ABSTRACT
New Zn sources have emerged recently, which may boost the beneficial effects of leaf sprays on rice cropping. Thus, this study aimed to compare the effects of foliar sprays using Zn chelate against those with new Zn sources such as sorbitol-stabilised Zn nitrate and Zn oxide nanoparticles on rice seed enrichment and production. Treatments were arranged in a completely randomised design, comprising of three Zn sources and four Zn concentrations with four replicates. Rice plants were sprayed three times: at the beginning of tillering, maximum tillering, and booting stage. Experimental unity consisted of 7-dm³ pots filled with 5-dm³ medium textured sand. Each pot had 10 plants, of which 6 randomly harvested at the flowering stage and the remaining at the maturity stage. Plant dry matter, content and accumulation of Zn in the shoot, seed Zn content, and seed production at the grain-filling stage were also estimated. Seed production and Zn accumulation showed a quadratic behaviour with the use of novel sources. Overall the new Zn sources were more effective than the traditional EDTA chelated Zn in terms of rice seed production and quality, especially the sorbitol-stabilised Zn nitrate.
Introduction
Zinc (Zn) is an important micronutrient for plants, since it takes part in several biochemical processes such as protein synthesis (Kambe et al. Citation2015). More than half of the major crops are affected by Zn deficiency (Swamy et al. Citation2016; Lee et al. Citation2017). In rice crops, this nutrient can be applied via foliar sprays at lower cost and provide satisfactory results in terms of plant growth and development (Guo et al. Citation2016).
Foliar fertilisation has been used to enrich rice seeds (grains and husk) with Zn and hence increasing their quality. The high content of Zn in the seeds ensure its supply in the early stages of the following season and improve plant growth.
Currently, the most used Zn source is Zn chelate, which is more efficient when compared to non-chelated salts (Shivay et al. Citation2016). However, new sources have arisen such as Zn oxide nanoparticles and sorbitol-stabilised Zn nitrate. The former has a very small particle size, about 50 nm, which might favour its absorption (Kolodziejczak-Radzimska and Jesionowski Citation2014; Sabir et al. Citation2014). The second has a sugar (sorbitol) that lengthens the absorption process and delays solution drying on the leaf surface by reducing the deliquescence point of Zn+ Sorbitol formulation (Will et al. Citation2011). Nevertheless, the performance of these new sources of Zn foliar fertilisation of rice crop is yet to be investigated in detail.
These new sources should be used focusing on rice farming sustainability in different regions of the world. An emerging hypothesis is whether Zn foliar application would improve grain yield and seed quality in rice crop by increasing contents of this nutrient. It is believed that the new zinc sources may be more efficient in providing the micronutrient than are the traditionally used chelated ones. Therefore, if this hypothesis is correct, this study will be able to strengthen foliar fertilisation practices with Zn in rice seed production fields since farmers have not used them yet.
In short, the aim of this study was to compare the effect of Zn foliar spraying using Zn chelate (Zn-EDTA) and new sources, e.g. Zn oxide nanoparticles (Zn-Nano), and sorbitol-stabilised Zn nitrate (Zn-N), on seed Zn enriching and production.
Materials and methods
Growth conditions
Plants of rice cultivar BRS-Esmeralda were grown under a soilless cultivation system in a greenhouse of the São Paulo State University (UNESP), campus in Jaboticabal-SP (Brazil), from January to April 2016. During the experimental period, climate variables were measured: relative air humidity – RH (54.5% ± 29.5), minimum temperature – Tmin (20.4°C ± 2.3), and maximum temperature – Tmax (38.1°C ± 8.1). Based on the data, we observed that the plants were grown at maximum temperatures higher than those indicated for an optimum rice development (i.e. from 20°C to 35°C) (Yoshida et al. Citation1981).
One experimental unit consisted of a 7-dm³ polypropylene pot (16-cm top diameter, 11-cm bottom diameter, and 33-cm height) filled with 6-dm³ medium textured sand, which was previously sterilised with water, 1%HCl solution, and deionised water. Each pot contained ten plants, of which six were sampled at flowering stage and the remaining ones at the maturity stage.
