity bioassay studies (Osuagwu and Anyanwu Citation2014; Vivekanandan and Ajeesh Citation2018). Therefore, we conducted the present study to investigate the phytotoxic potential of and to isolate and identify the phytotoxic substances in C. alata leaves.
Materials and methods
Collection of plant materials
The leaves of Cassia alata were collected from the Botanical Garden of Bangladesh Agricultural University (BAU) and Kewatkhali village in Sadar Upazilla, Mymensingh, Bangladesh during August 2016. After collection, the leaves were washed under running water to remove dust and other debris, dried in the shade, and then ground with a grinder into a fine powder. The powder was kept in a plastic bag at 2°C until extraction.
Test plant species
In this study, four dicotyledonous plants [alfalfa (Medicago sativa L.), cress (Lepidium sativum L.), lettuce (Lactuca sativa L.), and rapeseed (Brassica napus L.)] and four monocotyledonous plants [barnyard grass (Echinochloa crus-galli (L.) P. Beauv), foxtail fescue (Vulpia myuros (L.) C.C. Gmel), Italian ryegrass (Lolium multiflorum Lam.), and timothy (Phleum pratense L.)] were chosen as test plant species for bioassay. Alfalfa, cress, lettuce, and rapeseed were selected due to their known seedling growth pattern, and barnyard grass, foxtail fescue, Italian ryegrass, and timothy were used for their abundance in crop fields as common weeds.
Preparation of extracts
The powder of the C. alata leaves (1.3 kg) was extracted for 48 h using 8 L of 70% (v/v) aqueous methanol. The extract was then filtered through one layer of filter paper (No. 2; Toyo Roshi Ltd., Japan). The residue was re-extracted with the same volume of cold methanol for 24 h and filtered. The two filtrates were combined and evaporated to complete dryness using a rotary evaporator at 40°C.
Growth bioassay
For the biological assay, the dried extract of the C. alata leaves was dissolved in 300 mL cold methanol to prepare six assay concentrations (1, 3, 10, 30, 100, and 300 mg dry weight (DW) equivalent extract/mL). Aliquots of leaf extract at the assay concentrations were added to sheets of filter paper (No. 2) in 28 mm Petri dishes and dried in a draft chamber to remove the methanol. The filter paper in each Petri dish was then moistened with 0.6 mL of 0.05% (v/v) aqueous solution of Tween 20 (polyoxyethylene sorbitan monolaurate; Nacalai, Kyoto, Japan) as a surfactant. Seeds (n = 10) of alfalfa, cress, lettuce, and rapeseed and pre-germinated seeds (n = 10) of barnyard grass, foxtail fescue, Italian ryegrass, and timothy (incubated in darkness for 68, 48, 46, and 72 h, respectively at 25°C) were then arranged on the filter paper in the Petri dishes. Control treatments of the seeds and pre-germinated seeds were set with only an aqueous solution of Tween 20 without the plant extracts. The seedling growth of the tested species was measured after 48 h incubation in the darkness at 25°C. The percentage length of the seedlings was calculated by reference to the length of the seedlings in the control treatment (). The I50 values of each test species were calculated using a logistic regression equation of the concentration–response curves. In this study, the bioassay for each test plant was conducted with three replications and repeated twice (10 seeds or pre-germinated seeds/replication).
Figure 1. The growth inhibitory effect of Cassia alata leaf extracts on the shoot and root growth of alfalfa, cress, lettuce, rapeseed, barnyard grass, foxtail fescue, Italian ryegrass, and timothy. All the test plants were treated with concentrations of 1, 3, 10, 30, 100, and 300 mg dry weight equivalent extract of C. alata/mL. Mean ± SE from two independent experiments with three replications (10 seedlings replication−1) for each experiment (n=60) are shown. Significant differences between treatments and control are denoted by asterisks: *p < 0.05, **p < 0.01, ***p < 0.001 (one-way ANOVA, post hoc by LSD test).
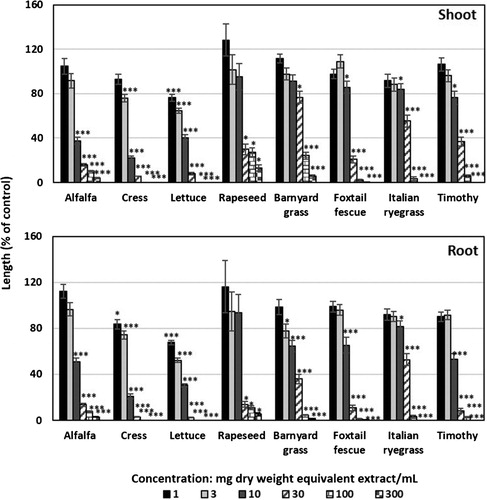
Extract partitions
The C. alata leaf extracts were dried using a rotary evaporator at 40°C to prepare an aqueous residue, then the pH was adjusted to 7.0 with 1 M phosphate buffer. After that, the extract was partitioned nine times against an equal volume of ethyl acetate and separated into ethyl acetate and aqueous fractions. The cress bioassay was done to determine the biological activity of these two fractions. The most active ethyl acetate fraction of the extracts was taken for further isolation.
