ABSTRACT
Our study aimed to determine the effect on barley (Hordeum vulgare L.) of adding potassium polyacrylate to a sandy soil under normal watering condition and drought. We focused on morphological and physiological characteristics of barley and its rhizosphere bacterial community structure using Illumina Miseq sequencing. It was found that under normal watering condition (70% of soil water holding capacity (WHC)), the soil water retention increased with the SAP addition to the soil. Barley plant height and weight, leaf relative water content and protein content decreased under drought (35% of soil WHC), the addition of SAP eliminated the negative effect of drought on plants, the proline content was reduced from 2.71 μg g−1 leave dry weight with stressed plants to 0.99 μg g−1 with only 0.2% of SAP. The barley rhizophere bacterial community was affected by drought. Changes in relative abundance were noticed at both phyla and OTU level. The SAP addition (0.8%) shifted the barley rhizophere bacterial community to become in part more similar to that of the normal watering condition. Also, we found that the SAP addition had a side effect itself on the barley rhizosphere bacterial community other than its capacity of water holding.
Introduction
Drought is one of the main threats to agriculture in arid regions. Soil water depletion inhibits numerous important processes, thus affect the plant’s growth and physiology by disrupting its water status as well as the composition of plant-associated microbiomes (Naylor et al. Citation2017; Santos-Medellín et al. Citation2017). Recently, it has been shown that super absorbent polymers (SAPs) have a great potential to relieve drought stress.
SAPs are three dimensional, cross-linked polymers with a high capacity of water absorption and retention (Sojka and Entry Citation2000). The rapid absorption of water by the SAPs qualifies them to retain efficiently water that is provided either by rain or irrigation (Abedi-Koupai et al. Citation2008; Sojka and Entry Citation2000). This can enhance soil water holding capacity (WHC), increase water use efficiency and in this way stimulate plant growth (Hutterman et al. 1999; Abedi-Koupai et al., Citation2008). However, the impact of SAPs on soil microbial communities remains controversial and it has not been studied whether it affects the composition of the microbiome of the rhizosphere.
We hypothesise that the addition of SAP under drought conditions will improve the soil WHC and therefore, the amount of available water for plants by which the composition of rhizosphere bacterial community could shift to that of non-drought stress conditions. To test this hypothesis, we developed a drought assay for barley, exploring the effect of drought stress on the composition of the rhizosphere microbiome and how such change is affected by SAP.
The SAP impact on the microbial community had been shown using classical approaches to study the microorganism diversity and abundance (El-Hady and Abo-Sedera Citation2006; Mikiciuk et al. Citation2015), the soil enzymatic activity (Sarapatka et al. Citation2004; DeVerennes and Queda Citation2005) and the evaluation of the soil microbial biomass carbon and soil microbial respiration (Xi et al. Citation2013; Khodadadi Citation2017). However, in none of these studies, the effect of SAP on microbiome composition was studied using high-throughput sequencing technology (Caoa et al. Citation2017; Wang et al. Citation2019).
In this paper, in the first part, we aimed to determine the effects of using different amounts of a superabsorbent polymer on both soil WHC and barley plant growth cultivated on sandy soil and under drought conditions (35% relative soil moisture). In the second part, we examined the impact of using 0.8% of the superabsorbent polymer on the composition of the bacterial community in barley rhizosphere under drought conditions.
Material and methods
Super absorbent polymer
The SAP used in this study is a cross-linked copolymer of acrylamide and potassium acrylate (Evonic industry-Allemagne) that was available in the Algerian market. It is commonly used for agricultural purposes. The presented SAP had a WHC of 299.86 g g−1.
Soil
The soil samples were collected from the experimental research station of the University of Science and Technology Houari Boumediene (USTHB) located in El-Menia region, Algeria (30°37′00.8″N, 2°52′47.0″E). The area has an arid climate with a mean annual rainfall of 34 mm. The soil selected for the experiments is classified as sandy loam soil with basic pH (8.34). Soil organic matter content was also low, around 0.5%. The soil used in the experiments was a mixture of two topsoil (0–20 Cm), the first one was collected from a barren field and the second one was collected from a 3-month-old winter barley (Hordeumvulgare L.) cultivated field. The physical and biochemical properties of soils were determined using standard methods (Berthrong et al. Citation2013) and summarised in .
Table 1. The main physical and chemical properties of the two type of soil used in the experiment.
To test the effect of SAP on soil WHC, different concentrations of SAP (0, 0.2, 0.4, 0.6, 0.8 and 1%) were added to dry soil. The pots were saturated with distilled water and the surface of the pots was covered to prevent evaporation, then left for 24 h to drain. After that, the pots were weighted in order to determine the WHC (Zhao et al. Citation2014).
