ABSTRACT
The tillage system, which joins soil tillage, fertilisation, and seed sowing in one pass of a machine, is rarely used in cereal cultivation. This research aimed to study whether strip-till and conventional tillage (post-harvest ripping and mixing of stubble, ploughing, pre-sowing fertilisation, and seedbed preparation) differ in plant density, yield components, and grain yield of winter barley cultivated on Cambisol in a region with low rainfall, the annual average is about 500 mm. To reach this aim, a two-year, large-plot experiment was conducted in a production field. The soil within the field was spatially variable in texture, moisture, and chemical and biological properties, CV 2.3% do 29.6%. Strip-till had lower within-field spatial variability of winter barley grain yield than conventional tillage. The standard deviation was 0.36 t ha−1, 0.67 t ha−1 in the first year and 0.12 t ha−1, 0.30 t ha−1 in the second year, respectively. During the period of limited rainfall, strip-till had greater uniformity of plants after emergence; after the winter with low air temperature and a low amount of snow, it had greater plant density. Thus, strip-till can reduce variability of plants and their yield within a field, especially in adverse environmental conditions.
Introduction
Plant uniformity is an important element of the architecture of crop plants that limits within- and between-species competition (Olsen et al. Citation2006; Olsen and Weiner Citation2007) and depends on both genetical and environmental factors (Makumburage and Stapleton Citation2011; Huang et al. Citation2017). Designed experiments, even those conducted on small plots with only a few replications, offer a possibility of limiting the variability of environmental conditions and their impact on the uniformity of plants (Lavezo et al. Citation2017), but such limiting of conditions is much more difficult in most production fields. In production conditions, the spatial and temporal variability of plants in height, density, and area of organs result from the genetic and environmental factors as well as agrotechnical measures (Barthélémy and Caraglio Citation2007; Tivoli et al. Citation2013). Plant development depends on, among others, seed size and vigour (Ambika et al. Citation2014; Patel et al. Citation2016). From large seeds of high vitality emerge larger plants than from small seeds of low vitality, and they emerge more quickly; such plants are also more resistant to adverse environmental conditions, thanks to which they are more uniform (Willenborg et al. Citation2005).
Across the arable fields there is often a high variation in the properties of soil, in time and space, in the layers at different depths (Iqbal et al. Citation2005; Delbari et al. Citation2011). Physical and chemical properties can be strongly differentiated, such as soil texture, bulk density, porosity, aggregate stability, soil moisture, saturated hydraulic conductivity, exchangeable acidity, pH, exchangeable capacity of cations, and contents of Ca, Na, K, Mg, P, N, and organic matter (Brocca et al. Citation2010; Cruz et al. Citation2011; Rabbi et al. Citation2014). Variability of physical and chemical properties of soil affects spatial variability of its biological properties. Piotrowska et al. (Citation2011) showed significant variability of within-field enzyme activity from 0.77 to 1.5 μM TPP g−1 h−1 for dehydrogenase and from 0.8 to 1.94 μM glucose g−1 24 h−1 for cellulase. This variability was related to the spatial variability of organic carbon and total nitrogen contents in the soil. Soil structure and organic carbon content can affect the distribution of weeds in a field, an important factor determining plant growth (Gaston et al. Citation2001). Another important factor differentiating soil conditions is plant residues (Bannari et al. Citation2015), which protect the soil against erosion and affect water content. Together with the tillage system, plant residues affect the physical, chemical, and biological properties of the soil (Derpsch et al. Citation2010; Lemtiri et al. Citation2016).
Soil tillage – treated here as an agrotechnical measure – is among the most important factors determining the conditions of seed germination and plant growth. It affects many soil properties, such as moisture, temperature, bulk density, resistance to penetration, and stability of soil aggregates (Alvarez and Steinbach Citation2009); the strength of this influence depends on the tillage system and its interaction with the environment (Busari et al. Citation2015; Jabro et al. Citation2016). Each tillage method allows for creating favourable conditions for crop growth. However, an intensive plough tillage increases the risk of soil erosion, degradation of soil organic matter, water losses, a decrease in the biological activity of microorganisms. Under the habitat conditions increasing that risk, conservation tillage is better as it enhances many soil properties (He et al. Citation2010; Sapkota Citation2012).
Strip-till is a modern cultivation method that combines the advantages of conventional tillage, no-till cultivation, and direct sowing. It uses minimum tillage, thanks to which it only slightly affects the soil by loosening it in rows – the only part of soil that strip-till affects – thereby creating good conditions for seed germination and root growth (Evans et al. Citation2010; Übelhör et al. Citation2014). It is seldom used in cereal cultivation because of the construction of strip-till drills and the narrow row spacing of plants sown in high density (Hossain et al. Citation2014). The most efficient are hybrid machines, which in one pass make it possible to cultivate the soil, apply fertilisers, and sow seeds. Combining many agrotechnical measures into one improves the environment, soil properties, and the economic efficiency of plant cultivation, as compared with conventional tillage, based on ploughing. After several years of using strip-till, the content of organic matter in the top soil and the stability of soil aggregates increase (Al-Kaisi et al. Citation2014; Fernandez et al. Citation2015). In addition, this tillage system requires smaller fuel consumption and shorter work time than conventional tillage, thereby reducing cultivation costs and work input (Overstreet Citation2009).