Isolation and purification of the active substances
The ethyl acetate fraction was evaporated to dryness after overnight soaking with anhydrous Na2SO4. The residue of the ethyl acetate fraction was loaded into a column of silica gel (60 g, silica gel 60, 70–230 mesh; Nacalai Tesque, Kyoto, Japan) and then eluted with n-hexane containing an increasing amount of ethyl acetate (10% increase step−1 (v/v), 150 mL step−1) and methanol (300 mL). The growth inhibitory activities of the obtained nine fractions were determined using the cress bioassay as described above. The cress bioassay results showed significant inhibitory activities in the fractions 5–9 but most activity was found in the fraction 6 (). The most active fraction obtained using the silica gel column was evaporated and purified on a column of Sephadex LH-20 (GE Healthcare Bio-Science AB, SE-75184, Uppsala, Sweden) and eluted with 20, 40, 50, 60, 70, and 80% (v/v) of aqueous methanol (150 mL step−1) and methanol (300 mL). The higher inhibitory activities on cress seedling were found in the fractions 2 and 3 (). Both active fractions were then separately evaporated to dryness, dissolved in 20% (v/v) aqueous methanol, and loaded on to a reverse-phase C18 cartridge (YMC Co. Ltd., Kyoto, Japan). For both fractions, the cartridge was eluted separately with 20, 30, 40, 50, 60, 80% (v/v) aqueous methanol and methanol. The most active fractions were eluted with 40% aqueous methanol in both cases which were then purified using reverse-phase HPLC (5 µm, 4.6 × 250 mm I.D., Inertsil® ODS-3; GL Science Inc., Tokyo, Japan). The column was eluted at a flow rate of 0.8 mL min−1 with 30% (v/v) aqueous methanol (for substance 1) and 20% (v/v) aqueous methanol (for substance 2). The active substances, detected at a wavelength of 220 nm and oven temperature of 40°C, were eluted in the peak fractions between 55–100 min retention time as a yellow substance (substance 1) and 62–80 min retention time as a colourless substance (substance 2). The substances were characterised using HRESIMS, 1H-NMR, and specific rotation.
Figure 2. Effects of the fractions obtained from silica gel column of the extract of Cassia alata on the seedling growth of cress. Cress was exposed to the concentration equivalent to the extracts obtained from 600 mg dry weight of Cassia alata/mL. Values given are means ± SE from two independent experiments. Significant differences between treatments and control are indicated by asterisks: *p < 0.05, **p < 0.01, ***p < 0.001 (one-way ANOVA, post hoc by LSD test).
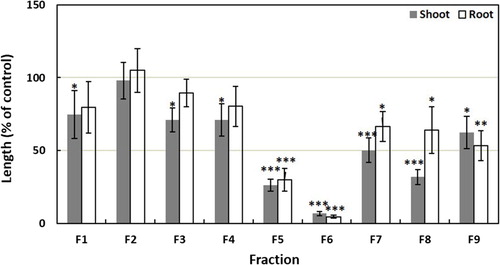
Figure 3. Effects of the fractions obtained from Sephadex LH-20 column (from fraction 6 in silica gel) of the extract of Cassia alata on the seedling growth of cress. Cress was exposed to the concentration equivalent to the extracts obtained from 900 mg dry weight of Cassia alata/mL. Values given are means ± SE from two independent experiments. Significant differences between treatments and control are indicated by asterisks: *p < 0.05, **p < 0.01, ***p < 0.001 (one-way ANOVA, post hoc by LSD test).
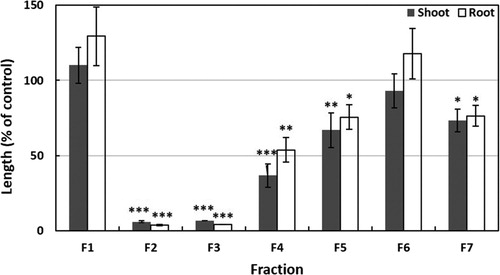
Bioassay of the isolated substances
The isolated substances were dissolved in methanol to prepare final assay concentrations of 1, 3, 10, 30, 100, 300, and 1000 µM, added to a sheet of filter paper (No. 2, 28 mm; Toyo) in 28 mm Petri dishes, and the solvent evaporated in a draft chamber. The biological activity of the isolated substances was determined using cress and foxtail fescue, as mentioned before. The I50 values of these substances on the test species were determined as described earlier.