Experimental design
To determine the effect of different amounts of SAP (0, 0.2, 0.4, 0.6, 0.8 and 1 g per 100 g of dry soil) on barley plant growth under drought conditions, we conducted a pot experiment under lab conditions. The soil samples, barley cultivated soil and sterilised bulk soil in a ratio of 1:9 were put into 60 pots (500 ml plastic pots) then the SAP was mixed with soil. Before sowing seeds were surface sterilised with 4% bleach for 5 min, then washed six times thoroughly with sterilised distilled water, after that incubated at 25 °C for 3–4 days. In each pot, we planted 10 healthy barley cultivars with an identical root length.
Pots with seedlings were maintained at 30 °C with low humidity and photoperiod lighting of 16 h of light and 8 h of dark. These conditions are similar to those in El-Menia during the barley growing season.
The pots were initially irrigated with Fareus solution (0.12 g MgSO4·7H2O, 0.1 g KH2PO4, 0.358 g Na2HPO4·12H2O, 1 ml 15 mM Fe-Citrate, 2.5 ml Sporen-elements β) until the soil reached its maximum water holding capacity, which is 96 ml. Then, all pots were watered only until the soil reached 70% of soil WHC.
After 7 days of growth, five pots from all experimental groups were subjected to drought which was created by a 50% of reduction of the soil moisture (35% of soil WHC). Meanwhile, the other control group was maintained at 70% of WHC.
After 28 days of growth, plants were harvested for the morphological and biophysical and analysis and the rhizospheric soil to determine the bacterial community.
Plant analysis
After the harvesting, growth parameters were measured for each plant. The height of the shoot, the length of the root, the fresh weight (FW) and the dry weight (DW) was measured.
The relative water content (RWC) of barley plants was calculated to determine the effect of SAP on plant physiology. Fresh leaves were weighed (FW) then each leave was submerged in distilled water for 24 h in dark and weighed again to determine the (SW), then the leaves were weighed for the third time after drying at 70 °C for 48 h in the oven (DW) (Wang et al. Citation2016).
The determination of total protein was performed according to the method of Bradford (Citation1976). Fifty milligrams of fresh leaves were mixed with 2 ml of Sodium Borate (0.1 N; pH8.8) and centrifuged for 15 min at 12,000 rpm and 10 °C. After that 100 µl of the supernatant is added to 3 ml of Biorad reactive. Then the absorbance is measured at 595 nm spectrophotometrically.
Proline content was performed according to Bruand et al. (Citation2003). Twenty milligrams of fresh leaves were mixed with 1 ml of distilled water and incubated for 1 h at 90 °C. After centrifugation at 13,000 rpm, 1 ml of the supernatant was homogenised with 2 ml of Ninhydrine and incubated for 20 min at 95°C, then 3 ml of toluene was added to the homogenate, then the absorbance is measured at 520 nm spectrophotometrically.
Rhizosphere bacterial community
The rhizosphere soil was collected from barley plants cultivated under normal watering irrigation (70% of soil WHC) and water deficit (35% of WHC) and plants that were cultivated under drought conditions (35% of WHC) with 0.8% of SAP.
DNA was extracted from 0.25 g of each rhizosphere soil samples using the Power Soil ADN kit (Mobio, Carlsbad, CA, USA). The concentration and quality of the DNA were determined using a spectrophotometer (NanoDrop 2000, USA) and electrophoresis Agarose gel 1%.
The DNA extracted from each soil sample served as a template for bacterial 16S rRNA. The 515F (forward primer) and 907R (reverse primer) primer sets were used for bacterial 16S rRNA genes V4 region amplification. The pyrosequencing Illumina MiSeq 2.0 was performed according to Caporaso et al. (Citation2010) protocol and Illumina, San Diego, CA, USA.
The raw 16S rRNA genes sequence pyrosequencing data were processed using MOTHUR following the standard operating procedure with some modifications (Clarke and McCaig Citation1982). Sequences with more than one mismatch to a primer, any mismatch to a barcode, any ambiguous base call, homopolymers longer than eight bases or reads shorter than 250 bases were eliminated; the filtered sequences were trimmed and assigned to each soil sample based on unique barcodes. The unique sequences for bacterial 16S rRNA gene data were aligned against the Silva bacterial database in MOTHUR (Bates et al. Citation1973), after screening, filtering, pre-clustering and the removal of chimeras, the retained sequences were classified against the Silva database for bacteria (Schloss et al. Citation2009) then, they were used to build a distance matrix with a distance threshold of 0.2. Finally, using the average neighbour algorithm with a cut-off of 97% similarity, sequences were clustered into operational taxonomic units (OTUs).
All the above analysis results were reported as the mean and standard error of three replicates. Multivariate analysis of variance was applied to test the groups’ differences with a significance level of p < .05. To compare the microbiota among the different samples, we performed Principal Co-ordinates Analysis (PCoA) on the relative rhizosphere bacterial community.
Statistical analysis
The data for this study were subjected to analysis of variance one-way ANOVA and Fisher's least significant difference (LSD) to evaluate significant differences among all treatments at the 5% level using RStudio v. 2.13 (R Development Core Team, 2016). The standard error (SE) was calculated and shown in the figures. Figures were constructed with Microsoft Office EXCEL 2016.
