ABSTRACT
A completely randomized factorial experiment was conducted to evaluate the growth and biomass production of single- and mixed crop species inoculated with mycorrhizae. Radish, (R), safflower (SF), pearl-millet (PM), mustard (M), field peas ((FP), faba-bean (FB), common vetch (CV), and berseem clover (BC) and their blends as R-SF-PM-M, FP-FB-CV-BC, R-SF-PM-M-FP-FB-CV-BC, R-SF-M-FP-FB-CV-BC, and PM-FP-FB-CV-BC were planted in a pot culture. The mycorrhizal inoculum significantly influenced the cover crops growth and biomass production under sterilized soils than that of non-sterile soils. While the safflower produced the highest total biomass, the pearl millet and field peas produced the lowest total biomass. Generally, in sterile and mycorrhizal conditions plant grown better and have high total C fixation. Within non-sterile and mycorrhizal inoculation, Safflower (1383.1 g C m−2) and Berseem Clover (1235.5 g C m−2) as a single species have fixed highest amount of carbon as biomass. Mycorrhizae inoculated BC+CV+FB+FP+SF+PM blends had a return of 1365.0 g C m−2 in non-sterile soil. Our results suggested that cover crops in single or mixed stands with mycorrhizal inoculations are expected to provide a significantly greater amount of biomass, which would contribute to soil organic matter and nutrient recycling for crop production.
Introduction
Cover crops as a biological primer influence soil health and crop yields by supplying essential nutrients, increase biodiversity and efficiency by providing labile and diverse organic C compounds, and improve physical stability (Mbuthia et al. Citation2015). Also cover crop influence the soil quality by supporting microbial diversity, providing organic matter, and recycling organically-bound nutrients for plant growth as well. Cover crops fix atmospheric CO2 and subsequently translate into soil organic matter (SOM) via biological metabolism and chemical polymerism (Piccoli et al. Citation2016). Legumes as cover crops often contribute soil N fertility through biological N fixation (Barea et al. Citation2011). Mahama et al. (Citation2016) reported that including legumines in cropping systems has the potential to improve the N availability and crop yield with a substantial reduction in chemical N fertilisation. de Sousa et al. (Citation2017) indicated that cover crops positively influenced the soil P and K availability to plants. Furthermore, integrating cover crops with cropping diversity has several benefits on agroecosystem services, such as improved soil health (Brennan and Acosta-Martinez Citation2017), mulching effect and erosion control, effective nutrient recycling (Kirchmann and Thorvaldsson Citation2000), suppress weed and pests, and contribute to carbon sequestration (Dabney et al. Citation2001).
Several studies have suggested that for sustainable agroecosystems, the effects of plant species diversity on soil biological functionality especially microbial diversity and efficiency is important (Barel et al. Citation2018; Lehman et al. Citation2019; Schmidt et al. Citation2019). While cover cropping is one of the components of sustainable agriculture, the importance of cover crops can better elucidate the role of soil organisms in nutrient cycles and plant nutrition as well. (Detheridge et al. Citation2016) reported that a diverse quality of carbon compounds released by and from cover crops synergistically influenced soil microbes. Likewise, Brennan and Acosta-Martinez (Citation2017) shown that cover crops influenced the abundance of pseudomonas and agromyces bacterial genera.