Statistical analysis
The bioassay experiments were conducted in a completely randomised design with three replications and repeated twice. The generated data were analysed (analysis of variance and post-hoc with an LSD test at a 5% level of probability) using SPSS version 16.0. The required concentrations for 50% inhibition (I50 value) of the test species were analysed using GraphPad Prism ®Ver. 6.0 (GraphPad Software, Inc., La Jolla, California, USA). Significant differences of I50 values for shoot and root growth of cress and foxtail fescue between rutin and syringone was analysed by Independent-samples T test at p ≤ 0.05.
Results and discussion
Phytotoxic activity of C. alata leaf extracts on test plant species
The aqueous methanol extracts of the C. alata leaves inhibited the growth of the test plant species (alfalfa, cress, lettuce, rapeseed, barnyard grass, foxtail fescue, Italian ryegrass, and timothy) at different concentrations (). The concentration obtained from 300 mg dry weight equivalent extract of C. alata/mL completely inhibited (100%) the shoot and root growth of cress, lettuce, Italian ryegrass, and timothy. The shoot and root growth of alfalfa, rapeseed, barnyard grass, and foxtail fescue were inhibited by 4.1, 13.0, 5.6, and 0.5% of the growth of the control shoots and 2.9, 5.3, 1.5, and 0.4% of the growth of the control roots, when exposed to the same concentration of the C. alata extracts. Other concentrations also showed different levels of growth inhibition of the test plants (). These results denote that the C. alata leaf extracts inhibited the growth of both the monocotyledonous and dicotyledonous plant species, and the inhibitory activity increased with the increase in extract concentration. The I50 values for the shoots and roots of all test species were 5.3–55.3 and 3.3–32.0 mg dry weight equivalent extract/mL, respectively (). The I50 values of the cress shoots and lettuce roots show they were the most sensitive to the extracts, and barnyard grass shoots and Italian ryegrass roots were the least sensitive. These results imply that growth inhibition by the C. alata leaf extracts was different for different species. Similar findings on plant extracts were also reported by Das and Kato-Noguchi (Citation2018), Boonmee et al. (Citation2018), and Kato-Noguchi et al. (Citation2016). The concentration-dependent and species-specific inhibitory pattern of the C. alata leaf extracts suggest that this plant may possess phytotoxic substances.
Table 1. The concentrations required for 50% growth inhibition (I50 values) of the aqueous methanol extracts from Cassia alata leaves for the shoot and root growth of the test plant species.
Identification of phytotoxic substances
After partitioning, both the ethyl acetate and aqueous fractions of the plant extracts showed concentration-dependent growth inhibitory activities on the cress seedlings, but greater inhibitory activity was observed with the ethyl acetate fraction. Therefore, the ethyl acetate fraction was further purified through several chromatographic steps (silica gel column, Sephadex LH-20 column, and reverse-phase C18 cartridges), and at every purification step, a cress bioassay was done to determine the biological activity. Two growth inhibitory substances were isolated after final purification using reverse-phase HPLC. The substances were identified based on NMR and high-resolution spectral data and comparison with previous reported data.
Substance 1 has a molecular formula of C27H30O16, determined using HRESIMS at m/z 633.1440 [M + Na] + (calcd for C27H30O16Na, 633.1432, Δ = +0.8mmu). The 1H NMR (400 MHz, CD3OD) spectrum of this substance showed δH 7.67 (d, J = 2.2 Hz, 1 H, H-2′), 7.63 (dd, J = 8.7, 2.1 Hz, 1 H, H-6′), 6.88 (d, J = 8.7 Hz, 1 H, H-5′), 6.40 (d, J = 2.1 Hz, 1 H, H-8),6.21 (d, J = 2.1 Hz, 1 H, H-6), 5.11 (d, J = 7.9 Hz, 1 H, H-1″), 4.52 (d, J = 1.3 Hz, 1 H, H-1″′), 3.80 (dd, J = 11.1, 1.3 Hz, 1 H, H-6″b), 3.63 (dd, J = 3.3, 1.3 Hz, 1 H, H-2″′), 3.54 (dd, J = 9.6, 3.3 Hz, 1 H, H-3″′), 3.47 (dd, J = 8.9, 7.9 Hz, 1 H, H-2″), 3.45 (m, 1 H, H-5″′), 3.41 (m, 1 H, H-3″), 3.39 (m, 1 H, H-6″a), 3.28 (m, 1 H, H-4″′), 3.26 (m, 1 H, H-4″), 1.12 (d, J = 6.5 Hz, 3 H, H-6″′), H-5″. The specific rotation of this substance was [α]D25 = +13 (c = 0.10, EtOH). From the comparison of these data with those reported in the literature (Krewson and Naghski Citation1952; Forino et al. Citation2016), the substance was identified as rutin ().