Results
Soil water holding capacity
The WHC of our soil from the Sahara agricultural field is 24 ml per 100 g soil. This low WHC is due to the sandy nature of the soil. We tested whether we could improve the WHC by the addition of SAP.
Different amounts of SAP (0.2, 0.6, 0.8 and 1.0 g) were added to 100 g of dry soil. This was mixed well before water was added. This resulted in an increased soil WHC () to 36 ml per 100 g soil at 0.2% of SAP and up to 85 ml per 100 g soil when 1% of SAP was added, which means an increase of soil WHC of more than 3-fold. Next, we tested which amount of SAP can stimulate barley growth best.
Figure 1. Soil WHC with and without SAP. Values are given as the mean of five independent replicates, and bearing different letters (a,b,c,d,e,f) are significantly different from each other according to the least significant difference (LSD) test (p < .05). The bars represent the standard error. C, Control. 0%, 0.2%, 0.6%, 0.8% and 1% are the amounts of SAP added to dry soil.
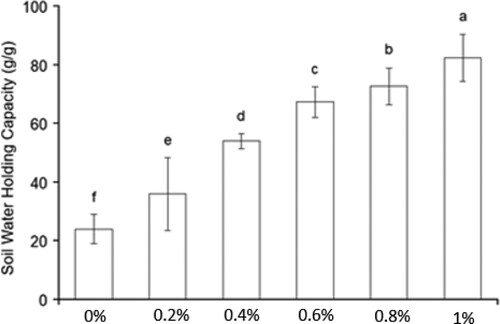
Plant growth
Morphology
To test which SAP level can stimulate the growth of barley best, we first developed a drought assay (see Material and methods). Barley plants were first grown for 7 days in Sahara soil at 70% of WHC (16.8 ml water for every 100 g soil) before the drought treatment was applied. Control plants were kept at 70% WHC, whereas the drought stress plants were not watered till they reached 35% WHC (8.4 ml water for every 100 g soil) after which it was kept at this level for 21 days.
Twenty-eight-day-old plants were harvested. Then shoot height, length of the root system, and fresh and dry weight of the plants were measured. These parameters provide a first insight in the effect of SAP on the growth and development of barley under drought condition.
The data are presented in . This showed that drought had severe effect on fresh and dry weight as it is reduced with about 25% and 36%, respectively. In contrast, the effect on the length of the roots and the height of the shoot is less strong. Barley plant subjected to the drought treatment have a significant shorter root system compared to control 9.57 ± 0.09 vs. 8.60 ± 1.08 Cm. The shoot height from control and drought stress plants was significantly different; 19.25 ± 0.07 and 18.60 ± 1.04 Cm, respectively.
Table 2. Effect of super absorbent polymer on plant growth parameters. Values are given as the mean of five independent replicates, and bearing different letters (a,b,c,d,e,f), they are significantly different from each other according to the least significant difference (LSD) test (p < .05). C is Control. 0%, 0.2%, 0.6%, 0.8% and 1% are the amounts of SAP added to dry soil.
When 0.2% of SAP is added, the root length is significantly longer (10.10 ± 0.07 Cm) than that of the drought stress (8.60 ± 1.08 Cm) and control plants (9.57 ± 0.09 Cm). At higher concentrations of SAP, the length of the root system gradually decreases. The effect on the shoot height is more or less the opposite. As they become slightly larger with increasing SAP levels. At 0.2%, the shoot height (19.33 ± 0.40 Cm) is similar to that of the control, whereas at the highest levels of SAP, it is about 20.6 ± 0.35 Cm.
The effect of SAP on fresh and dry weight () is very strong. At 0.2% SAP, the fresh and dry weight is already significantly higher than that of control plants. At the highest SAP levels, the fresh weight is more than 2-fold higher than that from drought stress plant and even 35% higher than that of control plants while the dry weight reaches its maximum at 0.6% of SAP then remained stable at higher concentrations . So although control plants obtain twice as much water as SAP-treated plants, at all concentrations tested, the biomass of SAP-treated plants is significantly higher than that of control plants.
So under drought conditions, SAP has a positive effect on biomass of barley, we next determined its effect on the physiology of the plants.
Physiology
Leaf RWC is an indicator used to show the stress intensity of plants. RWC is determined by measuring fresh weight (FW) and weight after soaking in water (Saturated weight, SW). After subtracting dry weight, the numbers reflect the amount of water that is and maximally can be present in plants. The ratio of the two values (multiplied by 100) is the RWC. At RWC = 100%, the plant is saturated with water. Healthy growing plants have RWC values of >80. Below this level, plants experience drought stress. The RWC values are presented in . It shows that the drought treatment caused a significant decrease of the RWC as control plants have an RWC level of almost 90%, whereas this of stressed plants is lower than 60%. SAP addition markedly increased the RWC. At 0.4% SAP, the RWC is similar to that of control plants and at 1.0%, it is even more than 98%.
SAP showed a very positive effect on plant physiology under drought conditions, which led us to determine its effect on protein and proline content.