In the scientific literature well-documented results of research on the effect of the strip-till system on the reaction of plants to the spatial variation in soil properties at the scale of arable field are missing. For that reason, the aim of the research has been to compare the variation in plant density, yielding components and grain yield for winter barley cultivated following the strip-till and conventional technology.
Material and methods
Experimental site
The experiment was conducted during two growing seasons for winter cereals, namely, 2014/2015 and 2015/2016, on a farm cooperating with Faculty of Agriculture and Biotechnology, University of Science and Technology in Bydgoszcz. The farm is located in Śmielin, (53°09′04.0″N; 17°29′10.7″E; 93,8 m a.s.l.), Poland. The experimental field was 250 m long and 144 m wide, and its soil was a Cambisol (WRB Citation2014) with the following average soil texture over the two years: 45.3% of sand, 48.2% of silt, and 6.6% of clay. The soil contained 10.5 g kg−1 of organic carbon, 1.1 g kg−1 of total nitrogen, 99.1 g kg−1 of available P by Egner-Riehm, 201.9 g kg−1 of available K by Egner-Riehm, 64.9 g kg−1 of available Mg by Schachtschabel, and its pHKCl was 6.1. According to the Köppen climate classification, the experimental site is characterised by a warm-summer humid continental climate (Peel et al. Citation2007). shows the site’s meteorological conditions during the experiment.
Table 1. Meteorological conditions during the studied seasons and long-term period (average from 30 years).
Experimental design
A one-factor field experiment with winter barley (Hordeum vulgare L.) cultivar ‘KWS Meridian’ was designed as a randomised block design with three replications, each replication being a large (250 m length and 24 m wide) plot. Earlier the following crops were consecutively grown in that field: spring barley, winter rapeseed, winter wheat, winter triticale, winter rapeseed. Growing cereals, a reduced ploughless tillage 15 cm deep and growing rapeseed – conventional tillage with plough tillage 20–22 cm deep were applied. The experimental factor consisted of two tillage systems: conventional tillage and strip-till. During the experiment, the production conditions of the farm were kept, so the regular tools for field production on this farm were used, instead of specialised tools for field experimentation. Conventional tillage used stubble cultivator (Horsch Tiger 6 AS), plough (Maschio Gaspardo s4), fertiliser spreader (Amazone ZG-TS 8200), and seed drill (Horsch Pronto 4DC). The distance between the rows was 14.3 cm. Strip-till used the one-pass strip tillage drill Pro-Til 4 T by Mzuri Limited, which simultaneously cultivates the soil, applies fertilisers, and sows seeds in two rows with a spacing of 12 and 24.3 cm.
Agrotechnical management
In both seasons, winter oilseed rape was used as a forecrop. After its harvest, the soil was cultivated, the fertilisation was applied, and the winter barley seeds were sown, all completed with the appropriate technologies for the two treatments. Applied before sowing the seeds in conventional tillage and during sowing in strip-till, fertilising consisted of 27 kg N ha−1, 69 kg P2O5 ha−1 (in the form of ammonium phosphate), and 80 kg K2O ha−1 (in the form of potassium chloride). In the spring, the whole field received additional 102 kg N ha−1 (in the form of ammonium nitrate), applied in two doses: 68 kg N ha−1 at growth stage BBCH 30 and 34 kg N ha−1 at growth stage BBCH 37. Seeds were sown on 16 September 2014 and 20 September 2015, at a density of 300 seeds m−2. Monocotyledonous and dicotyledonous weeds were controlled with the active ingredients diflufenican and isoproturon (herbicide Legato Plus 600 SC at a dose of 1.25 l ha−1, BBCH 13). Prothioconazole and spiroxamine were used against fungal diseases (fungicide Input 460 EC at a dose of 1.0 l ha−1, BBCH 37). The harvest was at the stage of fully ripened grains (BBCH 89), in the first half of July.
Sampling and measurements
Along each plot, sampling points were allocated at an equal distance of approximately 35 m (). Before the experiment, for each treatment (conventional tillage and strip-till), 21 soil samples (seven per plot) were taken from a depth of 0–20 cm. In these sampling points, plant density was determined at three time points: two weeks after sowing (BBCH 11–12), after the end of vegetation (BBCH 22–23) before the winter, and after the beginning of vegetation in the spring (BBCH 23–25). Before harvest, all plants were collected from these sampling points, from the area of 2 m2, to determine spike density, mean grain weight per spike, and grain yield. The soil samples were analysed to determine pH in 1 mol/KCl using pH-meter Schott Gerate CG 840 (Hofheim, Germany), soil texture using particle size analyser Mastersizer 2000 (Malvern Instruments Ltd., UK), organic carbon content and total nitrogen (Vario MAX CN – Elementar Hanau, Germany), and contents of available phosphorus and potassium after extraction by the Egner-Riehm method using the spectrometer Genesis 6 (Madison, USA) – for phosphor and atomic absorption spectrometer AAS, Philips 9100 (Cambridge, UK) – for potassium and magnesium. Absorbable magnesium was extracted from the soil with 0.025 mol/l CaCl2 (with the soil:solution ratio of 1:10), according to the Schachtschabel method. Spatial variation in the momentary water content in experimental field soil was determined as the ratio of mass of water in a sample to mass of dry soil in the sample. The total number of bacteria was determined according to Wallace and Lockhead (Citation1950), and the total number of fungi according to Martin (Citation1950). A Wintersteiger LD 180 laboratory thresher and a laboratory scale were used to assess grain weight per spike and grain yield per 2 m2, recalculated as grain yield ha−1.