Rutin (3′, 4′, 5, 7-tetrahydroxyflavone-3β-D-rutinoside; quercetin-3-O-rutinoside) is a bioflavonol, found in buckwheat, tomato, apple, apricot, rhubarb, tea, celery, spinach, passion flower, brussels sprouts, and lemon (Appleton Citation2010; Ganeshpurkar and Saluja Citation2017). Rutin is also one of the primary flavonols identified in ‘clingstone’ peaches (Chang et al. Citation2000). The plant Ruta graveolens also contains rutin, from which the name rutin is derived (Ganeshpurkar and Saluja Citation2017). Although rutin has been found in a wide variety of plants, this report is the first on rutin in C. alata.
The molecular formula of substance 2 was determined as C10H12O4 using HRESIMS at m/z 197.0799 [M + H] + (calcd for C10H13O4, 197.0814, Δ= −1.5 mmu). The 1H NMR (400 MHZ, CDCl3) spectrum of substance 2 showed δH 7.25 (s, 2 H, H2, 6), 5.94 (s, 1 H, 4-OH), 3.96 (s, 6 H, H8, 10), 2.58 (s, 3 H, H8). By comparing these data with previously reported data (Bao et al. Citation2009), substance 2 was identified as syringone ().
Syringone (4′-hydroxy-3′, 5′-dimethoxyacetophenone) is a natural phenolic compound, identified in sea grass, tobacco, and a wide variety of other plants (Agostini et al. Citation1998; Baker et al. Citation2005). This substance secretes from the wound sites of dicotyledons (Raja et al. Citation2010). However, this is the first report on isolating syringone from C. alata leaves.
Biological activity of rutin and syringone
The biological activities of rutin and syringone were measured by conducting bioassays with cress and foxtail fescue. The seedling growth of cress and foxtail fescue was significantly inhibited by rutin at concentrations greater than 3 µM (). In contrast, syringone significantly inhibited the seedling growth of cress and foxtail fescue at concentrations greater than 100 and 10 µM, respectively (). Rutin inhibited the shoot and root growth of cress by 13.5 and 4.4% of control at the concentration of 1000 µM, respectively, whereas the shoot and root growth of foxtail fescue were inhibited by 34.1 and 26.9% of control, respectively. At the same concentration, syringone inhibited the shoot and root growth of cress by 26.6 and 23.5% of control, respectively, and inhibited the shoot and root growth of foxtail fescue by 39.1 and 30.1% of control, respectively. In both cases, inhibition depended on the concentration of rutin and syringone ( and ).
Figure 5. Effect of rutin on the seedling growth of cress and foxtail fescue. Mean ± SE from three independent experiments with 10 seedlings for each treatment are shown. Significant differences between treatments and control are denoted by asterisks: *p < 0.05, **p < 0.01, ***p < 0.001 (one-way ANOVA, post hoc by LSD test).
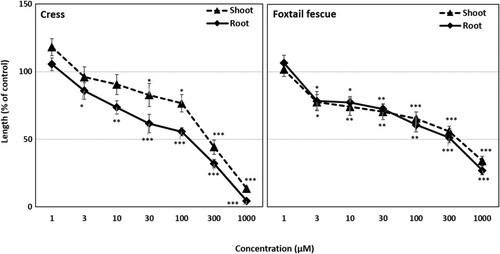
Figure 6. Effect of syringone on the seedling growth of cress and foxtail fescue. Mean ± SE from two independent experiments with 10 seedlings for each treatment are shown. Significant differences between treatments and control are denoted by asterisks: *p < 0.05, **p < 0.01, ***p < 0.001 (one-way ANOVA, post hoc by LSD test).
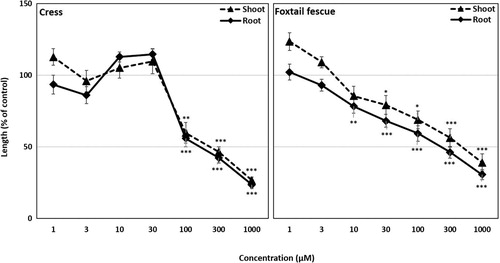
The I50 values of rutin for the shoots and roots of cress were 246.7 and 129.5 µM, respectively, and those of foxtail fescue were 417.8 and 320.4 µM, respectively (). On the other hand, the I50 values of syringone for the shoot and root growth of cress were 223.8 and 160.1 µM, respectively, whereas those of foxtail fescue were 466.5 and 219.6 µM, respectively ().