In order to determine whether protein metabolism was affected by drought total leave protein content was measured. The data are shown in . Total leave protein content was about 50% reduced by the drought stress (3.12 vs 1.66 μg g−1 of leave dry weight).
The application of the SAP increased protein content; 0.2% SAP increased the protein content almost to the control level and at 0.6–1.0%, it was more than 25% higher than in control (3.92 vs 3.12 µg g−1 dry weight).
Under stress conditions, plants accumulate in general proline. Also the drought stress caused more than 2.5 times increase of the free proline content of the barley plant cultivated under deficit water conditions, it goes from 1.04 µg g−1with the control to 2.71 µg g−1 leave dry weight with stressed plants.
The application of 0.2% SAP reduced the accumulation of proline (0.99 µg g−1 leave dry weight). At higher levels of SAP, proline accumulation was even lower than under well-watered control conditions.
Composition of the barley rhizosphere bacterial community
The composition rhizosphere bacterial community was determined after the seedlings of barley were grown for 28 days under normal water condition. The bacterial community of rhizosphere was analysed by 16S rDNA amplicon sequencing of 3 replicates on Miseq2000 platform (Illumina). The raw sequence data were demultiplexed, trimmed and quality filtered, resulting in a reduction to 148,582 reads, they were clustered in OTUs based on 97% similarity. After removing low abundance OTUs and normalising to account for differences in read depth, the data set included 336 OTUs.
The taxonomic assignment of OTUs of the normal condition revealed13 phyla and 4 of these had a relative abundance (RA) higher than 1% (). The most abundant phylum was Proteobacteria (77.57%), followed by Bacteroidetes (11.62%), Firmicutes (6.51%) and Actinobacteria (2.25%). The Proteobacteria included three classes; Alpha, Gamma and Deltaproteobacteria. Gamma Proteobacteria was the most abundant class with an RA of 68.93%. In this class, Pseudomonadales were represented by 39.07% followed by Enterobacteriales with 17.01% of the bacterial community. In the Alpha Proteobacteria (8.61%), Rhizobiales formed the vast majority (6.11%).
Figure 2. Phyla distribution of the rhizosphere bacterial community under normal and drought conditions with and without SAP. Values are given as the mean of three independent replicates, and bearing different letters (a,b) are significantly different from each other according to the least significant difference (LSD) test (p < .05). Filled black bars, normal wattering conditions; open bars, drought condition; filled grey bars, drought condition with 0.8% of SAP.
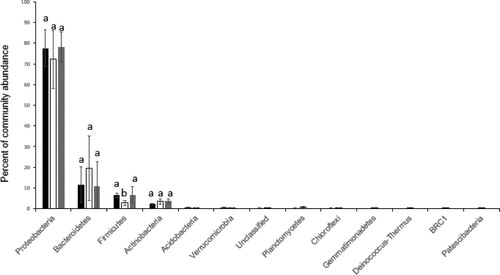
The 20 most abundant OTUs ((a)) of the rhizosphere bacterial community formed nearly 80% of the total community and they belonged to three phyla. Thirteen OTUs (66.55%) belonged to Proteobacteria, 4 OTUs (4.15%) to Firmicutes and 3 to Bacteroidetes (9.26%). Within the Proteobacteria, a huge diversity was present as the 13 OTUs belonged to 6 families. Five OTUs (39.58%) belonged to Pseudomondaceae, 3 OTUs (2.30%) to Rhizobiaceae and 2 OTUs (7.48%) to Burkholdraceae, the remaining 3 OTUs, belonged to Enterobacteriaceae (10.74%), Devisioaceae (0.72%) and Coulobacteriaceae (0.68%), respectively.
The effect of drought on the barley rhizosphere bacterial community
Drought stress was applied on barley plants after growing for 7 days under normal conditions (70% WHC), after which the water content was reduced to 35%WHC. The plants were grown for an additional 21 days at this drought stress condition.
The OTUs of the rhizosphere of the drought condition belonged to 13 phyla. The four most dominant phyla are the same as those of the control condition (). The RA of Bacteroides was markedly increased (4.5 times), whereas the RA of Firmicutes was significantly reduced (6.5 vs. 2.8%) at p < .05. Classes within Firmicutes showed two distinct responses. While Bacilli OTUs were overabundant under drought, clostridia OTUs were depleted significantly at p < .05.
To determine the effect of water deficit on microbiome composition unconstrained principal coordinate analyses (PCoA) of the Bray–Curtis distances were performed. These analyses demonstrated that the rhizosphere microbiome of normal and deficit conditions seemed to cluster in distinct groups (). However, a permutational multivariate analysis of variance (PERMANOVA) was performed using the Bray–Curtis distances for all control and deficit samples. This analysis indicated that at this global level, the microbiomes were not significantly influenced by the drought treatment. Therefore, we decided to determine whether individual OTUs are significantly affected.