It is expected that cover crops when inoculated with mycorrhizae may contribute greater nutrient availability in the non-mycorrhizal rhizosphere. In degraded or low fertility soils, mycorrhizae often exert substantial contribution to plant nutrient availability and uptake. Turrini et al. (Citation2017) reported that continuous living cover is one of the best management practices to preserve and/or enhance soil biology and soil fertility. With balanced nutrient availability, mycorrhizal inoculation has shown to have positive effects on plant growth with increased photosynthetic activity (Cho et al. Citation2009). Also it has been shown that the residual effects of cover crops influenced mycorrhiza formation, uptake of P, Zn, and Cu, and early growth of leek seedlings (Sorensen et al. Citation2005). The results of Perez-Luna et al. (Citation2012) shown that cover crops has positive effects on mycorrhizal growth and diversity. Mycorrhizal diversity is one of the critical components to regulate soil fertility in support of crop growth. The indigenous AM fungus populations in intercropped and cover cropped fields reduced the need for chemical fertilisation especially P inputs (Elbon and Whalen Citation2015). Garland et al. (Citation2011) showed that cover crops are a viable strategy to increase the effectiveness of native populations of AM fungi in soil. The results of Capo-Bauca et al. (Citation2019) indicated that cover soil can present double of spores of arbuscular mycorrhizal fungi. Similarly, Rosner et al. (Citation2018) and Cruz et al. (Citation2020) reported that cover cropping enhances the abundance of AMF in the soil and affects the succeeding crop yields. However, limited research information is available on the mycorrhizal influence on growth and biomass production of cover crops when grown in single species or mixed species stands.
We hypothesised that mycorrhizal inoculation of growing media with its synergistic effect is expected to promote growth and biomass production on single- or mixed cover crops when integrated in cropping systems. The objective of our study was to evaluate the growth and biomass production of single- and blends of cover crop species with- and without mycorrhizal inoculations under the sterile and non-sterile clay loam media and their suitability to contribute nutrient-rich biomass under the Mediterranean semi-arid conditions.
Material and methods
Material
Plant Material: Radish (Brassica rapa), safflower (Carthamus tinctorius L.), pearl-millet (Pennisetum americanum L), mustard (Sinapis alba), field peas (Pisum sativum L.), faba-bean (Vicia faba L.), common vetch (Vicia sativa L.), and berseem clover (Trifolium alexandrinum L.) were used as plant materials ().
Table 1. Description of experimental treatments.
A greenhouse experiment was conducted at the Dept. of Soil Science and Plant Nutrition, Çukurova University in Adana, Turkey, with single- and mixed stands of eight different types of cover crops with- and without mycorrhizal inoculations in sterile- and non-sterile growing media ().
Table 2. Some properties of sterile and non-sterile soils.
Methods
A portion of the 2-mm sieved partially air-dried soil was autoclaved at 121 ± 3°C for 2 h. and used as a sterile media for growing cover crops. Exactly, two kg of sterile- and non-sterile soil was taken in separate plastic pots (dia. 17-cm and 12-cm tall), re-moistened at field-moisture capacity (∼0.33 cbar). While a random sample of about 500 spores (indigenous mycorrhiza inoculum) per pot was mixed to the media for mycorrhizal treatments, same number of non-mycorrhizal growth medium was added to the control treatments. The experiment was set-up by following completely randomised design in 2 × 2 × 8 factorial combinations with each treatment replicated four times ().
All treatments were planted in a pot culture the 2nd week of November 2014. The cover crops were irrigated thrice a week. At maximum vegetative growth stage, cover crops were harvested during February and March 2015, and the fresh shoot and root biomass were separated and collected in paper bags.
A random sub-sample of fresh root was collected, washed with distilled water and stained to determine mycorrhizae colonisation (Koske and Gemma Citation1989). The percentage of mycorrhizal colonisation was calculated as the number of segments infected out of the 100 segments that were examined under a stereo microscope at 20× magnification (Giovannetti and Mosse Citation1980).
The remaining portion of the roots were washed with distilled water and oven-dried at 550C until a constant weight was obtained. Similarly, the fresh shoot biomass was oven-dried. Oven-dried shoot and root materials were ground in a Wiley-mill and passed through a 125 µm sieve prior to chemical analysis. Shoot and root C concentration was determined with a CE Elentec® automated dry combustion analyzer. The datum of total dry biomass for each species was multiplied with the respective C concentration to calculate for total biomass C contents. A random sample of finely-ground plant materials was placed in a ceramic cup and heated at 550°C for 4 h. to calculate for ash contents. Botanical composition was calculated by dividing the individual cover crop dry-weight with the total biomass weight.
