ABSTRACT
Nanoparticle (NP) use is becoming important alternative in different branches of science such as in agriculture, used as new source of fertiliser. In this study, it was tested iron (Fe) NP, applied as corn seed priming. In order to evaluate the early growth and development of the corn seedling a factorial experiment (5 × 3) was performed in a complete random way design with 4 replications. One of the factors were concentrations of (0, 20, 40, 80, 160 mg L−1) related to iron inside and the factor with three levels were sources (Fe3O4 NP, Fe3O4 bulk and FeCl3). 50 sterile seeds were the plot composition, which were soaked in the different Fe concentration solution containing a total volume of 200 mL for 8 h. Germination and biometric development were evaluated. Fe NPs promoted beneficial responses for seedling growth compared to other Fe sources tested here. It was realised that Fe3O4 NP concentration beneficial effects was lower compared to the other tested sources. Particle size had an important assignment in the bioavailability. The plant response reached in this research indicated iron NPs can consist efficiency for corn seed priming when applied between 50 and 70 mg L−1 concentration based on interpolation.
Introduction
Iron is one element considered essential for animals and plants nutrition (Martin et al. Citation1994). For plants, it is linked to photosynthesis oxidative reduction process and also is a constituent of haemoglobins and nitrogenase and participating in DNA synthesis and phytohormones production (Hell and Sthephan Citation2003). For humans, considerable amounts are needed daily, around 10–18 mg daily is recommended to prevent health problems (Claessens et al. Citation2013). Fe deficiency is one of the world’s leading causes of malnutrition, particularly in children (Olivares et al. Citation1999).
Corn is an important edible culture for humans and animals in Brazil and worldwide. Although Brazilian soils present on average Fe content in soils that may change from 10 to 100 mg kg−1 (Batista et al. Citation2018), which would be adequate to reach crop demand, Fe deficiency in soils occurs on more than 30% of Earth’s agricultural soils (Vose Citation1982). Some basic crops have shown promising results regarding seed priming such as corn (Harris et al. Citation2007), oats (Saric and Saciragic Citation1969) wheat (Marcar and Graham Citation1986) and bean (Duran et al. Citation2018). Likewise, using NP for agronomic purposes as seed priming fertilisers. Lal (Citation2008) is considered one of the most significant ways to increase crop yields, since their application efficiency is higher compared to commercial fertilisers which range from 30 to 50% of efficiency (Liu and Lal Citation2015).
The hypothesis was that Fe concentration and sources differing in size and solubility, when applied as seed priming, change corn seedlings early development. Therefore, it was reported the effects of nanomagnetite synthesised in an easy and green way on growth and germination of corn seeds. The effects of seed priming were observed on the germination rate, vigour, root and shoot elongation, root and shoot dry biomass production.
Materials and methods
Part of the research was carried out at Maringa, in the state of Parana, Brazil (23° 40ʹ 37ʺ South and 51° 94ʹ 08ʺ West) and part in Logan, in the State of Utah, USA (41° 74ʹ 27ʺ North and 111° 80ʹ 73ʺ West) between January and December 2019. Fe3O4 NPs were prepared primarily using the reaction precursors of 100 mL of FeSO4.7H2O 0.1 and 0.4 mol L−1 NaOH another 100 mL. The blends were done, and then gently admixed in an Erlenmeyer flask, the resulting solution was agitated energetically at 1500 rpm for 15 continuous minutes using a magnetic stirrer. Once mixed, the Erlenmeyer flask was put covered inside of microwave oven (700 W) and heated in time 2 min. After this procedure, the flask was cooled to room temperature and a black precipitate was formed. The remaining solution was washed many times using distilled water, to remove sulphate and sodium ions. The solution was placed in liquid nitrogen (−70°C) to freeze and dried lyophiliser Terroni® and then packed in a vacuum glass desiccator, thus protecting it from light.
The particles were characterised as mineral magnetite, according to the X-ray diffraction analysis (XRD 6000-Shimadzu) (). The X-ray diffractograms were achieved addressing XRD in 20° and 75° 2θ, in interval of 0.02° 2θ in time 0.6 s, using CoKα radiation and filter of nickel.