Figure 3. The PcoA of the Bray–Curtis distances for control, drought and drought with SAP addition treatments. Values are given as the mean of three independent replicates. Filled triangle, normal wattering conditions; filled circle, drought condition; filled square, drought with 0.8% SAP.
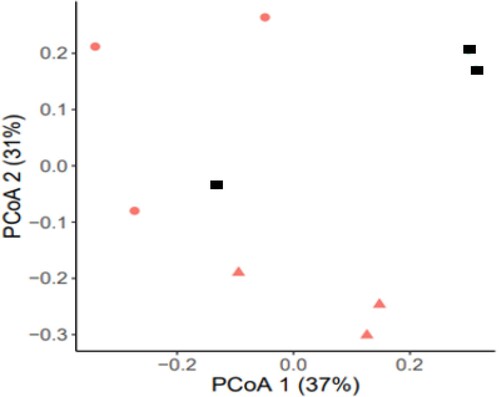
The most abundant OTUs from the drought conditions (Top 20 OTUs) represented about 80% of the bacterial community and they represented 62.21% of the bacterial community of the normal water condition. They showed more diversity compared to the top 20 OTUs from the normal watering conditions, they were affiliated to four phyla with a dominance of Proteobacetria (15 OTUs), Bacteroidetes and Actinobacteria were represented by two OTUs each with 17.62% and 1.18% of the total bacterial community, respectively, and one OTUs (1.42%) was affiliated to Firmicutes.
To further compare the differences in taxonomic composition and to assess whether some bacterial taxa were differentially abundant, we analysed whether the 20 most abundant OTUs of the normal and drought stress, respectively, were differentially expressed 15 OTUs from the 20 most abundant OTUs under normal conditions were depleted under drought condition ((a)), 7 OTUs of the 20 most abundant normal OTUs showed a decrease of more than 3 folds and in four cases, this difference was significant p < .05. For the 20 most abundant OTUs under drought conditions ((b)), six OTUs showed a decrease of more than 3-fold and only OTU_10 was significantly decreased at p < .05.
Figure 4. Most abundant bacteria community structure in barley rhizosphere at the OTU level under normal condition (a) and deficit condition (b). Values are given as the mean of three independent replicates, and bearing different letters (a,b) are significantly different from each other according to the least significant difference (LSD) test (p < .05). Filled black bars, normal wattering conditions; open bars, drought condition; open arrow, OTUs decreased more than 3 floods.
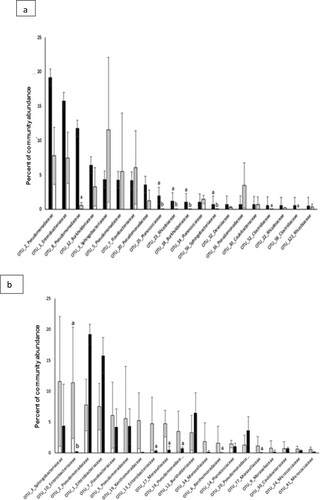
Effect of SAP on the rhizosphere microbiome
The barley plants cultivated under drought with SAP addition were healthy. Therefore, we hypothesised that the addition of SAP under drought conditions would shift the microbiome composition to that of plants grown under normal watering conditions. To test this hypothesis, we cultivated plants with 0.8% of SAP under drought conditions (35% of soil WHC).
Under drought condition, the RA of Proteobacteria and Firmicutes was decreased, while the RA of Bacteroidetes and Actinobacteria had enriched. SAP addition led to an increase of the relative abundance of Proteobacteria and Firmicutes and a decrease of the RA of Bacteroidetes. So, the RA of these three phyla shifts by the addition of SAP to that of normal watering conditions. For Actinobacteria, with SAP addition, the RA was higher than both control and drought conditions ().
To determine the effect of SAP addition on microbiome composition, a PCoA of the Bray–Curtis distances was performed. It is showed that the bacterial community of barley rhizosphere seemed to cluster in distinct groups depending on the applied treatment (). The bacterial community from the drought condition with SAP addition was situated in the middle, between the two other clusters. However, using the PERMANOVA, the SAP addition did not lead to a significant global difference, like was shown for normal and drought microbiomes. Further, we focused on the 13 individual OTUs that were at least 3-fold different between normal and drought, of which 5 were significantly affected. We tested whether SAP addition changed these OTUs towards the normal condition.
Drought increased the RA of six OTUs (), four of these OTUs obtained an RA similar to that of normal watering by the addition of SAP. These are OTU_16, OTU_17, OTU_77 and OTU_10. The relative abundance of OTU13 was slightly reduced by SAP addition, whereas that of OTU_6 was not affected.
Figure 5. Enriched and depleted OTUs of barley rhizosphere with SAP addition. Values are given as the mean of three independent replicates, and bearing different letters (a,b) are significantly different from each other according to the least significant difference (LSD) test (p < .05). Filled black bars, normal wattering conditions; open bars, drought condition; filled grey bars, deficit with SAP addition.