Statistical analysis
Significant differences in data on growth and biomass production and C contribution attributed to the effects of mycorrhizae inoculations, growth media, and type of cover crops were evaluated by three-way ANOVA using the SAS (SAS Citation2009). All independent factors were considered as fixed effects. Main and interactive effects of treatment combinations were separated by the Least Significant Difference (LSD) test with a value of p ≤ 0.05 unless otherwise mentioned. At harvest, cover crops were separated plant by plant and each plant species was recorded separately and percentages of species for single and mixture were calculated for cover crops botanical composition by using Minitab 17 computer program.
Results
Mycorrhizae inoculations and cover crops root colonisation
Cover crops significantly responded to mycorrhizal inoculations except for radish (R) and mustard (M) in sterile soil. Under non-sterile soils, there is a nearly equal root colonisation for both non-mycorrhizal (38%) and mycorrhizal inoculations (39%). However, under sterile soils (4%), only mycorrhizal inoculations significantly increased (38%) root colonisation (). In general, in sterile and mycorrhizal inoculated Field peas (69%), Berseem Clover (55%), Common vetch (54%) Faba beans (50%), and Safflower (45) had the highest root colonisation than that other cover crop treatments. The main effect and interaction of soil sterilisation and mycorrhizae statistically significantly affected root colonisation (). Check the data and justify what does this mean?
Figure 1. Mycorrhiza inoculated root ratio (%). Single Cover crop plant species grown under sterile and non-sterile soil conation and inoculated with mycorrhiza inoculation.
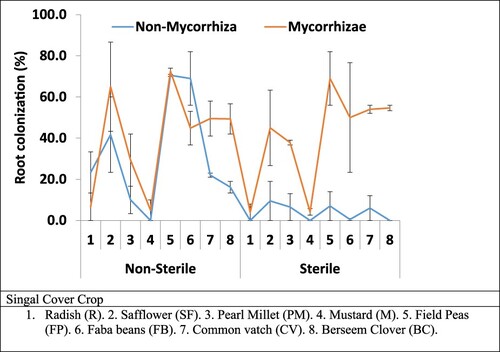
Table 3. Significant of F-values (probability) from analysis of variance for different plant parameters.
Cover crop blends have also higher root colonisation with mycorrhizal inoculations than that of non-mycorrhizal colonisation under both non-sterile and sterile soils. The Berseem Clover (BC) + Common vetch (CV)+ Faba beans + Field Peas + Mustard (M)+ Radish (R)+ Safflower + Pearl Millet (PM) blend has the highest root colonisation with mycorrhizal inoculation 57% for non-sterile and 48% for sterile soil when compared with other cover crop blends ().
Figure 2. Mycorrhiza inoculated root ratio (%). Mix Cover crop plant species grown under sterile and non-sterile soil conation and inoculated with mycorrhiza inoculation.