In order to ensure the magnetite are nanoparticles (NPs), transmission electron microscopy analysis (TEM) (Philips CM200) was performed to observe the shape and size of the synthetic NP. The samples were done by drop casting the NP suspension onto carbon–copper coated grids and were then allowed to dry overnight. The Fe3O4 NPs had a diameter around 30 nm and rounded shape ().
Figure 2. Transmission electron microscopy image of the synthetic iron oxide NP. Scale bar is 50 nm.
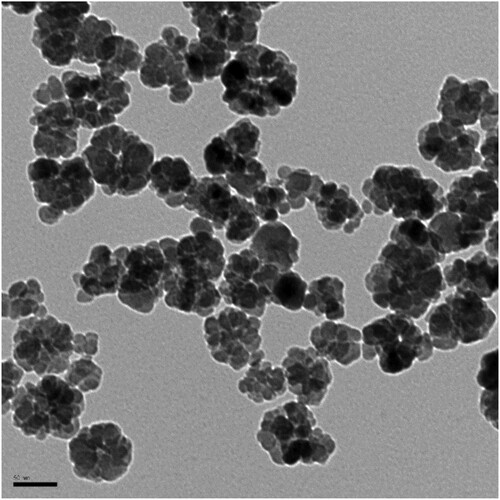
The hybrid IAC 8046 of corn seeds were donated by Sella Sementes, Astorga, Brazil. The seeds were uniformly selected in order to reduce seed variation characteristics. The chosen seeds were cleaned during 2 min with sodium hypochlorite (1%). Before the experiment, seeds were kept at a temperature of 16°C in a dry and dark site.
A factor experiment (5 × 3) was performed in complete random way with 4 replications. First factor were Fe concentrations (0, 20, 40, 80 and 160 mg L−1 stated on Fe quantities) and three level factor were the sources (Fe3O4 NPs, FeCl3, and Fe3O4 bulk). 50 seeds were considered the plot. The seeds were soaked in the different solutions with 200 mL of Fe concentrations during 8 h, after let the seeds dried during another hour.
The FeCl3 ionic solution was prepared by dissolving FeCl36H2O in distilled water. The other sources (Fe3O4 NPs and Fe3O4 bulk) were added in distilled water and spread for 5 min with ultrasonic vibration shaker. The source Fe3O4 at non-nanometric size >300 nm.
NP-primed and control corn seed germination was assessed on the ‘Germitest’ paper. For this, the primed corn seeds were transferred to 3 ‘Germitest’ moist sheet (2.5 times the paper dry mass). The seeds were placed and 1 cm apart. Later, seeds were placed to germinate in the Mangelsdorf chamber for 8 days and 25°C. The variables were expressed in percentage (%) of normal, abnormal and non-viable seedlings. The vigour test was carried out on the fifth day by the vigour test (Brasil Citation2009).
Fifteen seedlings were randomly selected to assess root length (distance from the stem base to the root tip) and shoot length (distance from the base of the leaf to the tip of the leaf). Dry biomass production was assessed by seedlings put seedlings in a paper bags and put in a drying oven set at 60°C for 72 h.
The obtained data were subjugated to statistical assumptions using normality of errors test (Shapiro–Wilk) and homogeneity of variances test (Bartelett) (p > 0.01). After that, concentrations, sources and interactions were tested by F test. Interaction was partitioned only for concentrations within source. Regression test was used to analyse Fe concentration and their coefficients by T test. While, sources were analysed by the Tukey test (p < 0.05) (Zimmermann Citation2014).
Results
Results obtained after the concentration partitioning within each tested Fe source (Fe3O4 NPs, Fe3O4 bulk and FeCl3) for the variables vigour, germination, non-viable and abnormal corn seeds are shown in . No statistical differences were found for germination, vigour, non-viable and abnormal seeds as well (p < 0.05). The general averages for germination, vigour, non-viable and abnormal seed were 84.1, 83.3 and 81.8%; and 78.6, 77.3 and 73.5%; and 7.4, 6.8 and 6.1%; and 10.8, 10.0 and 9.9% for FeCl3, Fe3O4 bulk and Fe3O4 NPs, respectively.