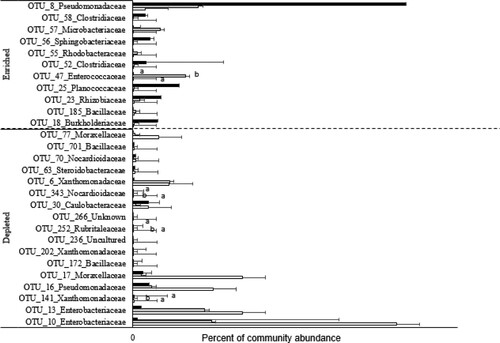
Of the seven OTUs of which the RA was markedly decreased by the drought only, the RA of OTU_8 showed a substantial increase by the addition of SAP. The RA of the others was hardly affected by SAP addition ().
Previously, it has been reported that SAP affects the physical properties of the soil and SAP might also be used as a carbon source by some microbes. To determine if the SAP addition could have another effect rather than the water supply we selected OTUs that had not different RA between control and drought but had reduced or increased 3-fold with the SAP addition.
Four OTUs had increased and 11 OTUs had decreased with the SAP addition. The OTU_47 and OTU_57 s had enriched to be in the top 20 most abundant OTUs under SAP treatment.
For the 11 OTUs that had a decreased RA with SAP addition, three of them had a significant decrease and only OUT_30 was on the 20 most abundant OTUs in normal and drought conditions.
Discussion
In the current study, we have shown that the use of SAP under drought has helped the barley plants to obtain similar physiological characteristics as the control plants, cultivated under normal watering condition. On the contrary, only a part of the rhizosphere microbiome becomes similar to that of the control.
The reduction in soil moisture led to drought stress, which caused both morphological and physiological changes in barley plants. Our results showed that plants biomass, leaf RWC, protein and proline content reduced significantly (). These are the major consequences of drought. The same results have also been found in several other species (Sajad et al. Citation2013; Saneoka et al. Citation2004).
Barley plants grown under drought conditions appeared to become healthy by the addition of SAP. Our results showed that biomass, RWC and protein content had significantly increased with the SAP addition (). SAPs are known for the huge amount of water that could absorb and retain, which increase the availability of water in the soil (Buchholz and Graham Citation1997; Hüttermann et al. Citation1999) and then create a better place for the plants establishment.
The SAP addition had a significant effect on the soil WHC (). The results we had are in consistence with findings by Hüttermann et al. (Citation1999) and Abedi-Koupai et al. (Citation2008), and explained the increase of the morphological and physiological traits of our barley plants ().
Previous studies have shown the positive effect of SAP application only through yield characteristics. In contrast, this study focused not only on morphological characteristics of plants, but also on several metabolic changes in response to drought. We showed that SAP contributes to the adaptation to this environmental constraint showed by the decreased proline levels (), as a main physiological parameter linked to water stress (Hong Bo et al. Citation2006; Khamssi et al. Citation2014). As plants became physiological very similar to plants growing under control conditions, we studied the effect of SAP on the rhizosphere microbiome.
The SAP effect on the rhizosphere microbiome has never been studied and only a few studies have been done on the effect of it on soil microbiome composition (Wang et al., Citation2019). To study this effect, we first had to determine the effect of drought on the rhizosphere microbiome.
The bacterial community of our barley rhizosphere was dominated by four phyla: Proteobacteria, Bacteroidetes, Firmicutes and Actinobacteria (). This is in line with previous studies (Bulgarelli et al. Citation2015; Qiao et al. Citation2017; Yin et al. Citation2017).
The rhizospheric bacterial community of the barley plants was dominated by 20 OTUs which represent almost 80% of the total density ((a)). These 20 OTUs were distributed over 3 phyla, with a dominance of the Proteobacteria with 13 OTUs. Proteobacteria contains bacteria with a great morphological, physiological and metabolic diversity which allows them to play a major role in the cycles of carbon, phosphorus and nitrogen (Delgado-Baquerizo et al. Citation2018).
In the current work, we analysed the effect of drought (35% of soil WHC) on the bacterial rhizosphere community of barley.
The PCoA analysis showed that the rhizosphere microbiome of barley plants under normal watering and drought conditions were cluster in different groups () and they were represented by the same phyla. However, the RA of the most abundant phyla were different (). The most significant change at the phyla level is the decrease of RA of Firmicutes. This change was not in line with previous studies which found that in moisture-limited soils, the RA of the Gram-positive phyla Firmicutes increased (Bouskill et al. Citation2013; Chodak et al. Citation2015; Hartmann et al. Citation2017; Dai et al. Citation2019). This phylum contains Clostridia and Bacilli. Clostridia have members with thick cell walls by which they are better resistant to water stress (Vollmer and Seligman Citation2010; Farrow et al. Citation1995; Wiegel et al. Citation2006), whereas Bacilli are less resistant (Weidenmaier and Peschel;Citation2008; Vollmer and Seligman Citation2010). In our study, Bacilli OTUs are far more abundant than Clostridia OTUs. This could explain why in our study the RA of the Firmicutes decreased under drought conditions.