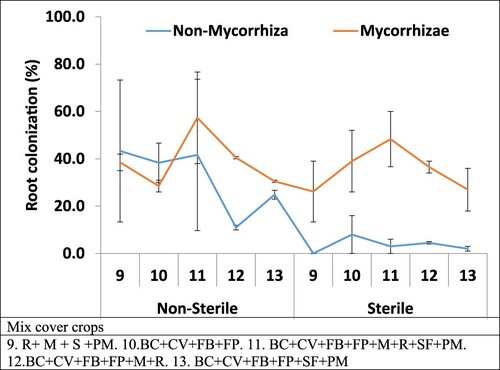
Cover crops biomass production and partition
Results showed that cover crops significantly responded to soil conditions and mycorrhizal inoculations (). Averaged across cover crops, non-sterile soil (control) has produced highest total biomass as compared to sterile soil. Moreover, mycorrhiza inoculated sterile soils had the highest cover crop total biomass than the non-mycorrhizal inoculated soils. Safflower and Berseem Clover were the individual cover crop species produced highest total biomass as compared to other individual cover crop species. Total biomass production of non-sterile and non-mycorrhizal inoculated Berseem Clover (BC) produced 2254.9 g m2 and mycorrhizal inoculated BC produced 2504.5 g m2. In sterile and non-mycorrhizal plant BC produced 1922.5 g m2 and sterile and mycorrhizae inoculated plant produced 2188.6 g m2. Mycorrhiza inoculated Safflower produced 2.112.4 g m−2 in non-sterile soils and 2.713.7 g m−2 in sterile soils. In contrast, Field Peas (FP) and Pearl Millet (PM) did not performed well in both non-sterile and non-mycorrhizae inoculated treatments. PM and FP plants produced less total dry weight compared with other treatments (). Since the experiment was set up during winter season, pearl millet and field pea as a summer growing plants were not grown properly. That’s way they have less yield and biomass.
Table 4. Single and mix cover crops shoot root dry weight and total dry weight g m2 was determined under sterile and non-sterile soil with and without mycorrhizal inoculation.
Combination of cover crop blends also varied with mycorrhizal inoculations. Cover crop blends with mycorrhizal inoculations have performed better than the control. The non-sterile soils have produced highest total biomass than cover crops grown in sterile soils. In mycorrhiza inoculated sterile soils, total biomass was higher than the non-mycorrhizal inoculated ones. In non-sterile and mycorrhizal inoculated soils BC + CV + FB + FP + SF + PM blend produced 2706.5 gm−2 and with soil sterilisation and mycorrhizal inoculation produced 2372.6 gm−2 total dry biomass than other cover crops.
Cover crops botanical composition
Results presented in showed that under non-sterile soils mycorrhiza inoculated mix of BC + CV + FB + FP + SF + PM treatment botanical composition of Berseem Clover (BC) %, for Common Vetch (CV), Safflower (SF) and Faba Beans (FB) is higher (by 40.48, 30.35, 18.64 and 10.53% respectively) than Pearl Millet (PM) (0.0%) and Field peas (FP) (0.0%). Under sterile soils, mycorrhiza inoculated mix of BC + CV + FB + FP + SF + PM treatment BC 17.62%, CV 33.19%, FB 8.93%, FP 16.17 and SF 24.10% botanical composition was determined. Usually under mycorrhizae planted conditions botanical composition was significantly higher for CV by 56.41%, BC 27.81% SF 24.10% than other treatments. The CV and BC plants were dominant in the mix cultivation with highest percentages composition. Based on the results we expect that these cover crops can be used in our semi-arid soil conditions.
Table 5. Botanical composition. Botanical composition was calculated on base of each species dry weight in composition.
Cover crops biomass carbon content and contribution
As can be seen in , in generally mycorrhiza inoculated mix plant have high C concentrations. While the control PM shoot biomass produced in non-sterile soil has 51.3% of C, the highest shoot C% was obtained in FB by accounting 53.3%, which were significant different. Mycorrhiza inoculated FP had highest shoot C concentration (51.8%) among the cover crops. Under non-sterile and non-mycorrhizal conditions BC + CV + FB + FP + SF + PM mix cover crops have high 4.37% C and under non-sterile and mycorrhizal inoculation 50.66% of C concentration was measured.
Table 6. Single and mix cover crops shoot and root % C and C fixation.
Root carbon concentration was measured and usually under non-sterile and mycorrhizal conditions with SF by % 53.87 C was measured. Under sterile and non-mycorrhizal soil conditions Radish plant have higher C concentration % 52.24 C (). In mix cover crops under non-sterile and non-mycorrhizal conditions R+ M + SF + PM mix crop composition have 53.51%.