Statistical analysis
The influence of the tillage system on the studied plant traits and grain yield of winter barley was analysed by one-way ANOVA. Variability of soil conditions within the experimental field (N = 42) was represented by the minimum and maximum values as well as coefficient of variation (CV) of traits representing physical, chemical, and biological soil properties. The effect of the tillage system on the variability of winter barley plant traits, including grain yield, was analysed by comparing standard deviations (s) and extreme values of the traits for the two systems. Distributions of the traits were presented in box-and-whisker plots. The simple correlation was also evaluated between soil properties and the characteristics of crops in both years depending on the tillage system. The study presents only the soil properties significantly correlated with the characteristics of plants at least in one research year. The results were analysed using Excel 2010 (Microsoft Corporation) and Statistica 12 (StatSoft Inc).
Results
The soil was spatially variable within the experimental field in texture, pH, available nutrients, moisture, and biological properties (). The most variable were bacteria and fungi totals, clay content in soil texture, and soil moisture in the first year of research. Total nitrogen in soil in the 2014/2015 season and available phosphorus in the 2015/2016 season had CV over 10%. CV of the other soil traits, except for pH, ranged from 5.0 to 10.0%.
Table 2. Soil conditions at the experimental site (N = 21 for each treatment).
In both seasons, the tillage system affected all the traits studied (P < 0.05) but one: it did not influence grain weight per spike in the 2015/2016 season (). Two weeks after sowing, strip-till had a higher plant density than conventional tillage in the first season of the study, but lower in the second one. In 2014/2015, the spatial variability of plants after emergence was lower in strip-till – it had a smaller standard deviation, shorter whiskers on the box-and-whiskers plot, and a smaller distance between the extreme value and the mean ().
Figure 2. Within-field variability of plant density of winter barley two weeks after sowing (N = 21 for each treatment).
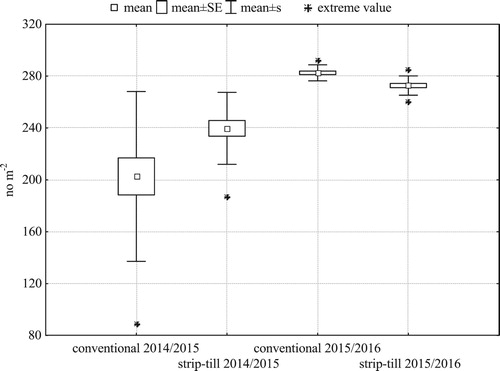
Table 3. P-values representing the influence of the tillage and sowing technology on plant traits of winter barley.
In the first season, plant density two weeks after sowing was larger in strip-till, but in the second season it was lower than in conventional tillage. In 2014/2015, spatial variability of plant density after emergence was lower in strip-till than in conventional tillage: plant density’s standard deviation was lower, as indicated by the shorter whiskers on a box plot and a smaller distance between the extreme value and the mean ().
Before the winter of both seasons, after the end of autumn vegetation, strip-till had significantly lower plant density (). In both years, but especially in 2014/2015, the tillage systems had similar spatial variability of plant density.
Figure 3. Within-field variability of plant density of winter barley before the winter (N = 21 for each treatment).
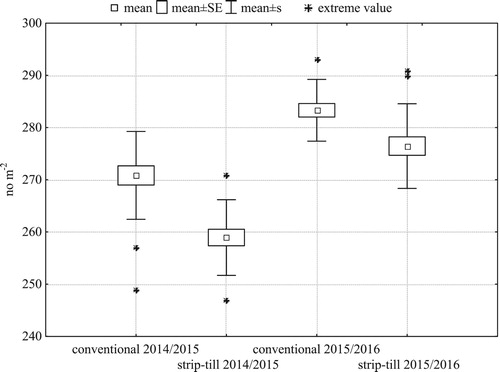
After the winter, plant density decreased in both systems, but more in 2015/2016. After a very unfavourable period of low temperatures and a thin layer of snow in January 2016, strip-till had a greater plant density in the spring than conventional tillage (), even though the situation had been opposite in the autumn. Plant density was also more spatially uniform in strip-till, as indicated by lower standard deviation of plant density than that in conventional tillage.
Figure 4. Within-field variability of plant density of winter barley in the spring (N = 21 for each treatment).
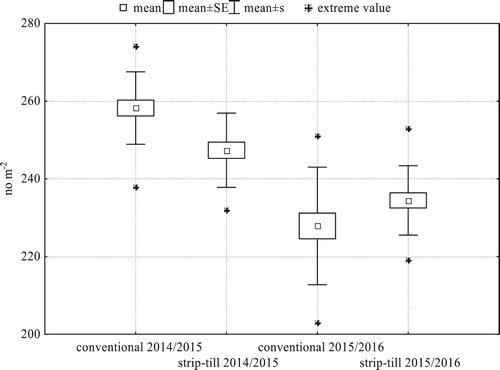
Spike density in both seasons () and grain weight per spike in 2014/2015 () were larger and, more importantly, more spatially uniform in strip-till. In both years, strip-till had significantly higher grain yield than conventional tillage (). Smaller distance between the extreme values and the mean indicate lower spatial variability of grain yield in strip-till than in conventional tillage. The standard deviation of the yield in strip-till in the first and the second research year was 0.36 t ha−1, 0.12 t ha−1, respectively, and it was lower than in the traditional system (0.67 t ha−1, 0.30 t ha−1).