The 20 most abundant OTUs under drought conditions were represented by four phyla. The three same phyla as normal condition (Proteobacteria, Bacteroidetes, Firmicutes) and Actinobacteria which was represented by two OTUs ((a)). It has been shown that during the dry season, root-associated communities show elevated abundance for Actinobacteria (Shakya et al., Citation2013)
With the SAP addition, the RA of Proteobacteria, Firmicutes and Bacteroidetes were more similar to these of the normal watering conditions (). This result could be explained by the ability of SAP to absorb a large amount of water and therefore increase the availability of water in the soil (Buchholz and Graham Citation1997; Hüttermann et al. Citation1999).
The comparison of the most abundant OTUs from the drought and control conditions revealed that some OTUs reduced more than 3-fold under drought conditions, while others had increased more than 3-fold ((a,b)). The microbiome under drought tends to involve changes in RA rather than the complete elimination of drought susceptible OTUs or new recruitment of tolerant ones (Naylor et al. Citation2017).
Our results showed that several of the affected OTUs by drought had shifted to be more similar to that of the control with the SAP addition. The majority of the OTUs that had an increased RA due to drought had a decreased RA in the presence of SAP. In contrast, only one of the OTUs with a decreased RA had a markedly increased RA by SAP addition. We cannot exclude that this difference in response to SAP is a coincidence as we studied a relatively small number of OTUs. However, the response of the OTUs with an increased RA under drought seems consistent with the cry-for-help hypothesis. This hypothesis proposes that plants under stress conditions have the ability to recruit beneficial microbes that can make them more tolerant to the stress. In line with this, the OTUs with an increased RA under drought might support plant growth under this abiotic stress condition. After the addition of SAP, the plant obtains an optimal physiological condition and no longer recruits these OTUs.
Our analyses on the barley rhizosphere bacterial community under SAP addition had shown that some OTUs were affected more related to the SAP-induced changes other than the watering condition (). Previous research (Bai et al. Citation2010; Caoa et al. Citation2017) suggested that adding SAP can influence the soil's physical and chemical properties like the soil pH, texture and water and/ or nutriments availability. It seems probable that such soil physicochemical properties have affected these SAP ‘specific’ OTUs (Zhalnina et al. Citation2015; Dong et al. Citation2017).
In summary, our study showed that the addition of SAP has a positive effect on the physiological properties of barley grown under drought conditions. We confirmed that drought-induced changes in the RA of certain bacteria that are part of the rhizosphere microbiome. The addition of SAP only in part restored these changes. SAP addition also induced changes unrelated to water availability. Under the growth conditions, we used this change in microbiome composition had no negative effect on the growth of barley. It remains to be studied whether such changes do affect the plants ability to cope with other stresses.
Disclosure statement
No potential conflict of interest was reported by the author(s).
Additional information
Notes on contributors
Bouherama Amina
Bouherama Amina is an Algerian biologist. She got her Magister degree in 2013 at Science and Technolgy University -Bab Ezzouar, Algeria for a work on the rhizobia associated to Acacia horrida of Algeria. She works on the structure and diversity of barley and alfalfa microbiome. She works as teacher at Yahia Fares University-Medea-Algeria.
Djouadi Samir
Djouadi Samir is an Algerian biologist. In 2018, he earned his PhD in eco-biology and plant promotion after a work on the rhizobia associated to lotus in Algeria. He is a teacher at Science and Technolgy University -Bab Ezzouar.
Schneijderberg Martinus
Schneijderberg Martinus is a Dutch molecular biologist and bioinformatics scientist. He is a researcher at Wageningen University. He works on the microbiome associated to plant.
Bisseling Ton
Bisseling Ton is a Dutch molecular biologist. He is a professor at Wageningen University and Research Centre. In 1975 Bisseling obtained a degree in biology from the Radboud University Nijmegen. Five years later he earned his PhD in molecular biology at Wageningen University.
Amrani Said
Amrani Said is an Algerian biologist. He is a professor at Science and Technolgy University -Bab Ezzouar. In 2010, he earned his PhD at Science and Technolgy University -Bab Ezzouar. He worked on legumineuse-rhizobia symbiosis.
References
- Abedi-Koupai J, Sohrab F, Swarbrick GW. 2008. Evaluation of hydrogel application on soil water retention characteristics. J Plant Nutr. 31(2):317–331. doi:10.1080/01904161003654030.
- Bai W, Zhang H, Liu B, Wu Y, Song J. 2010. Effects of super-absorbent polymers on the physical and chemical properties of soil following different wetting and drying cycles. Soil Use Manage. 26(2):253–260. doi:10.1111/j.1475-2743.2010.00271.x.
- Bates L, Waldren RP, Teare ID. 1973. Rapid determination of free proline for water-stress studies. Plant Soil. 39(1):205–207. doi:10.1007/BF00018060.
- Berthrong ST, Buckley DH, Drinkwater LE. 2013. Agricultural management and labile carbon additions affect soil microbial community structure and interact with carbon and nitrogen cycling. Microb Ecol. 66(1):158–170. doi:10.1007/s00248-013-0225-0.