Shoot and root C fixed in plant dry-mass (gr C m−2) have been calculated. In single plants, under non-sterile and sterile soil conditions, mycorrhiza inoculated crops had the highest for shoot C fixation was obtained 1237.8 g C m−2 with SF and 1129.1 g C m−2 with BC treatments than non-inoculated. However, in sterile soil with mycorrhizal inoculations the SF and BC fixed a total of 968.3 and 907.3 g C m−2, respectively. The BC grown in non-mycorrhiza inoculated and non-sterile conditions produced 1049.1 g C m−2 and in sterile non-mycorrhiza inoculated conditions the BC fixed 855 g C m−2. Under the control condition, BC + CV + FB + FP and BC + CV + FB + FP + M+R + SF + PM blends fixed higher amount of C than other treatments. However, mycorrhizae inoculated BC + CV + FB + FP + SF + PM blends fixed 1296.1 g C m−2 in non-sterile soil and 1122.5 g C m−2 under sterile soil.
The highest root C fix was obtained in sterile and mycorrhiza inoculated BC treatments obtained 200.7 g C m−2 and following these sterile and non-mycorrhizae treated SF cover crop treatments fixed 194.3 g C m−2. And mycorrhiza inoculation statistically significantly affected plant carbon fixation ().
Under mycorrhizal and sterile soil conditions, the BC + CV + FB + FP + M+R + SF + PM blends fixed higher amount of root C 184.2 g C m−2, with sterile and non-mycorrhizal inoculations, have 91.8 g C m−2 amount of C.
All cover crops treatments mean data was tested for dendrogram cluster analyses. It is clear non-mycorrhizal Radish, treatment number (TN) 1 and Mustard, TN 4 are very close to each other with 76.1 similarity. Pearl millet, TN 3 and Field pea TN 5 are less similar with 63.1. TN 2 and TN 11 (75.7 similarly), TN 6 and 7 (75.4 similarity) and TN 10 and TN 13 are (83.7 similarity). The highest similarity was found with TN10 (FP-FB-CV-BC) and TN 13 (PM-FP-FB-CV-BC).
Discussion
Results showed a significant positive effect exerted by mycorrhizae on cover crops either as single species or blends. Without mycorrhizal colonisation, the cover crops biomass production was decreased, which may be due to a lesser root colonisation. As nutrient content in sterile soil was relatively higher than in non-sterile soil, which may have reduced the colonisation of mycorrhizae and subsequently limit the cover crop growth in sterile soil (Jones and Smith Citation2004). As soil initial P was high, a higher input of C may have exerted negative effects on root colonisation. Moreover, non-sterile soil had indigenous soil organisms which may possibly have developed among native and introduced organisms. Mycorrhizal inoculation with soil sterilisation had a significant interaction on shoot and root biomass of cover crops. Since with soil sterilisation indigenous soil organisms including viable mycorrhiza species were eliminated, plant roots did not enter into food competition with other microorganisms and consequancely with re-inoculation plant grown better. Under non-sterile soil conditions, the effect of mycorrhizal inoculation was significantly less than the sterile soil conditions due to competition with indigenous spores. (Ortas and Ustuner Citation2014) reported that there is a natural competition between exotic and indigenous mycorrhizal spores. In another study, it is reported that under Indian mustard, hairy vetch, and a mixture of seven cover crops mycorrhizal species richness significantly increased (Njeru et al. Citation2015).
Mycorrhizae inoculation under sterile soil conditions increased plant biomass production, which may be due to less competition for food, space, and other inputs from indigenous species. Usually under non-sterile soil conditions soil organisms have negative effects on plant root growth and up to 20% of photosynthesis assimilate leaked to rhizosphere (Ortaş et al. Citation2016). Similarly Parniske (Citation2008) indicated that up to 20% of plant-fixed carbon by photosynthesis is transferred to the fungus. In many works, because of the sterilisation process, the effect of re-mycorrhizal inoculation plant growth is increased (Ortas Citation2015; Ortas et al. Citation2018). In present work, shown that mycorrhiza inoculated treatments cover crops treatments were better than non-inoculated treatments More C fixation is very important for mitigation of atmospheric CO2 which is directly related with climate changes.