Figure 3. Corn germination parameters after Fe concentrations seed priming and sources. Note A: Seed germination. Note B: Seed vigour. Note C: Nom-viable seed. Note D: abnormal seed
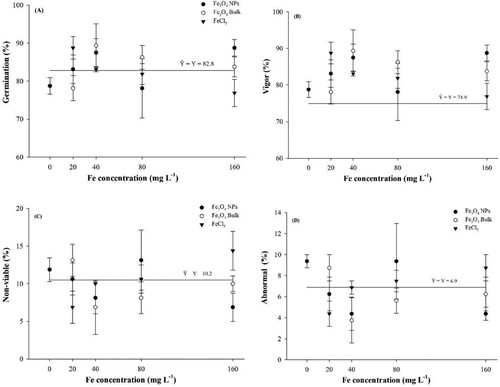
It was observed significant modification in shoot and root length with the Fe concentration increases ((A,B)). On the other hand, there were no significant modifications to shoot/root length ratio for Fe concentrations, although there were significant changes related to Fe sources ((C)). Shoot length data for Fe3O4 bulk and NPs were fitted in a quadratic model, where a maximum length (9.71 cm) of 53.0 mg L−1 Fe concentration for Fe3O4 NPs and 83.3 mg L−1 Fe concentration (10.3 cm) for Fe3O4 bulk was observed. The soluble source of Fe (FeCl3) had shoot length average of 9.0 cm.
Figure 4. Corn biomass length production after Fe concentrations seed priming and sources. Note A: Shoot dry length. Note B: Root length. Note C: shoot/root length ratio.
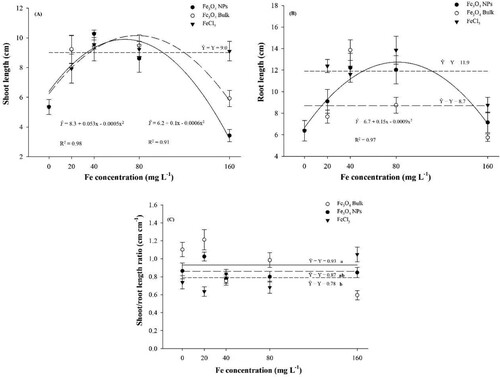
On the other hand, it was evident that there was inhibition of root growth, with the concentration increased, since for the higher concentration tested 160 mg L−1 the length was 3.98 cm, that is a reduction of seedling growth in 41%. Fe3O4 bulk concentrations altered shoot length quadratically as well, in which 83.3 mg L−1, promoted the higher shoot seedlings (10.3 cm), although at the concentration 160 mg L−1 shoot seedling decreased like NPs. The soluble source was not significant altered, and its average was 9.0 cm. Although the increments in corn shoot, length in the best Fe concentration using Fe3O4 NPs was not very large, the toxic effect was evident in highest concentration Fe3O4 NPs (160 mg L−1).
Root length results are shown in (B). It was found that only Fe3O4 NPs concentrations altered root length significantly and quadratic regression model was fitted. The concentration 83.3 mg L−1 promoted the higher root length (12.95 cm), on the other hand, it was evident that there was inhibition of root growth, with the concentration increased, since for the higher concentration tested 160 mg L−1 the length was 7.66 cm, that is a reduction of the seedling root in 40.9%. The other concentrations using the Fe3O4 bulk and FeCl3 did not significantly and averages were 11.1 and 8.6 cm, respectively.
Shoot/root length ratio ((C)) was not altered by Fe concentrations tested, although when compared just the sources in concentrations averages differences were significant. Fe3O4 NPs obtained higher ratio than Fe3O4 bulk, although did not differ from FeCl3 ratio.
show the effects of the Fe concentrations application for each source tested for evaluated dry biomass production variables. The concentrations affected significantly seedling shoot dry biomass production only for Fe3O4 NPs ((A)). The maximum shoot dry biomass observed (0.36 g) was obtained at 75.34 mg L−1. Nevertheless, it was evident that shoot dry biomass production decreased in higher concentrations, since for the higher concentration tested 160 mg L−1 the biomass production was 0.14 g that is a reduction of almost of 50%. Fe3O4 bulk and FeCl3 was not altered with treatment, and the averages were 0.25 and 0.28 g, respectively. Regarding root dry biomass production, Fe3O4 NPs had similar behaviour, obtaining the maximum biomass production (0.16) at 70.42 mg L−1, concentration ((B)). Fe3O4 bulk was not significant altered and its average was 0.15 g. Despite that, FeCl3 decrease root dry biomass production with the higher concentration. For each mg added in seed priming solution, root dry biomass production will decrease in 0.5 mg.