Figure 6. Within-field variability of grain weight per spike of winter barley (N = 21 for each treatment).
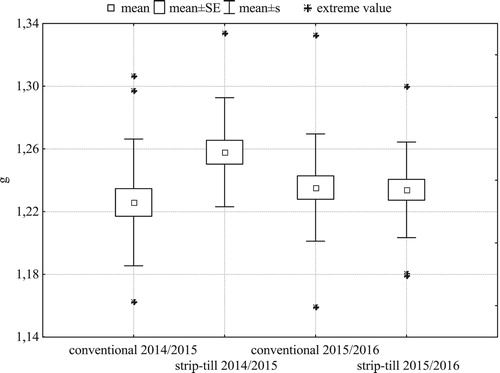
The tillage system affected the correlation between the soil properties and the traits of the plants (). In the first research year, with low precipitation, a higher share of sand and a lower share of silt and clay fraction in over-dried soil in conventional tillage system were favourable for emergence and plant density. Under such precipitation conditions, the density of the winter barley plants grown following the strip-till technology with soil surface mulching with the preceding-crop straw was, however, enhanced by a lower share of sand in the soil texture. In both research years strip-till decreased the dependence of the yield components and the grain yield on soil properties. The coefficients of simple correlation for that object showed a lower value than after conventional tillage and, in most cases, the dependencies were non-significant.
Table 4. Simple correlation coefficients between the soil properties and the plant traits depending on the tillage system.
Discussion
As shown in and environmental conditions, meteorological and soil, were temporally and spatially differentiated. The soil properties variation found in own research, CV from 2.3% to 29.6%, is characteristic for large arable fields. It refers to the soils representing different systematic units and found in different parts of the world. Millán et al. (Citation2012) showed spatial variability of penetration resistance, overall porosity, and water content in a Vertisol (Typic Hapludert). Cortés-D et al. (Citation2016) found spatial variability of penetration resistance in a Typic Haplustox (according to the USDA system). In Central Europe and Poland, many agricultural soils are sandy. Such soils have high spatial variability of its properties (Sándor et al. Citation2015), and such variability of physical and chemical properties within a field comes from morphogenetic and topographical factors as well as agronomic management practices (Jankowski et al. Citation2011; Gajda et al. Citation2016; Gałka et al. Citation2016). In Poland Usowicz and Lipiec (Citation2017), evaluating a spatial variation in Podzol properties within the field with the acreage more than 3-fold lower than in this experiment, also found their high variation. Soil was spatially variable in many physical and chemical properties, including soil texture, organic carbon content, exchange capacity of cations, and pH. The variability occurred in two soil layers: the surface layer (0–10 cm) and subsoil (30–40 cm). The most variable (CV above 30%) were silt, clay, and organic carbon content, cation exchange capacity, and soil moisture. The least variable (CV below 10%) were sand content, pHKCl, and bulk density. The soil (Cambisol) texture was different in the present research: the soil contained less sand, and the contents of silt and clay were more evenly distributed within the field, despite its larger area. Organic carbon content, pHKCl, and soil moisture were also less variable, in general CV < 10%. Out of the soil texture elements the lowest variation in the content of silt at the commercial field scale and soil pH was also reported by Perez-Quezada et al. (Citation2003). Gołaszewski et al. (Citation2008), on the other hand, point to a high variation in space, CV about 20%, the content of available forms of P, K, Mg in soil. Those properties were correlated with the yield and the biometrics of the plants, which was not found in the present research. However, it can be due to a few-fold lower variation in those characteristics. Their CV ranged from 5.7% to 12.5%. However, it should not come as surprise – because within-field spatial variability and nonuniformity of plants depend not only on soil properties (Vieira and Paz Gonzalez Citation2003), but also on the plant species and its variety (Andrade and Abbate Citation2005).
According to Ayoubi et al. (Citation2009), biomass and grain yield of barley strongly depend on soil properties, such as total nitrogen, available phosphorus and potassium contents, exchange capacity of cations, electrical conductivity, pH, mean weight diameter of aggregates, proportion of water-stable aggregates, available water content, and bulk density. The authors showed that over 70% of variance of biomass production and grain yield was due to the soil properties. This explains the large spatial variability of plant density, yield components, and grain yield of winter barley observed in the present research despite the relatively small spatial variability of the soil properties.
However, the correlation between the soil properties and the plant traits was not always significant. The significance and the strength of those dependencies were affected by the tillage system.
The hypothesis of this research was that the tillage system affects the within-field spatial variability of plant traits. The main objective of the research was to determine whether strip-till reduces the spatial variability of plants in the field, thereby increasing their uniformity. The data in indicate that the technology of soil cultivation and sowing affects significantly the growth and yield of plants. It was found that winter barley plants grown following the strip-till technology emerged fast and evenly, they overwintered better and showed a higher spike density as well as they yielded better. A favourable impact of that technology on growth and yielding was mostly recorded in the year with low precipitation. In the light of scientific literature it is understandable as strip-till is one of the conservation tillage methods. It limits soil loosening and leaves mulch on the surface, it increases microporosity, the content of water in soil and its availability to plants (Morris et al. Citation2010; Busari et al. Citation2015).