Table 2. I50 values (concentration required for 50% growth inhibition) of rutin and syringone for the shoot and root growth of cress and foxtail fescue.
From the results of the I50 values, it is clear that both substances possess a wide range of activity on different plant species, and the phytotoxic activity was different among the substances though there is no significance differences of the effectiveness of rutin and syringone (). The structural differences in the substances may be responsible for the different levels of phytotoxic activity (Islam et al. Citation2017). The structure of rutin consists of an aglycone quercetin and a disaccharide rutinose bound to quercetin at position 3, ring C (). The four -OH groups in rutin and rutinose bonded at position 3 on ring C may strongly influence the activity of rutin (Macikova et al. Citation2012; Seruga and Tomac Citation2017). Jovanovic et al. (Citation1996) also investigated the importance of the -OH groups on ring C on the potentiality of flavonoids. Guerrero et al. (Citation2012) reported that catechol group in the B-ring, double bond between C2 and C3 at C-ring and ketone group in C4 at C-ring, are responsible for inhibitory effect of flavonoids. Syringone is a member of the class of acetophenones in which 1-phenylethanone is replaced by a hydroxy group at position 4 and methoxy groups at positions 3 and 5 (). The presence and distribution of the hydroxy group on the aromatic ring may be responsible for the activity of syringone (Prihantini et al. Citation2015). Besides, a six-membered pyranose ring, equatorial or axial hydroxyls at C-4, and a permissible equatorial substitution at the C-5 carbon, are the common structural features, may be induce the activity of syringone (Ankenbauer and Nester Citation1990).
A number of pharmacological activities of rutin such as antioxidant, cytoprotective, anticarcinogenic, neuroprotective, vasoprotective, and cardioprotective have been demonstrated (Janbaz et al. Citation2002; Mellou et al. Citation2006; Trumbeckaite et al. Citation2006; Richetti et al. Citation2011; Javed et al. Citation2012). Moreover, Golisz et al. (Citation2007) reported that rutin possesses growth inhibitory activity against lettuce seedlings. However, this report is the first on the phytotoxic activity of rutin isolated from C. alata leaves. In contrast, syringone is well known for its involvement in plant-pathogen identification and is also important in biotechnology for increasing transformation efficiency in plants (Baker et al. Citation2005; Sheikholeslam and Weeks Citation1987 ). Flores-Gómez et al. (Citation2008) reported that syringone activates root fungus communication in the fungus Glomus intraradices. Although several reports have documented the activity of syringone, as far we know, this report is the first on the phytotoxic activity of syringone.
Therefore, the leaves of C. alata containing phytotoxic substances may have potential as soil additive for weed management option. Because phytotoxic substances are an important source of natural herbicides, these two phytotoxic substances from C. alata could be act as lead for the development of environment friendly new natural herbicides which may provide a new insight into the management of weeds without using synthetic herbicides.
In conclusion, the aqueous methanol extracts of the C. alata leaves showed concentration-dependent and species-specific inhibitory effects on the shoot and root growth of the test plant species, suggesting that this medicinal plant may contain growth inhibitory substances. Two growth inhibitory substances were isolated from C. alata through several chromatographic steps and identified using spectroscopic analysis as rutin and syringone. Both substances inhibited the seedling growth of cress and foxtail fescue. These results on the two identified active substances from this plant indicate that these substances may be responsible for the phytotoxic properties in C. alata, and therefore, could be used to suppress the growth of different weeds in crop fields. However, more investigation is required to corroborate our findings under field conditions.
Acknowledgement
We thank Professor Dennis Murphy, The United Graduate School of Agricultural Sciences, Ehime University, Japan for checking and editing the English of the manuscript.
Disclosure statement
No potential conflict of interest was reported by the authors.
Notes on contributors
Krishna Rany Das is a PhD student, The United Graduate School of Agricultural Sciences, Ehime University, Japan.
Arihiro Iwasaki is an Assistant Professor, Faculty of Science and Technology, Keio University, Japan.
Kiyotake Suenaga is a Professor, Faculty of Science and Technology, Keio University, Japan.
Hisashi Kato-Noguchi is a Professor, Faculty of Agriculture, Kagawa University, Japan.