After sowing, pots were watered three times a day with deionised water until emergence. Then, nutrient solution application (Hoagland and Arnon Citation1950) started, using 20% of the indicated concentration for 7 days, increased to 50% for the next 7 days, and finally to 75% which was maintained until the end of the experiment. Deionised water was weekly applied to the pots for substrate drainage and salinisation control.
Experimental design
Treatments were arranged in a completely randomised design in a 3 × 4 factorial scheme, with four replicates. The zinc sources evaluated here were chelated zinc EDTA (from zinc sulfate heptahydrate) (14 g L−1 of Zn), sorbitol-stabilised Zn nitrate (Zn-N) (74.3 g L−1 of Zn; 32.1 g L−1 of N), and Zn oxide nanoparticulles Zn-Nano) (74.3 g L−1 of Zn, 8.8- µm agglomerates, and 49.9-nm particle diameter); the foliar spray concentrations were 0.0, 0.4, 1.0 and 2.0 g L−1 Zn. Ammonium nitrate was applied to balance nitrogen to a uniform level among the different sources.
Zinc foliar application
Leaf sprays with Zn were made three times: at the beginning of tillering (V4), at maximum tillering (V5), and booting (R2). The pH of spray solution was adjusted between 6.0 and 6.5, and the spray volume varied with to plant size. We applied 2, 4, and 6 mL spray solution per pot in the first, second, and third sprayings, respectively. Treatments were performed immediately after solution preparation, using a backpack sprayer over the entire plant shoots. Temperature and relative air humidity were measured during the first, second and third leaf sprays, being of 23.2°C and 84%, 29.2°C and 70% and 30.4°C and 60%, respectively.
Leaf amino acid and protein concentration in leaves
At flowering, flag leaves and three successively lower leaf blades were collected from six plants of each experimental unit; then, they were washed in deionised water, 0.1% detergent solution, 0.1% HCl solution, and deionised water, packed in paper bags and dried in forced air circulation oven at 65°C ± 5 until constant weight. Afterward, protein and amino acid concentration was determined following the method described by Galvani and Gaertner (Citation2006), and Peoples et al. (Citation1989), respectively.
Shoot height, dry matter and Zn accumulation
At maturity, plant height was measured from the stem-base to the first node insertion in the first fully expanded leaf. Also, the remaining plants were harvested and separated into leaves, stem, panicles, and roots. Leaf and stem samples were washed with deionised water, 0.1% detergent solution, 0.1% HCl solution, and deionised water, packed in paper bags and dried in a forced air circulation oven at 65°C ± 5 until constant weight. Afterward, plant shoot was ground in a Wiley mill and used for Zn content analysis through the method described by Bataglia et al. (Citation1978). Zinc accumulation was obtained from the product of Zn content in the plant tissue and dry matter.
Seed production and Zn content
Seeds were manually removed from panicles for determination of grain yield per pot; for that, whole grains were weighed and corrected to 13% moisture content. Thereafter, they were ground for Zn content analysis according to Bataglia et al. (Citation1978).
Statistical analysis
All data were submitted to analysis of variance by the F test. When significant, the means of Zn sources were compared by the Tukey’s test at 5% probability. For Zn concentrations, a polynomial regression analysis was performed to select the model with the highest coefficient of determination (at 5% probability). The analyses were performed with the aid of AgroEstat® statistical software (Barbosa and Maldonado Júnior Citation2010).
Results
Zinc accumulation increased in plant shoot as concentration was raised. Both Zn-EDTA and Zn-N showed a quadratic adjustment with maximum points at 1.07 and 1.37 g L−1 of Zn, respectively, whereas Zn-Nano had a linear adjustment ((a)). Among the sources, Zn-N stood out at 0.4 g L−1 of Zn; yet, no difference was observed among them at 1 g L−1 of Zn. However, at the highest studied concentration, Zn-Nano showed the highest Zn accumulation per pot ((a)).
Figure 1. Zn accumulation (a); amino acid content (b), protein content (c) and shoot dry mass (d) of rice plants grown under different Zn sources (S): chelated zinc EDTA (EDTA-Zn), sorbitol-stabilised zinc nitrate (N-Zn); and zinc oxide nanoparticles (Nano-Zn); and different concentrations (C), grown in a greenhouse. ns, * and **, not significant and significant at 5% and 1% probability. Different letters differ the sources in the same Zn concentration by the Tukey test at 5% of probability.