In the period between sowing (Karayel and Özmerzi Citation2002) and the stage of forming grain yield and its quality (Reyniers et al. Citation2006; Allaire et al. Citation2014; Song et al. Citation2017), spatial variability of plants increases plant competition and makes agrotechnical management difficult (Gan et al. Citation1992). Within-field uniformity of plants in the subsequent development stages results from the interaction of genetic factors, environmental factors, and the quality of seeds (Egli et al. Citation2010). Equally important, however, is the impact of agrotechnical measures that shape environmental conditions, of which soil temperature and moisture (Wang et al. Citation2009; Schillinger et al. Citation2017) are particularly important. These conditions depend to a large extent on method of soil tillage and seed sowing (Celik et al. Citation2007). Altikat and Celik (Citation2011) showed that soil tillage and seed sowing affected the size of seedbed aggregates, mean weight diameter, soil moisture and temperature, cone index, and bulk density. In turn, soil properties significantly affected wheat emergence percentage and emergence time. Reduced tillage had a higher percentage of emergence and a shorter time of emergence than conventional tillage. However, the impact of soil tillage and seed sowing on soil properties and plant emergence differed between the seasons studied.
Weather conditions throughout the growing season affected the spatial variability of plant traits and of grain quality in wheat as well as the plants’ reaction to the tillage system (Diacono et al. Citation2012). In Evans et al. (Citation2010) several-year study, strip-till had higher grain yield than conventional tillage only in one year. In this year, strip-till improved the conditions on the soil surface, thereby limiting plant damage by weather factors (that is, soil erosion due to wind). In the present research, strip-till increased plant density after emergence also only in the year in which the soil during the sowing and emergence period was dry. In a year abundant in precipitation and before winter, when the soil is more moisture, strip-till has not had a positive effect on plant density ( and ). In the second year, however, strip-till resulted in the better wintering of barley plants, which in turn increased plant density (in the spring – ) and spike density (). The strip-till technology applied in the research used the one-pass strip tillage drill Pro-Til 4 T, which drops seeds into several-centimeter-wide furrows, helping water accumulate throughout the growing season and snow accumulate throughout the winter; thus, the plants have good conditions for development. This hypothesis is supported by the data in and . It was likely due to the improvement of conditions for plant development – mainly water availability – that in both years strip-till increased grain yield and reduced its and grain weight per spike spatial variability.
It is confirmed by a significant correlation between the soil moisture evaluated before sowing and the grain yield in the dry year as shown in . However, the correlation was lower when barley was grown following the strip-till technology. It can point to the ‘buffering’ effect of that technology on the soil properties. A simultaneous tillage, fertilisation and sowing as well as preceding crop post-harvest plant residue, especially when exposed to low precipitation and high temperature, limit the water loss and soil structure degradation.
The area where the experiment was conducted has a small average annual rainfall, about 500 mm. In the first year of the research, the annual rainfall was even 30% lower (). It is under such conditions that many traits of winter barley grown following the strip-till technology were more even and less correlated with the soil properties than in the conventional tillage system. According to Haramoto and Brainard (Citation2017), strip-till and the plant residues it leaves on the soil surface reduce the spatial and temporal variability of plant emergence especially in dry conditions. In such conditions, strip-till also increases nutrient uptake, thereby improving the growth and yielding of plants (Fernandez and White Citation2012). Overly simplified tillage, such as no-till, may aggravate the emergence of plants and reduce their density and uniformity within a field (Barut and Celik Citation2010). Strip-till, as one of the ways of soil functional zone management (Williams et al. Citation2016), combines the advantages of deep soil-loosening tillage, no-tillage, and direct sowing.
As a conclusion of the performed experiments, it can be said that each element of plant management that reduces spatial variability of plants is important for agricultural science and practice. The research indicates that strip-till – in which one pass of a machine makes it possible to cultivate the soil, apply fertilisers, and sow seeds – can be such an important element. A large amount of plant residues on soil surface along with soil functional zone management can help decrease the variability of soil and microclimate properties, creating more uniform conditions for plant growth. This hypothesis has been proven, to a great extent, by the results of this research: the spatial variability of plants was reduced, mainly in unfavourable environmental conditions, such as limited rainfall and low winter temperatures. Own results recorded in the year with higher precipitation, especially found in the scientific literature, point to a favourable effect of the strip-till technology on the soil properties and crop yielding also when exposed to high precipitation, e.g. by decreasing the surface runoff, limiting erosion, a fast water infiltration.
Acknowledgements
The authors thank the company, Agro-Land Marek Różniak in Śmielin, Poland, for allowing experimentation in their production field with the use of the strip-till drill by Mzuri Limited.
Disclosure statement
No potential conflict of interest was reported by the authors.
Notes on contributors
Iwona Jaskulska is an Associate Professor in the department of agronomy at the University of Science and Technology in Bydgoszcz. Her research focuses on elements of modern agrotechnical measure, including simplified tillage. Currently, she is researching on the environmental and economic conditions of strip-till technology.