Table 7. Total carbon fixation of single and mix cover crop under non-sterile and sterile soil conditions.
Positive effects of cover crops were attributed to the large amount of P incorporation, increase in the P-solubilising fungal population and/or biomass P in soil (Karasawa and Takahashi Citation2015). Similarity it has been indicated by Hallama et al. (Citation2019) cover cropping enhances soil microbial community abundance, activity, and in particular, benefits from increases in AMF abundance in soils with low available P. It has been reported that management of cover crops and potentially enhanced AMF colonisation may increase the availability of soil P pool by stimulating microbial activity and release of root exudates (Nelson and Janke Citation2007).
Mukherjee and Lal (Citation2015) reported that an improvement in soil properties is likely with an appropriate cover crop under short-term field experiment; however, long-term data are needed to evaluate whether or not these trends are transient or consistent. Mukherjee and Lal (Citation2015) and (de Sousa et al. Citation2017) indicated that biomass contribution from cover crops increased SOC contents. Further studies are needed to verify whether or not the mycorrhizal plant species have contribution or condition in between plant species. Forage radish is being used in many parts of the World as a winter cover crop to alleviate soil compaction, reduce nitrate leaching, suppress weeds and control erosion (Weil and Kremen Citation2007).
Cover crops blends have been shown that can successfully grow under such as semi-arid Mediterranean climate. It seems that those combinations have the advantage of fixing more carbon and increase biomass. Plants species and their mix grown cover crops may also have a greater effect on atmospheric carbon mitigation. Brennan and Acosta-Martinez (Citation2017) indicated that C inputs from frequent cover cropping are the primary driver of changes in the soil food web and soil and crop managements. Finally, our results are shown that several combination of cover corps can be used. PM-FP-FB-CV-BC combination and FP-FB-CV-BC can be suggested to be used in the semi-arid soil conditions.
In generally mycorrhiza inoculated plant produced highest total C fixation compare to non-inoculated plants. Usually under both sterile and non-sterile soil conditions mycorrhizal inoculated mix cover crops fixed highest C fixation. And all treatments were statistically significantly affected C fixation. It is very important to have such as cover crop can retain more carbon which is very important for carbon mitigation and climate change. With mycorrhize inoculation Safflower and Berseem Clover single plant species have fixed highest carbon. Also mycorrhiza inoculated BC, CV, FB, FP, M, R and BC + CV + FB + FP + SF + PM mix cover crops fixed carbon. Mixed cover crops have shown a great advantage of sequestering carbon with increased increase biomass. Cover crops can be successfully raised under the semi-arid Mediterranean climate. It can be concluded that legume and non-legume cover crops mixture can be incorporated into existing crop rotation systems in Turkey for greater carbon retention, biomass mulching, and nutrient recycling, especially nitrogen to support for sustainable agricultural management practices.
Disclosure statement
No potential conflict of interest was reported by the author(s).
Additional information
Notes on contributors
Ibrahim Ortas
İbrahim Ortas is a Prof. Soil Science and Plant Nutrition, Agriculture Faculty, Cukurova University, Adana, Turkey. He is the main author for the current study.
Celal Yucel
Celal Yucel is a Prof. Dept. of Field Crops, Agriculture Faculty, Sirnak University, Şırnak, Turkey. He is a co-author for the current study.
References
- Barea JM, Palenzuela J, Cornejo P, Sanchez-Castro I, Navarro-Fernandez C, Lopez-Garcia A, Estrada B, Azcon R, Ferrol N, Azcon-Aguilar C. 2011. Ecological and functional roles of mycorrhizas in semi-arid ecosystems of Southeast Spain. J Arid Environ Dec. 75:1292–1301.