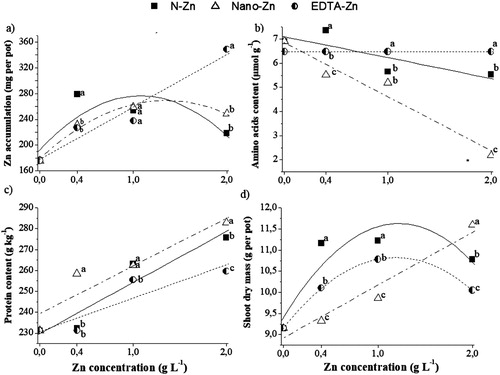
Amino acid contents in rice leaves had a decreasing linear adjustment to the increase of Zn concentration for Zn-N and Zn-Nano, but non-significant for Zn-EDTA ((b)). At 0.4 g L−1, Zn-Nano showed the lowest amino acid content. Conversely, at 1.0 g L−1 of Zn, Zn-EDTA presented the highest amino acid content in leaves and no differences between Zn-Nano and Zn-N. Moreover, at 2.0 g L−1 Zn, the lowest leaf amino acid content was observed for Zn-Nano.
Protein production increased linearly with the use of all tested sources ((c)). All concentration of Zn-Nano showed the highest protein content. At 1.0 g L−1 Zn, no difference was observed between Zn-Nano and Zn N in terms of leaf protein content.
No significant differences were found among the sources for height; however, higher plants were observed at 1.0 g L−1 of Zn ().
Table 1. Height of rice plants under different sources and concentrations of Zn applied via foliar.
In general, Zn supply via foliar spraying promoted increases in dry matter production. Zn-EDTA and Zn-N showed a quadratic adjustment with maximum points at 1.18 and 1.21 g L−1 Zn, respectively, while Zn-Nano had a linear adjustment ((d)). In terms of shoot dry matter production, the concentrations of 0.4 and 1.0 g L−1 Zn stood out for Zn-N, and 2.0 g L−1 Zn for Zn-Nano.
All the Zn sources increased seed production ((a)) and seed Zn content ((b)) as the concentration in solutions increase, showing a quadratic adjustiment. The highest values were found for Zn-N at the concentration of 1.0 and 2.0 g L−1 Zn. However, none of the sources differed significantly from each other at 0.4 g L−1 Zn.
Figure 2. Seed production (a), and Zn content in seeds (b) of rice plants under different Zn sources (S) leaf aplication: chelated zinc EDTA (EDTA-Zn), sorbitol-stabilised zinc nitrate (N-Zn); and zinc oxide nanoparticles (Nano-Zn); and different concentrations (C), grown in a greenhouse. **, significant at 1% probability. Different letters differ the sources in the same Zn concentration by the Tukey test at 5% of probability.
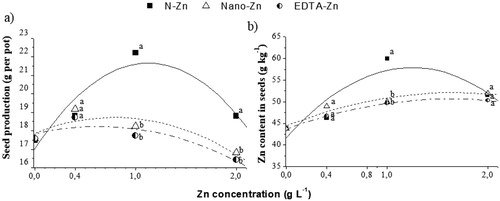
Discussion
The efficiency of foliar sprays of Zn on rice plants in influenced by Zn source and concentration used ((a)). The linear increase in Zn shoot accumulation by Zn-Nano ((a)) highlights its efficiency in supplying the nutrient to plant. Sabir et al. (Citation2014) have already pointed out its effectiveness over other sources. However, at extremely low concentration (e.g. 0.4 g L−1 Zn), the source showed lower efficiency if compared to the Zn-N. This might be explained by the high solubility of Zn-N and the presence of sorbitol, which acts as an adjuvant, breaking the water surface tension and increasing the spread of droplets on the leaf surface, thus enhancing absorption even at low concentrations.