Dr Anna Gałązka is the head of the Department of Agricultural Microbiology at the Institute of Soil Science and Plant Cultivation - State Research Institute Pulawy, Poland. She has specialised in the evaluation of microbial diversity of soils associated with agricultural practice and environmental protection.
Dariusz Jaskulski is a Professor in the field of agricultural sciences. He works in the department of agronomy at the University of Science and Technology in Bydgoszcz, Poland. He is the author or co-author of over 100 scientific and popular science papers in the field of agronomy, including the methods of soil cultivation and their impact on soil properties, plant growth and yield, and the economic and organisational effects of field crop production.
References
- Al-Kaisi MM, Douelle A, Kwaw-Mensah D. 2014. Soil microaggregate and macroaggregate decay over time and soil carbon change as influenced by different tillage systems. J Soil Water Conserv. 69:574–580. doi: 10.2489/jswc.69.6.574
- Allaire SE, Cambouris AN, Lafond JA, Lange SF, Pelletier B, Dutilleul P. 2014. Spatial variability of potato tuber yield and plant nitrogen uptake related to soil properties. Agron J. 106:851–859. doi: 10.2134/agronj13.0468
- Altikat S, Celik A. 2011. The effects of tillage and intra-row compaction on seedbed properties and red lentil emergence under dry land conditions. Soil Till Res. 114:1–8. doi: 10.1016/j.still.2011.03.003
- Alvarez R, Steinbach HS. 2009. A review of the effects of tillage systems on some soil physical properties, water content, nitrate availability and crops yield in the Argentine Pampas. Soil Till Res. 104:1–15. doi: 10.1016/j.still.2009.02.005
- Ambika S, Manonmani V, Somasundaram G. 2014. Review on effect of seed size on seedling vigour and seed yield. Res J Seed Sci. 7:31–38. doi: 10.3923/rjss.2014.31.38
- Andrade FH, Abbate PE. 2005. Response of maize and soybean to variability in stand uniformity. Agron J. 97:1263–1269. doi: 10.2134/agronj2005.0006
- Ayoubi S, Khormali F, Sahrawat KL. 2009. Relationships of barley biomass and grain yields to soil properties within a field in the arid region: use of factor analysis. Acta Agric Scand Section B–S–Plant. 59:107–117.
- Bannari A, Staenz K, Champagne C, Khurshid KS. 2015. Spatial variability mapping of crop residue using Hyperion (EO-1) hyperspectral data. Remote Sens. 7:8107–8127. doi: 10.3390/rs70608107
- Barthélémy D, Caraglio Y. 2007. Plant architecture: a dynamic, multilevel and comprehensive approach of plant form, structure and ontogeny. Ann Bot. 99:375–407. doi: 10.1093/aob/mcl260
- Barut ZB, Celik I. 2010. Different tillage systems affect plant emergence, stand establishment and yield in wheat-corn rotation. Philipp Agric Sci. 93:392–398.
- Brocca L, Melone F, Moramarco T, Morbidelli R. 2010. Spatial-temporal variability of soil moisture and its estimation across scales. Water Resour Res. 46:W02516. doi: 10.1029/2009WR008016
- Busari MA, Kukal SS, Kaur A, Bhatt R, Dulazi AA. 2015. Conservation tillage impacts on soil, crop and the environment. Int Soil Water Conserv Res. 3:119–129. doi: 10.1016/j.iswcr.2015.05.002
- Celik A, Ozturk I, Way TR. 2007. Effects of various planters on emergence and seed distribution uniformity of sunflower. Appl Eng Agric. 23:57–61. doi: 10.13031/2013.22331
- Cortés-D DL, Camacho-Tamayo JH, Giraldo R. 2016. Spatial prediction of soil penetration resistance using functional geostatistics. Sci Agric. 73:455–461. doi: 10.1590/0103-9016-2015-0113
- Cruz JS, de Assis Júnior RN, Matias RSS, Camacho-Tamayo JH. 2011. Spatial variability of an Alfisol cultivated with sugarcane. Cien Inv Agr. 38:155–164. doi: 10.4067/S0718-16202011000100015
- Delbari M, Afrasiab P, Loiskandl W. 2011. Geostatistic analysis of soil texture fractions on the field scale. Soil Water Res. 6:173–189. doi: 10.17221/9/2010-SWR
- Derpsch R, Friedrich T, Kassam A, Hongwen L. 2010. Current status of adoption of no-till farming in the world and some of its main benefits. Int J Agric Biol Eng. 3:1–25.
- Diacono M, Castrignanò A, Troccoli A, De Benedetto D, Basso B, Rubino P. 2012. Spatial and temporal variability of wheat grain yield and quality in a Mediterranean environment: a multi-variate geostatistical approach. Field Crop Res. 131:49–62. doi: 10.1016/j.fcr.2012.03.004
- Egli D, Hamman B, Rucker M. 2010. Seed vigor and uniformity of seedling emergence in soybean. Seed Technol. 32:87–95.