- Barel JM, Kuyper TW, de Boer W, Douma JC, De Deyn GB. 2018. Legacy effects of diversity in space and time driven by winter cover crop biomass and nitrogen concentration. J Appl Ecol. 55:299–310.
- Brennan EB, Acosta-Martinez V. 2017. Cover cropping frequency is the main driver of soil microbial changes during six years of organic vegetable production. Soil Biol Biochem. 109:188–204.
- Capo-Bauca S, Marques A, Llopis-Vidal N, Bota J, Baraza E. 2019. Long-term establishment of natural green cover provides agroecosystem services by improving soil quality in a Mediterranean vineyard. Ecol Eng. 127:285–291.
- Cho EJ, Lee DJ, Wee CD, Kim HL, Cheong YH, Cho JS, Sohn BK. 2009. Effects of AMF inoculation on growth of Panax ginseng CA Meyer seedlings and on soil structures in mycorrhizosphere. Sci Hortic. 122:633–637.
- Cruz AF, Pires MD, do Nascimento LKB, Ramos MLG, Oliveira SA, Blum LEB, Yamanishi OK. 2020. Cover cropping system and mulching can shape soil microbial status in fruit orchards. Scientia Agricola. 77:7.
- Dabney SM, Delgado JA, Reeves DW. 2001. Using winter cover crops to improve soil and water quality. Commun Soil Sci Plant Anal. 32:1221–1250.
- de Sousa DC, Medeiros JC, Dalla Rosa J, de Jesus JJ, Lacerda ÁLM. 2017. Chemical attributes of agricultural soil after the cultivation of cover crops. Aust J Crop Sci. 11:1497–1503.
- Detheridge AP, Brand G, Fychan R, Crotty FV, Sanderson R, Griffith GW, Marley CL. 2016. The legacy effect of cover crops on soil fungal populations in a cereal rotation. Agric Ecosyst Environ. 228:49–61.
- Elbon A, Whalen JK. 2015. Phosphorus supply to vegetable crops from arbuscular mycorrhizal fungi: a review. Biol Agric Hortic. 31:73–90.
- Garland BC, Schroeder-Moreno MS, Fernandez GE, Creamer NG. 2011. Influence of summer cover crops and mycorrhizal fungi on strawberry production in the Southeastern United States. Hortscience. 46:985–992.
- Giovannetti G, Mosse B. 1980. An evaluation of techniques for measuring vesicular-arbuscular mycorrhiza in roots. New Phytol. 84:489–500.
- Hallama M, Pekrun C, Lambers H, Kandeler E. 2019. Hidden miners – the roles of cover crops and soil microorganisms in phosphorus cycling through agroecosystems. Plant Soil. 434:7–45.
- Jones MD, Smith SE. 2004. Exploring functional definitions of mycorrhizas: are mycorrhizas always mutualisms? Can J Bot. 82:1089–1109.
- Karasawa T, Takahashi S. 2015. Introduction of various cover crop species to improve soil biological P parameters and P uptake of the following crops. Nutr Cycl Agroecosyst. 103:15–28.
- Kirchmann H, Thorvaldsson G. 2000. Challenging targets for future agriculture. Eur J Agron. 12:145–161.
- Koske RE, Gemma JN. 1989. A modified procedure for staining roots to detect VA-mycorrhizas. Mycol Res. 92:486–488.
- Lehman RM, Osborne SL, Taheri WI, Buyer JS, Chim BK. 2019. Comparative measurements of arbuscular mycorrhizal fungal responses to agricultural management practices. Mycorrhiza. 29:227–235.
- Mahama GY, Prasad PVV, Roozeboom KL, Nippert JB, Rice CW. 2016. Cover crops, fertilizer nitrogen rates, and economic return of grain sorghum. Agron J. 108:1–16.
- Mbuthia LW, Acosta-Martinez V, DeBruyn J, Schaeffer S, Tyler D, Odoi E, Mpheshea M, Walker F, Eash N. 2015. Long term tillage, cover crop, and fertilization effects on microbial community structure, activity: Implications for soil quality. Soil Biol Biochem. 89:24–34.