Figure 5. Corn dry biomass production after Fe concentrations seed priming and sources. Note A: Shoot dry biomass. Note B: Root dry biomass production. Note C: shoot/root dry biomass production ratio.
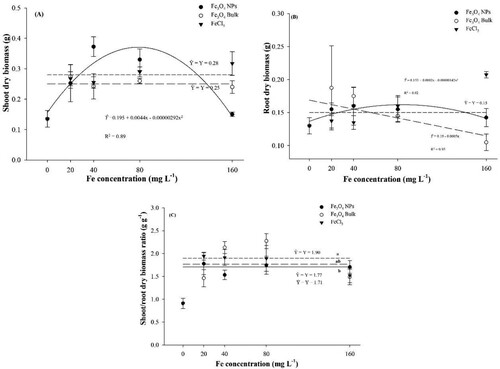
Shoot/root biomass ratio ((C)) was not altered by concentrations tested, although when compared just the sources in concentrations averages, differences were significant. FeCl3 obtained higher ratio than Fe3O4 NPs.
Discussion
Fe3O4 NPs corn seed priming is a pre-planting management that exposes seed determined Fe content. The Fe concentrations tested in these experiments were incapable in making seeds more vigourous and raise germination tests. Although, in the literature there are different reports showing that iron oxide may trigger different impacts on germination %, according to plant type, the Fe oxide polymorph, NPs morphology and size, and the NPs concentration (Pariona et al. Citation2017). For example, magnetite NPs seed priming for cucumber and lettuce seeds in 116 mg L−1 decreased germination % (Barrena et al. Citation2009). Despite that, for maghemite (γ-Fe2O3) NPs seed priming for mung bean decreased germination in a low concentration of 10 mg L−1 (Ren et al. Citation2011). Despite this, no changes or undesirable effects were observed for concentrations or sources tested for corn seed priming in our study, regarding germination variables as showed in . The interaction mechanism among Fe NPs and seed changes during germination process has not been well enlightened (Pariona et al. Citation2017).
Our results also demonstrated some interesting biometric changes when seeds were primed in levels between 50 and 70 mg L−1 with Fe3O4 NP, resulted in a positive root and shoot elongation as well as in root and shoot dry biomass production. As shown in and , our data are in consistence with findings (Alidoust and Isoda Citation2014; Li et al. Citation2013; Pariona et al. Citation2017). These researchers found some beneficial results were shown in preceding researches that applied maghemite and magnetite NPs as seed priming for watermelon, rice and corn, respectively.
However, biological indicators of toxicity such as reduction of corn seedling growth were observed high concentrations of seed priming. This effect is more likely that a Fe concentration threshold is the problem (Jalali et al. Citation2017). Batty and Younger (Citation2003) studying the effects of Fe concentrations for Phragmites australis, seedling growth, realised a threshold of Fe 1 mg L−1 concentration above which the growth was significantly inhibited. The authors suggested the threshold might be lessened for new plants. It is likely that Fe NPs remains in the seeds restrained cellular division and embryonic axis development (Takahashi et al. Citation2011) at higher concentrations, these cited effects may be the reason by the decreasing of some variables here studied as shown in and (A,B).
Our results were quite intriguing, because it settles Fe NPs had the ability to improve development of corn seedlings, but their effects were concentration dependent. Therefore, should always be a right Fe NPs concentration, when plants will show the higher and best responses. Altogether, the results showed that Fe NPs easy way synthesis are latent fertiliser for seed priming. The undesired effects of Fe NPs were seen just in higher concentrations and were smaller than those shown by FeCl3 ((B)). Root dry biomass production was negatively concentration-dependent affected with increase in soluble concentration. The results clearly signalled that exposure of iron chloride was more toxic than other iron sources (both nano and bulk forms) corn root seedlings. Poor root seedling growth in seeds primed with FeCl3 indicates the possible toxic effects of Cl− on cellular respiration (Stankovi et al. Citation2011). Normally, soluble sources release the nutrient instantaneously to the plants at once, which can be a risk to the plants since higher availability of one element can promote toxicity or deficiency of other elements that compete for the same absorption binding site. On the other hand, NPs through mechanisms such as insoluble and smaller size allow nutrient slow or controlled delivery, in returning environmental and biological triggers more accurately.