- Evans RG, Stevens WB, Iversen WM. 2010. Development of strip tillage on sprinkler irrigated sugarbeet. Appl Eng Agric. 26:59–69. doi: 10.13031/2013.29476
- Fernandez FG, Sorensenb BA, Villamil MB. 2015. A comparison of soil properties after five years of no-till and strip-till. Agron J. 107:1339–1346. doi: 10.2134/agronj14.0549
- Fernandez FG, White C. 2012. No-till and strip-till corn production with broadcast and subsurface-band phosphorus and potassium. Agron J. 104:996–1005. doi: 10.2134/agronj2012.0064
- Gajda A, Czyż E, Dexter A. 2016. Effects of long-term use of different farming systems on some physical, chemical and microbiological parameters of soil quality. Int Agrophys. 30:165–172. doi: 10.1515/intag-2015-0081
- Gałka B, Kabała C, Karczewska A, Sowiński J. 2016. Variability of soil properties in an intensively cultivated experimental field. Soil Sci Annu. 67:10–16. doi: 10.1515/ssa-2016-0002
- Gan Y, Stobbe EH, Moes J. 1992. Relative date of wheat seedling emergence and its impact on grain yield. Crop Sci. 32:1275–1281. doi: 10.2135/cropsci1992.0011183X003200050042x
- Gaston LA, Locke MA, Zablotowicz RM, Reddy KN. 2001. Spatial variability of soil properties and weed population in the Mississippi delta. Soil Sci Soc Am J. 65:449–459. doi: 10.2136/sssaj2001.652449x
- Gołaszewski J, Załuski D, Stawiana-Kosiorek A, Zaręba A. 2008. The usefulness of some soil properties and plant traits for the estimation of spatial variation in the 3^5 field experiment with pea (Pisum sativum L. sensu lato). EJPAU. 12(2):1–16.
- Haramoto ER, Brainard DC. 2017. Spatial and temporal variability in Powell amaranth emergence under strip-tillage with cover crop residue. Weed Sci. 65:151–163. doi: 10.1614/WS-D-16-00082.1
- He J, Li HW, Kuhn NJ, Wang QJ, Zhang XM. 2010. Effect of ridge tillage, no-tillage, and conventional tillage on soil temperature, water use, and crop performance in cold and semi-arid areas in Northeast China. Aust J Soil Res. 48:737–744. doi: 10.1071/SR09155
- Hossain MI, Gathala MK, Tiwari TP, Hossain MS. 2014. Strip tillage seeding technique: A better option for utilizing residual soil rainfed moisture stress environments of North-West Bangladesh. Int J Recent Dev Eng Technol. 2:132–136.
- Huang SB, Gao YB, Li YB, Xu LN, Tao HB, Wang P. 2017. Influence of plant architecture on maize physiology and yield in the Heilonggang River valley. Crop J. 5:52–62. doi: 10.1016/j.cj.2016.06.018
- Iqbal J, Thomasson JA, Jenkins JN, Owens PR, Whisler FD. 2005. Spatial variability analysis of soil physical properties of alluvial soils. Soil Sci Soc Am J. 69:1338–1350. doi: 10.2136/sssaj2004.0154
- Jabro JD, Iversen WM, Stevens WB, Evans RG, Mikha MM, Allen BL. 2016. Physical and hydraulic properties of a sandy loam soil under zero, shallow and deep tillage practices. Soil Till Res. 159:67–72. doi: 10.1016/j.still.2016.02.002
- Jankowski M, Przewoźna B, Bednarek R. 2011. Topographical inversion of sandy soils due to local conditions in Northern Poland. Geomorphology. 135:277–283. doi: 10.1016/j.geomorph.2011.02.005
- Karayel D, Özmerzi A. 2002. Effect of tillage methods on sowing uniformity of maize. Can Biosyst Eng. 44:23–26.
- Lavezo A, Filho AC, de Bem CM, Burin C, Kleinpaul JA, Pezzini RV. 2017. Plot size and number of replications to evaluate the grain yield in oat cultivars. Bragantia. 76:512–520. doi: 10.1590/1678-4499.2016.410
- Lemtiri A, Degrune F, Barbieux S, Hiel MP, Chélin M, Parvin N, Vandenbol M, Francis F, Colinet G. 2016. Crop residue management in arable cropping systems under temperate climate. Part 1: soil biological and chemical (phosphorus and nitrogen) properties. A review. Biotechnol Agron Soc Environ. 20:236–244.
- Makumburage GB, Stapleton AE. 2011. Phenotype uniformity in combined-stress environments has a different genetic architecture than in single-stress treatments. Front Plant Sci. 2:12. doi:10.3389/fpls.2011.00012.
- Martin JP. 1950. Use of acid, rose bengal and streptomycin in the plate method for estimating soil fungi. Soil Sci. 69:215–232. doi: 10.1097/00010694-195003000-00006
- Millán H, Tarquís AM, Pérez LD, Mato J, González-Posada M. 2012. Spatial variability patterns of some Vertisol properties at a field scale using standardized data. Soil Till Res. 120:76–84. doi: 10.1016/j.still.2011.11.003
- Morris NL, Miller PCH, Orson JH, Froud-Williams RJ. 2010. The adoption of noninversion tillage systems in the United Kingdom and the agronomic impact on soil, crops and the environment - A review. Soil Till Res. 108:1–15. doi: 10.1016/j.still.2010.03.004
- Olsen J, Kristensen L, Weiner J. 2006. Influence of sowing density and spatial pattern of spring wheat (Triticum aestivum) on the suppression of different weed species. Weed Biol Manag. 6:165–173. doi: 10.1111/j.1445-6664.2006.00210.x
- Olsen J, Weiner J. 2007. The influence of Triticum aestivum density, sowing pattern and nitrogen fertilization on leaf area index and its spatial variation. Basic Appl Ecol. 8:252–257. doi: 10.1016/j.baae.2006.03.013
- Overstreet LF. 2009. Strip tillage for sugarbeet production. Int Sugar J. 111:292–304.