All studied sources promoted low protein content ((c)) and high amino acid concentration ((b)) in the absence of Zn, which can be attributed to the role of this micronutrient in the ribosome structure (Prask and Plocke Citation1971), activation of RNA polymerase, and inhibition of RNAase (Cakmak et al. Citation1996), as well as auxin synthesis, which also affects protein production(Begum et al. Citation2016). However, the increased Zn concentration in the leaves and the consequent higher Zn accumulation ((a)) lead to increased conversion of amino acids into proteins. At 2.0 g L−1 Zn, Zn-Nano showed the lowest amino acid content ((b)) and highest protein content ((c)), which is due to the highest Zn accumulation in plant shoots.
In terms of shoot dry matter, the best performance of Zn-N at 0.4 g L−1 Zn could be attributed to a higher Zn accumulation in the plant ((a)). This product also had a higher dry matter production at 1.0 g L−1 Zn ((d)), even though micronutrient accumulation was similar between the Zn-Nano and Zn-EDTA. This result indicates the higher efficiency of Zn-N in rice plants.
This effect of Zn on pant height is well known since it is involved in the synthesis of indole-acetic acid (AIA). This phytohormone induces elongation between internodes, and hence plant height. At the highest concentration, Zn-Nano stood out from the other sources regarding shoot dry matter production ((d)). This result is related to the increase in leaf Zn accumulation and amino acid decrease, in addition to protein content increase, which serves as substrate for dry matter production.
The efficiency of Zn-N at 1.0 and 2.0 Zn was evidenced in seed production. Despite its good performance in accumulating Zn in plant and increasing dry matter production, it had no effect on productivity. This effect possibly occurred because part of the Zn absorbed from Zn-Nano may be in the non-bioavailable form since it is a ZnO. This form needs an acid intercellular medium to form Zn2+ and not being completely used in plant metabolism (Miao et al. Citation2010). Furthermore, when totally dissociated into Zn2+, Zn-N would be fully bioavailable (Dagnall et al. Citation1982) and its complexation with sorbitol could decrease nutrient adsorption with carboxylic compounds of leaf cuticle.
Finally, our findings accept the hypothesis indicating that sorbitol-stabilised zinc nitrate is more efficient than Zn chelate for foliar spraying of rice crop. This opens new perspectives on Zn foliar fertilisation in rice crop and indicates the possibility of conciliating crop quality and productivity.
Disclosure statement
No potential conflict of interest was reported by the authors.
Notes on contributors
R. C. F. Alvarez holds PhD in agronomy from Paulista State University “Júlio de Mesquita Filho” (UNESP), Campus of Ilha Solteira. Currently, she is Adjunct Professor II of the Federal University of Mato Grosso do Sul and has experience in agronomy with emphasis on agriculture, working mainly with components of the production.
R. M. Prado holds PhD in agronomy from UNESP, Campus of Jaboticabal. Currently, he is an associate professor of UNESP, Campus of Jaboticabal and has experience in plant nutrition. He is the coordinator of the group of studies in nutrition of plant of the unesp (Genplant) that works with the subjects: studies of nutritional disorders; nutrient mobility; leaf nutrition, nutritional efficiency; silicon, leaf diagnoses and plant nutrition.
J. P. Souza Júnior, PhD student at UNESP, Campus of Jaboticabal, is a member of the Genplant. Currently Jonas Pereira de Souza Júnior is working on the thesis “relationship between boron and silicon in cotton plants grown under boron deficiency”.
R. L. L. Oliveira, PhD student at Rural Federal University of Amazônia, works with research in management and conservation of environmental resources.
G. Felisberto holds PhD in agronomy from UNESP, Campus of Jaboticabal. Currently, he works in the coordinate of sustainable rural development of the Secretary of Agriculture and Supply of the State of São Paulo.
A. C. F. Deus holds PhD in agronomy from UNESP, Campus of Botucatu. She has experience in soil, soil fertility, fertilisers and plant nutrition.
F. J. R. Cruz holds PhD in agronomy by UNESP, Campus of Jaboticabal. He works with plant physiology focused on studies of abiotic stress in plants of agricultural and forestry interest.
References
- Barbosa JC, Maldonado Júnior W. 2010. Agroestat: Sistema para análises estatísticas de ensaios agronômicos [ System for statistical analysis of agronomic trials]. Jaboticabal: Unesp.
- Bataglia OC, Teixeira JPF, Furlani PR, Furlani AMC, Gallo JR. 1978. Métodos de análise química de plantas [ Methods of chemical analysis of plants]. Campinas: IAC.