Beside this, supplying Fe NPs seems to be more efficient to promote growth, when compared to Fe bulk ((A)). It was needed lower concentration of Fe NPs seed priming to obtain the maximum plant response.
Furthermore, the data showed here offer insight into the short-term impacts of Fe fertilisation how these effects might differ from their sources and concentrations when applied as seed priming for corn seed. These results prove the need to increase and improve studies nonfertiliser and of course Fe NPs, to obtain better understanding of the mechanism function.
Herein, it has been demonstrated that it is possible to synthesise Fe NPs in a simple and fast way, in addition to the fact that these particles are effectively used as bio functional sources of crystalline iron oxide. The seeds primed with Fe NP proved to be more effective compared with FeCl3 and Fe bulk counterparts and can be used sustainably to raise root and shoot growth potential. However, the observed concentration that promotes these beneficial effects on plant growth is between 50 and 70 mg L−1. Therefore, further studies are necessary on the durability of Fe NPs response at the later phase of corn plant growth and development.
Authors’ contributions
Conceptualisation: Esper Neto, M.; Jackson, K.A.; Britt, D.W; Batista, M. A.; Inoue, T.T. Data acquisition: Esper Neto, M.; Jackson, K.A.; Britt, D.W. Design of methodology: Esper Neto, M.; Batista, M. A.; Inoue, T.T. Coneglian, C.F. Software development: Esper Neto, M.; Jackson, K.A.; Britt, D.W; Batista, M. A. Coneglian, C.F. Writing and editing: Esper Neto, M.; Jackson, K.A.; Britt, D.W; Batista, M. A.; Inoue, T.T. Coneglian, C.F.
Acknowledgements
The authors thank CAPES and CNPq PROC 141140/2017. Grupo de Estudos em Solos e Plantas-UEM and Utah State University.
Disclosure statement
No potential conflict of interest was reported by the author(s).
Additional information
Notes on contributors
Michel Esper Neto
Michel Esper Neto, PhD, Agronomy Department (UEM). Soil Science and Plant Nutrition. Research interests: Nanofertilizer and Soil Fertility. E-mail: [email protected]
David W. Britt
David W. Britt, PhD, Bioengineering, Biological Engineering Department Professor (USU), Research interests: Biomaterials, nanoparticles, Nanoparticle interactions. E-mail: [email protected]
Kyle Alan Jackson
Kyle Alan Jackson, graduate in Biological Engineering. Research interests: nanoparticle, bioactivity in plant, nanoparticle synthesis and application. Email: [email protected]
Carolina Fedrigo Coneglian
Carolina Fedrigo Coneglian, PhD candidate, Agronomy Department. Soil Science and Plant Nutrition. Research interests: Soil Fertility. E-mail: [email protected]
Tadeu Takeyoshi Inoue
Tadeu Takeyoshi Inoue, PhD, Agronomy Department Professor (UEM). Soil Science and Plant Nutrition. Research interests: Plant Nutrition. E-mail: [email protected]
Marcelo Augusto Batista
Marcelo Augusto Batista, PhD, Agronomy Department Professor (UEM). Soil Science and Plant Nutrition. Research interests: Soil Chemistry and Fertility. E-mail: [email protected]
References
- Alidoust D, Isoda A. 2014. Phytotoxicity assessment of γ-Fe2O3 nanoparticles on root elongation and growth of rice plant. Environ. Earth Sci. 71:5173–5182.
- Barrena R, Casals E, Colón J, Font X, Sánchez A, Puntes V. 2009. Evaluation of the ecotoxicity of model nanoparticles. Chemosphere. 75:850–857.
- Batista MA, Inoue TT, Esper Neto M, Muniz AS. 2018. Princípios de fertilidade do solo, adubação e nutrição mineral. p. 113–161. In: Brandão Filho JUT, Freitas PSL, Berian LOS, Goto R. Hortaliças-fruto. 1oed.