References
- Abo KA, Fred-Jaiyesimi AA, Jaiyesimi AE. 2008. Ethnobotanical studies of medicinal plants used in the management of diabetes mellitus in South Western Nigeria. J Ethnopharmacol. 115:67–71. doi: 10.1016/j.jep.2007.09.005
- Agostini S, Desjobert JM, Pergent G. 1998. Distribution of phenolic compounds in the seagrass Posidonia oceanica. Phytochemistry. 48:611–617. doi: 10.1016/S0031-9422(97)01118-7
- Ajose FO. 2007. Some Nigerian plants of dermatologic importance. Int J Dermatol. 46:48–55. doi: 10.1111/j.1365-4632.2007.03466.x
- Amb MK, Ahluwalia AS. 2016. Allelopathy: potential role to achieve new milestones in rice cultivation. Rice Sci. 23:165–183. doi: 10.1016/j.rsci.2016.06.001
- Ankenbauer RG, Nester EW. 1990. Sugar-mediated induction of Agrobacterium tumefaciens virulence genes: structural specificity and activities of monosaccharides. J Bacteriol. 172:6442–6446. doi: 10.1128/jb.172.11.6442-6446.1990
- Appleton J. 2010. Evaluating the bioavailability of isoquercetin. Nat Med J. 2:1–6.
- Awal MA, Ainun N, Hossain MS, Bari MA, Rahman M, Haque ME. 2004. Brine shrimp toxicity of leaf and seed extracts of Cassia alata Linn. and their antibacterial potency. J Med Sci. 4:188–193. doi: 10.3923/jms.2004.188.193
- Baker CJ, Mock NM, Whitaker BD, Roberts DP, Rice CP, Deahl KL, Aver’yanov AA. 2005. Involvement of acetosyringone in plant-pathogen recognition. Biochem Biophys Res Commun. 328:130–136. doi: 10.1016/j.bbrc.2004.12.153
- Bao K, Fan A, Dai Y, Zhang W, Cheng M, Yao X. 2009. Selective demethylation and debenzylation of aryl ethers by magnesium iodide under solvent-free conditions and its application to the total synthesis of natural products. Org Biomol Chem. 7:5084–5090. doi: 10.1039/b916969e
- Benvenuti S, Cioni PL, Flamini G, Pardossi A, Hatcher P. 2017. Weeds for weed control: asteraceae essential oils as natural herbicides. Weeds Res. 57:342–353. doi: 10.1111/wre.12266
- Boonmee S, Iwasaki A, Suenaga K, Kato-Noguchi H. 2018. Evaluation of phytotoxic activity of leaf and stem extracts and identification of a phytotoxic substance from Caesalpinia mimosoides Lamk. Theor Exp Plant Physiol. 30:129–139. doi: 10.1007/s40626-018-0108-3
- Chang S, Tan C, Frankel EN, Barrett DM. 2000. Low-density lipoprotein antioxidant activity of phenolic compounds and polyphenol oxidase activity in selected clingstone peach cultivars. J Agric Food Chem. 48:147–151. doi: 10.1021/jf9904564
- Das KR, Kato-Noguchi H. 2018. Assessment of allelopathic activity of Swietenia mahagoni (L.) seed extracts on different plant species. Australian J Crop Sci. 12:1782–1787. doi: 10.21475/ajcs.18.12.11.p1526
- Duke SO, Dayan FE, Rimando AM, Schrader K, Aliotta G, Oliva A, Romagni JG. 2002. Chemicals from nature for weed management. Weed Sci. 50:138–151. doi: 10.1614/0043-1745(2002)050[0138:IPCFNF]2.0.CO;2
- Fernand VE, Dinh DT, Washington SJ, Fakayode SO, Losso JN, Ravenswaay ROV, Warner IM. 2008. Determination of pharmacologically active compounds in root extracts of Cassia alata L. by use of high performance liquid chromatography. Talanta. 74:896–902. doi: 10.1016/j.talanta.2007.07.033
- Flores-Gómez E, Gómez-Silva L, Ruiz-Medrano R, Xoconostle-Cázares B. 2008. Role of acetosyringone in the accumulation of a set of RNAs in the arbuscular mycorrhiza fungus Glomus intraradices. Int Microbiol. 11:275–282.
- Forino M, Tartaglione L, Dell’Aversano C, Ciminiello P. 2016. NMR-based identification of the phenolic profile of fruits of Lycium barbarum (goji berries). Isolation and structural determination of a novel N-feruloyl tyramine dimer as the most abundant antioxidant polyphenol of goji berries. Food Chem. 194:1254–1259. doi: 10.1016/j.foodchem.2015.08.129
- Ganeshpurkar A, Saluja AK. 2017. The pharmacological potential of rutin. Saudi Pharmaceutical J. 25:149–164. doi: 10.1016/j.jsps.2016.04.025
- Ghani A. 1998. Medicinal plants of Bangladesh. Asiatic Society of Bangladesh.