- Begum MC, Islam M, Sarkar MR, Azad MAS, Huda AKMN, Kabir AH. 2016. Auxin signaling is closely associated with Zn-efficiency in rice (Oryza sativa L.). J Plant Interac. 11(1):124–129. doi: 10.1080/17429145.2016.1220026
- Cakmak I, Yilmaz A, Kalayci M, Ekiz H, Torun B, Braun HJ. 1996. Zinc deficiency as a critical problem in wheat production in Central Anatolia. Plant Soil. 180:165–172. doi: 10.1007/BF00015299
- Dagnall SP, Hague DN, Towl ADC. 1982. X-ray diffraction study of aqueous zinc(II) nitrate. J Chem Soc Faraday Trans. 78:2161. doi: 10.1039/f29827802161
- Galvani F, Gaertner E. 2006. Adequação da metodologia Kjeldahl para determinação de nitrogênio total e proteína bruta [ Adequacy of the Kjeldahl methodology for the determination of total nitrogen and crude protein]. Corumbá: Pantanal.
- Guo JX, Feng XM, Hu XY, Tian GL, Ling N, Wang JH, Shen QR, Guo SW. 2016. Effects of soil zinc availability, nitrogen fertilizer rate and zinc fertilizer application method on zinc biofortification of rice. J Agric Sci. 154(4):584–597. doi: 10.1017/S0021859615000441
- Hoagland DR, Arnon DI. 1950. The water-culture method for growing plants without soil. Circ Calif Agric Exp Stn. 347(2):32.
- Kambe T, Tsuji T, Hashimoto A, Itsumura N. 2015. The physiological, biochemical, and molecular roles of zinc transporters in zinc homeostasis and metabolism. Physiol Rev. 95(3):749–784. doi: 10.1152/physrev.00035.2014
- Kołodziejczak-Radzimska A, Jesionowski T. 2014. Zinc oxide - from synthesis to application: a review. Materials. 7:2833–2881. doi: 10.3390/ma7042833
- Lee JS, Wissuwa M, Zamora OB, Ismail AM. 2017. Biochemical indicators of root damage in rice (Oryza sativa) genotypes under zinc deficiency stress. J Plant Res. 130:1071–1077. doi: 10.1007/s10265-017-0962-0
- Miao AJ, Zhang XY, Luo Z, Chen CS, Chin WC, Santschi PH, Quigg A. 2010. Zinc oxide-engineered nanoparticles: dissolution and toxicity to marine phytoplankton. Environ Toxicol Chem. 29:2814–2822. doi: 10.1002/etc.340
- Peoples MB, Faizah AW, Rerkasem B, Herridge DF. 1989. Methods for evaluating nitrogen fixation by nodulated legumes in the field. Monographs. 11(7):39–40.
- Prask JA, Plocke DJ. 1971. A role for zinc in the structural integrity of the cytoplasmic ribosomes of Euglena gacilis. Plant Physiol. 48:150–155. doi: 10.1104/pp.48.2.150
- Sabir S, Arshad M, Chaudhari SK. 2014. Zinc oxide nanoparticles for revolutionizing agriculture: synthesis and applications. Sci J. 2014:1–8.
- Shivay YS, Prasad R, Kaur R, Pal M. 2016. Relative efficiency of zinc sulphate and chelated zinc on zinc biofortification of rice grains and zinc use-efficiency in basmati rice. Proc Natl Acad Sci India Sect B Biol Sci. 86:973–984. doi: 10.1007/s40011-015-0544-7
- Swamy BPM, Rahman MA, Inabangan-Asilo MA, Amparado A, Manito C, Chadha-Mohanty P, Reinke R, Slamet-Loedin IH. 2016. Advances in breeding for high grain zinc in rice. Rice. 9:49. doi: 10.1186/s12284-016-0122-5
- Will S, Eichert T, Fernández V, Möhring J, Müller T, Römheld V. 2011. Absorption and mobility of foliar-applied boron in soybean as affected by plant boron status and application as a polyol complex. Plant Soil. 344:283–293. doi: 10.1007/s11104-011-0746-6
- Yoshida S, Satake T, Mackill DS. 1981. High temperature stress in rice. IRRI Res Pap Ser 67.