- Mukherjee A, Lal R. 2015. Short-term effects of cover cropping on the quality of a Typic Argiaquolls in Central Ohio. Catena. 131:125–129.
- Nelson NO, Janke RR. 2007. Phosphorus sources and management in organic production systems. Horttechnology. 17:442–454.
- Njeru EM, Avio L, Bocci G, Sbrana C, Turrini A, Barberi P, Giovannetti M, Oehl F. 2015. Contrasting effects of cover crops on ‘hot spot’ arbuscular mycorrhizal fungal communities in organic tomato. Biol Fertil Soils. 51:151–166.
- Ortas I. 2015. Comparative analyses of Turkey agricultural soils: potential communities of indigenous and exotic mycorrhiza species’ effect on maize (Zea mays L.) growth and nutrient uptakes. Eur J Soil Biol. 69:79–87.
- Ortas I, Demirbas A, Akpinar C. 2018. Under sterilized and non-sterilized soil conditions, mycorrhizal dependency in citrus plants depends on phosphorus fertilization rather than zinc application. Eur J Hortic Sci. 83:81–87.
- Ortas I, Ustuner O. 2014. The effects of single species, dual species and indigenous mycorrhiza inoculation on citrus growth and nutrient uptake. Eur J Soil Biol. 63:64–69.
- Ortaş I, Razzaghi S, Rafique M. 2016. Arbuscular mycorrhizae: effect of rhizosphere and relation with carbon Nutrition. In: D. Choudhary, A. Varma, N. Tuteja, editors. Plant-Microbe Interaction: An Approach to Sustainable Agriculture. Singapore: Springer; p. 125–152.
- Parniske M. 2008. Arbuscular mycorrhiza: the mother of plant root endosymbioses. Nat Rev Microbiol. 6:763–775.
- Perez-Luna YD, Alvarez-Solis JD, Mendoza-Vega J, Pat-Fernandez JM, Gomez-Alvarez R, Cuevas L. 2012. Diversity of arbuscular mycorrhizal fungi in maize with cover crops and biofertilizers in Chiapas, Mexico. Gayana Bot. 69:46–56.
- Piccoli I, Chiarini F, Carletti P, Furlan L, Lazzaro B, Nardi S, Berti A, Sartori L, Dalconi M, Morari F. 2016. Disentangling the effects of conservation agriculture practices on the vertical distribution of soil organic carbon. Evidence of poor carbon sequestration in North-Eastern Italy. Agric Ecosyst Environ. 230:68–78.
- Rosner K, Bodner G, Hage-Ahmed K, Steinkellner S. 2018. Long-term soil tillage and cover cropping affected arbuscular mycorrhizal fungi, nutrient concentrations, and yield in sunflower. Agron J. 110:2664–2672.
- SAS/STAT user’s guide. 2009. Version 8. Cary, NC: SAS Inst.
- Schmidt R, Mitchell J, Scow K. 2019. Cover cropping and no-till increase diversity and symbiotroph:saprotroph ratios of soil fungal communities. Soil Biol Biochem. 129:99–109.
- Sorensen J, Larsen J, Jakobsen I. 2005. Mycorrhiza formation and nutrient concentration in leeks (Allium porrum) in relation to previous crop and cover crop management on high P soils. Plant Soil. 273:101–114.
- Turrini A, Caruso G, Avio L, Gennai C, Palla M, Agnolucci M, Tomei PE, Giovannetti M, Gucci R. 2017. Protective green cover enhances soil respiration and native mycorrhizal potential compared with soil tillage in a high-density olive orchard in a long term study. Appl Soil Ecol. 116:70–78.
- Weil R, Kremen A. 2007. Thinking across and beyond disciplines to make cover crops pay. J Sci Food Agric. 87:551–557.