- Golisz A, Lata B, Gawronski SW, Fujii Y. 2007. Specific and total activities of the allelochemicals identified in buckwheat. Weed Biol & Manage. 7:164–171. doi: 10.1111/j.1445-6664.2007.00252.x
- Guerrero L, Castillo J, Quinones M, Garcia-Vallve S, Arola L, Pujadas G, Muguerza B, Lu JR. 2012. Inhibition of angiotensinconverting enzyme activity by flavonoids: structure-activity relationship studies. Plos One. 7:e49493. doi: 10.1371/journal.pone.0049493
- Hirt HM, Binadanda M, Lindsey K. 2008. Natural medicine in the tropics. Anamed: Winnenden Germany.
- Hemlata Kalidhar SB. 1993. Alatinone, an anthraquinone from Cassia alata. Phytochemistry. 32:1616–1617. doi: 10.1016/0031-9422(93)85192-T
- Hennebelle T, Weniger B, Joseph H, Sahpaz S, Bailleul F. 2009. Senna alata. Fitoterapia. 80:385–393. doi: 10.1016/j.fitote.2009.05.008
- Hong NH, Xuan TD, Eiji T, Khanh TD. 2004. Paddy weed control by higher plants from Southeast Asia. Crop Protect. 23:255–261. doi: 10.1016/j.cropro.2003.08.008
- Idu M, Omonigho SE, Igeleke CL. 2007. Preliminary investigation on the phytochemistry and antimicrobial activity of Senna alata L. flower. Pak J Biol Sci. 10:806–809. doi: 10.3923/pjbs.2007.806.809
- Islam AKMM, Ohno O, Suenaga K, Kato-Noguchi H. 2014. Two novel phytotoxic substances from Leucas aspera. J Plant Physiol. 171:877–883. doi: 10.1016/j.jplph.2014.03.003
- Islam MS, Iwasaki A, Suenaga K, Kato-Noguchi H. 2017. Evaluation of phytotoxic potential and identification of phytotoxic compounds in Rumex maritimus. Plant Biosys. 152:804–809. doi: 10.1080/11263504.2017.1338630
- Janbaz KH, Saeed SA, Gilani AH. 2002. Protective effect of rutin on paracetamol and CCl4-induced hepatotoxicity in rodents. Fitoterapia. 73:557–563. doi: 10.1016/S0367-326X(02)00217-4
- Javed H, Khan MM, Ahmad A, Vaibhav K, Ahmad ME, Khan A, Ashafaq M, Islam F, Siddiqui MS, Safhi MM, Islam F. 2012. Rutin prevents cognitive impairments by ameliorating oxidative stress and neuroinflammation in rat model of sporadic dementia of Alzheimer type. Neuroscience. 17:340–352. doi: 10.1016/j.neuroscience.2012.02.046
- Jovanovic SV, Steenken S, Hara Y, Simic MG. 1996. Reduction potentials of flavonoid and model phenoxyl radicals. Which ring in flavonoids is responsible for antioxidant activity? J Chem Soc Perkin Trans. 2:2497–2504. doi: 10.1039/p29960002497
- Kato-Noguchi H, Suzuki M, Noguchi K, Ohno O, Suenaga K, Laosinwattana C. 2016. A potent phytotoxic substance in Aglaia odorata Lour. Chem Biodivers. 13:549–554. doi: 10.1002/cbdv.201500175
- Khan MH, Yadava PS. 2010. Antidiabetic plants used in Thoubal district Nanipur, Northeast India. Indian J Trad Knowl. 9:510–514.
- Krewson CF, Naghski J. 1952. Some physical properties of rutin. J Am Pharm Assoc. 41:582–587. doi: 10.1002/jps.3030411106
- Kuddus MR, Ali MB, Rumi F, Aktar F, Rashid MA. 2011. Evaluation of polyphenols content and cytotoxic, membrane stabilizing and antimicrobial activities of seed of Rumex maritimus Linn. Bangladesh Pharm J. 14:67–71.
- Levy A, Lewis A. 2011. Cassia alata leaf extract induces cytotoxicity in A549 lung cancer cells via a mechanism that is caspase 8 dependent. West Indian Med J. 60:608–614.
- Macikova P, Halouzka V, Hrbac J, Bartak P, Skopalova J. 2012. Electrochemical behavior and determination of rutin on modified carbon paste electrodes. Sci World J. 2012( Article ID 394756):1–9. doi: 10.1100/2012/394756
- Mahmood I, Imadi SR, Shazadi K, Gul A, Hakeem KR. 2016. Effect of pesticides on environments. In: Hakeem K, Akhtar M, Abdullah S, editor. Plant, soil and microbes. Cham: Springer; p. 253–269.