- Batty LC, Younger PL. 2003. Effects of External iron Concentration upon Seedling Growth and Uptake of Fe and Phosphate by the Common Reed, Phragmites australis (Cav.) Trin ex. Steudel. Ann. Bot. 92:801–806.
- Brasil, Ministério da Agricultura, Pecuária e Abastecimento. 2009. Regras para análise de sementes. Brasília, DF: MAPA/ACS.
- Claessens M, Contor L, Dhonukshe-Rutten R, De Groot LCpgm, Fairweather-tait SJ, Gurinovic M, Koletzko B, Van Ommen B, Raats MM, Van’t Veer P. 2013. Principles and future for deriving micronutrient recommendations. Crit. Rev. Food Sci. 53:1135–1146.
- Duran M, Medina-llamas M, Cassanji GB, Lima R, Almeida E, Macedo WR, Mattia D, Carvalho HWP. 2018. Bean seedling growth enhancement using magnetite nanoparticles. J. Agric. Food Chem. 66:5746–5755.
- Harris D, Rashid A, Miraj G, Arif M, Shah H. 2007. ‘On-farm’ seed priming with zinc sulphate solution—a cost-effective way to increase the maize yields of resource-poor farmers. Field Crop Res. 102:119–127.
- Hell R, Sthephan UW. 2003. Iron uptake, trafficking and homeostasis in plants. Planta. 216:541–551.
- Jalali M, Ghanati F, Modarres-Sanavi AM, Khoshgoftarmanesh AH. 2017. Physiological effects of repeated foliar application of magnetite nanoparticles on maize plants. J. Agron. Crop Sci. 203:593–602.
- Lal R. 2008. Promise and limitations of soils to minimize climate change. J. Soil Water Conserv. 63:113A–118A.
- Li J, Chang PR, Huang J, Wang Y, Yuan H, Ren H. 2013. Physiological effects of magnetic iron oxide nanoparticles towards watermelon. J. Nanosci. Nanotechnol.13:5561–5567.
- Liu R, Lal R. 2015. Potentials of engineered nanoparticles as fertilizers for increasing agronomic productions. Sci. Total Environ. 514:131–139.
- Marcar NE, Graham RD. 1986. Effect of seed manganese content on the growth of wheat (triticum aestivum) under manganese deficiency. Plant Soil. 96:165–173.
- Martin JH, Coale KH, Johnson KS, Fitzwater SE, Gordon RM, Tanner SJ, Hunter CN, Elrod VA, Nowicki JL, Coley TL, et al. 1994. Testing the iron hypothesis in ecosystems of the equatorial Pacific Ocean. Nature. 371:123–129.
- Olivares M, Walter T, Hertrampf E, Pizarro F. 1999. Anaemia and iron deficiency disease in children. Br. Med. Bull. 55:534–543.
- Pariona N, Martinez A, Hdz-garcıa HM, Cruz LA. 2017. Effects of hematite and ferrihydrite nanoparticles on germination and growth of maize seedlings. Saudi J. Biol. Sci. 24:1547–1554.
- Ren HX, Liu L, Liu C, He SY, Huang J, Li JL, Zhang Y, Huang XJ, Gu N. 2011. Physiological investigation of magnetic iron oxide nanoparticles towards Chinese Mung Bean. J. Biomed. Nanotechnol. 7:677–684.
- Saric T, Saciragic B. 1969. Effect of oat seed treatment with microelements. Plant Soil. 31:185–187.
- Stanković A, Veselinović LJ, Škapin, SD, Marković S, Uskoković D. 2011. Controlled mechanochemically assisted synthesis of ZnO nanopowders in the presence of oxalic acid. J. Mater. Sci. 46:3716–3724.
- Takahashi F, Mizoguchi T, Yoshida R, Ichimura K, Shinozaki K. 2011. Calmodulin-dependent activation of MAP kinase for ROS homeostasis in Arabidopsis. Mol. Cell 41:649–660.
- Vose PB. 1982. Iron nutrition in plants: a world overview. J. Plan. Nutr. 5:233–249.
- Zimmermann FJP. 2014. Estatística aplicada à pesquisa agrícola. Brasília, DF, Brazil: Ed. Embrapa.