- Patel JB, Bhatiya VJ, Babariya CA, Sondarva J. 2016. Effect of seed size on seedling vigour, plant growth, seed yield and its parameters: A review. Res Environ Life Sci. 9:859–864.
- Peel MC, Finlayson BL, McMahon TA. 2007. Updated world map of the Köppen-Geiger climate classification. Hydrol Earth Syst Sci. 11:1633–1644. doi: 10.5194/hess-11-1633-2007
- Perez-Quezada JF, Cavero J, Williams J, Roel A, Plant RE. 2003. Simulation of within field yield variability in a four-crop rotation field using SURRGO soil-unit definitions and the EPIC model. Trans ASAE. 46(5):1365–1374.
- Piotrowska A, Długosz J, Namysłowska-Wilczyńska B, Zamorski R. 2011. Field-scale variability of topsoil dehydrogenase and cellulase activities as affected by variability of some physico-chemical properties. Biol Fertil Soils. 47:101–109. doi: 10.1007/s00374-010-0507-3
- Rabbi S, Roy B, Miah M, Amin M, Khandakar T. 2014. Spatial variability of physical soil quality index of an agricultural field. Appl Environ Soil Sci. ID. 379012:1–10.
- Reyniers M, Maertens K, Vrindts E, de Baerdemaeker J. 2006. Yield variability related to landscape properties of a loamy soil in central Belgium. Soil Till Res. 88:262–273. doi: 10.1016/j.still.2005.06.005
- Sándor R, Lichner L, Filep T, Balog K, Lehoczky É, Fodor N. 2015. Spatial variability of hydrophysical properties of fallow sandy soils. Biologia. 70:1468–1473. doi: 10.1515/biolog-2015-0182
- Sapkota TB. 2012. Conservation tillage impact on soil aggregation, organic matter turnover and biodiversity. In: Lichtfouse E, editor. Organic fertilization, soil quality and human health. 9 Sustainable agriculture reviews. Dordrecht: Springer; p. 141–160.
- Schillinger WF, Schofstoll SE, Smith TA, Jacobsen JA. 2017. Laboratory method to evaluate wheat seedling emergence from deep planting depths. Agron J. 109:2004–2010. doi: 10.2134/agronj2016.12.0715
- Song X, Yang G, Yang C, Wang J, Cui B. 2017. Spatial variability analysis of within-field winter wheat nitrogen and grain quality using canopy fluorescence sensor measurements. Remote Sens. 9:237. doi:10.3390/rs9030237.
- Tivoli B, Calonnec A, Richard B, Ney B, Andrivon D. 2013. Current knowledge on plant/canopy architectural traits that reduce the expression and development of epidemics. Eur J Plant Pathol. 135:471–478. doi: 10.1007/s10658-012-0066-6
- Übelhör A, Witte I, Billen N, Gruber S, Hermann W, Morhard J, Claupein W. 2014. Feasibility of strip-tillage for field grown vegetables. J Cult Plants. 66:365–377.
- Usowicz B, Lipiec J. 2017. Spatial variability of soil properties and cereal yield in a cultivated field on sandy soil. Soil Till Res. 174:241–250. doi: 10.1016/j.still.2017.07.015
- Vieira SR, Paz Gonzalez A. 2003. Analysis of the spatial variability of crop yield and soil properties in small agricultural plots. Bragantia. 62:127–138. doi: 10.1590/S0006-87052003000100016
- Wallace R, Lockhead A. 1950. Qualitative studies of soil microorganisms. Amino acid requirements of rhizosphere bacteria. Can J Res. 28c:1–6. doi: 10.1139/cjr50c-001
- Wang H, Cutforth H, McCaig T, McLeod G, Brandt K, Lemke R, Goddard T, Sprout C. 2009. Predicting the time to 50% seedling emergence in wheat using a Beta model. NJAS-Wagen J Life Sci. 57:65–71. doi: 10.1016/j.njas.2009.07.003
- Willenborg CJ, Wildeman JC, Miller AK, Rossnagel BG, Shirtliffe SJ. 2005. Oat germination characteristics differ among genotypes, seed sizes and osmotic potentials. Crop Sci. 45:2023–2029. doi: 10.2135/cropsci2004.0722
- Williams A, Kane DA, Ewing PM, Atwood LW, Jilling A, Li M, Lou Y, Davis AS, Grandy AS, Huerd SC, et al. 2016. Soil functional zone management: a vehicle for enhancing production and soil ecosystem services in row-crop agroecosystems. Front Plant Sci. 7(65):1–15.
- WRB 2014 - IUSS Working Group WRB. 2014. World reference base for soil resources, 2014. International soil classification system for naming soils and creating legends for soil maps. Rome: FAO. World Soil Resources Reports No. 106. ISBN 978-92-5-108369-7.