- Mellou F, Loutrari H, Stamatis H, Roussos C, Kolisis FN. 2006. Enzymatic esterification of flavonoids with unsaturated fatty acids: effect of the novel esters on vascular endothelial growth factor release from K562 cells. Process Biochem. 41:2029–2034. doi: 10.1016/j.procbio.2006.05.002
- Mushtaq MN, Cheema ZA, Khaliq A, Naveed MR. 2010. A 75% reduction in herbicide use through integration with sorghum + sunflower extracts for weed management in wheat. J Sci Food and Agric. 90:1897–1904. doi: 10.1002/jsfa.4159
- Nebo L, Varela RM, Molinillo JMG, Sampaio OM, Severino VGP, Cazal CM, Das MF, Fernandes G, Fernandes JB, Macias FA. 2014. Phytotoxicity of alkaloids, coumarins and flavonoids isolated from 11 species belonging to the Rutaceae and Meliaceae families. Phytochem L. 8:226–232. doi: 10.1016/j.phytol.2014.02.010
- Osuagwu GGE, Anyanwu MI. 2014. The effect of aqueous leaf and stem extracts of Senna alata (L) Roxb. on the flowering and fruiting of okra (Abelmoschus esculentus and groundnut (Arachis hypogea). J Pharm Biol Sci. 9:70–73.
- Owoyale JA, Olatundi GA, Oguntoye SO. 2005. Antifungal and antibacterial activities of an alcoholic extract of Senna alata leaves. J Appl Sci Environ Manage. 3:1105–1107.
- Panichayupakaranant P, Kaewsuwan S. 2004. Bioassay guided isolation of the antioxidant constituent from Cassia alata L. leaves. Songklanakarin J Sci Technol. 26:103–107.
- Pimentel D, Burgess M. 2014. Environmental and economic costs of the application of pesticides primarily in the United States. In: Pimentel D, Peshin R, editor. Integrated pest management. Dordrecht: Springer; p. 47–71.
- Prihantini AI, Tachibana S, Itoh K. 2015. Antioxidant active compounds from Elaeocarpussylvestris and their relationship between structure and activity. Proc Environ Sci. 28:758–768. doi: 10.1016/j.proenv.2015.07.089
- Promgool T, Pancharoen O, Deachathai S. 2014. Antibacterial and antioxidative compounds from Cassia alata Linn. Songklanakarin J Sci Technol. 36:459–463.
- Raja NI, Bano A, Rashid H, Chaudry Z, Ilyas N. 2010. Improving Agrobacterium-mediated transformation protocol for integration of XA21 gene in wheat (Triticum aestivum L. Pak J Bot. 42:3613–3631.
- Richetti SK, Blank M, Capiotti KM, Piato AL, Bogo MR, Vianna MR, Bonan CD. 2011. Quercetin and rutin prevent scopolamine-induced memory impairment in zebrafish. Behav Brain Res. 217:10–15. doi: 10.1016/j.bbr.2010.09.027
- Seruga M, Tomac I. 2017. Influence of chemical structure of some flavonols on their electrochemical behaviour. Int J Electrochem Sci. 12:7616–7637. doi: 10.20964/2017.08.79
- Sheikholeslam SN, Weeks DP. 1987. Acetosyringone promotes high efficiency transformation of Arabidopsis thaliana explants by Agrobacterium tumefaciens. Plant Mole Biol. 8:291–298. doi: 10.1007/BF00021308
- Trumbeckaite S, Bernatoniene J, Majiene D, Jakstas V, Savickas A, Toleikis A. 2006. The effect of flavonoids on rat heart mitochondrial function. Biomed Pharmacother. 60:245–248. doi: 10.1016/j.biopha.2006.04.003
- Ullah N, Haq IU, Mirza B. 2015. Phytotoxicity evaluation and phytochemical analysis of three medicinally important plants from Pakistan. Toxicol Ind Health. 31:389–395. doi: 10.1177/0748233712472527
- Varshney S, Hayat S, Alyemeni MN, Ahmad A. 2012. Effects of herbicide applications in wheat fields: is phytohormones application a remedy? Plant Signal Behav. 7:570–575. doi: 10.4161/psb.19689
- Villaseñor IM, Canlas AP, Pascua MP, Sabando MN, Soliven LA. 2002. Bioactivity studies on Cassia alata Linn. leaf extracts. Phytother Res. 16:93–96. doi: 10.1002/ptr.768
- Vivekanandan KE, Ajeesh PR. 2018. Phytochemical screening and antibacterial evaluation of the leaf and root extracts of Cassia alata. Int J Res Appl Sci Eng Tech. 6:820–826. doi: 10.22214/ijraset.2018.3131
- Xuan TD, Tsuzuki E, Uematsu H, Terao H. 2001. Weed control with alfalfa pellets in transplanting rice. Weed Biol Manage. 1:231–235. doi: 10.1046/j.1445-6664.2001.00034.x