- Bouskill NJ, Lim HC, Borglin S, Salve R, Wood TE, Silver WL, Brodie EL. 2013. Pre-exposure to short-term drought increases the resistance of subtropical forest soil bacterial communities to extended drought. ISME J. 7(2):384–394. doi:10.1038/ismej.2012.113.
- Bradford MM. 1976. A rapide and sensitive methods for quantification of microgram quantities of protein utilizing the principle of proteine dye binding. Anal Biochim. 72(1–2):248–254.
- Bruand A, Fernandez PN, Duval O. 2003. Use of class pedotransfer functions based on texture and bulk density of clods to generate water retention curves. Soil Use Manage. 19(3):232–242. doi:10.1111/j.1475-2743.2003.tb00309.x.
- Buchholz FL, Graham AT. 1997. Modern superabsorbent polymer technology. New York: John Wiley & Sons.
- Bulgarelli D, Garrido-Oter R, Münch P, Weiman A, Dröge J, Pan Y, McHardy A, Schulze-Lefert P. 2015. Structure and function of the bacterial root microbiota in wild and domesticated barley. Cell Host Microbe. 17(3):392–403. doi:10.1016/j.chom.2015.01.011.
- Caoa Y, Wanga B, Guoa H, Xiaoa H, Wei T. 2017. The effect of super absorbent polymers on soil and water conservation on the terraces of the loess plateau. Ecol Eng. 102:270–279. doi:10.1016/j.ecoleng.2017.02.043.
- Caporaso GJ, Lauber CL, Walters WA, Berg-Lyons D, Lozupone CA, Turnbaugh PJ, Fierer N, Knight R. 2010. Global patterns of 16S rRNA diversity at a depth of millions of sequences per sample. Proc Natl Acad Sci USA 108(Suppl 1):270–279. doi:10.1073/pnas.1000080107.
- Chodak M, Golębiewski M, Morawska-Ploskonka J, Kuduk K, Niklińska M. 2015. Soil chemical properties affect the reaction of forest. Soil bacteria to drought and rewetting stress. Ann Microbiol. 65(3):1627–1637. doi:10.1007/sl3213-014-1002-0.
- Clarke JM, McCaig TN. 1982. Excised-leaf water retention capability as an indicator of drought resistance of Triticum genotypes. Can J Plant Sci. 62(3):571–578. doi:10.4141/cjps82-086.
- Dai L, Zhang G, Yu Z, Ding H, Xu Y, Zhang Z. 2019. Effect of drought stress and developmental stages on microbial community structure and diversity in peanut rhizosphere soil. Int J Mol Sci 20(9):2265. doi:10.3390/ijms20092265.
- De Varennes A, Queda C. 2005. Application of an insoluble polyacrylate polymer to copper-contaminated soil enhances plant growth and soil quality. Soil Use Manage. 21(4):410–414. doi:10.1079/SUM2005354.
- Delgado-Baquerizo M, Oliverio AM, Brewer TE, Benavent-González A, Eldridge DJ, Bardgett RD, Maestre FT, Singh BK, Fierer N. 2018. A global atlas of the dominant bacteria found in soil. Science. 2:359–320.
- Dong W, Liu E, Yan C, Zhang H, Zhang Y. 2017. Changes in the composition and diversity of top soil bacterial, archaeal and fungal communities after 22 years conventional and no-tillage managements in northern China. Arch Agron Soil Sci. 63(10):1369–1381. doi:10.1080/03650340.2017.1281392.
- El-hady O, Abo-Sedera SA. 2006. Conditioning effect of compost and acrylamide hydrogels on a sandy calcareous soil. II-Physico-bio-chemical properties of the soil. Int J Agric Biol. 8:876–884.
- Farrow JAE, Lawson PA, Hippe H, Gauglitz U, Collins MD. 1995. Phylogenetic evidence that the gram-negative nonsporulating Bacterium tissierella (bacteroides) praeacuta is a member of the Clostridium subphylum of the gram-positive bacteria and description of Tissierella creatinini sp. nov. Int J Syst Bacteriol. 45(3):436–440. doi:10.1099/00207713-45-3-436.
- Hartmann M, Brunner I, Hagedorn F, Bardgett RD, Stierli B, Herzog C, Chen X, Zingg A. 2017. A decade of irrigation transforms the soil microbiome of a semi-arid pine forest. Mol Ecol. 26(4):1190–1206. doi:10.1111/mec.13995.
- Hong-Bo S, Xiao-Yan C, Li-Ye C, Xi-Ning Z, Gangh W, Yong-Bing Y, Zhao Chang-Xing Z, Zan-Min H. 2006. Investigation on the relationship of proline with wheat anti-drought under soil water deficits. Colloids Surf B. 53(1):113–119. doi:10.1016/j.colsurfb.2006.08.008.
- Hüttermann A, Zommorodi M, Reise K. 1999. Addition of hydrogels to soil for prolonging the survival of Pinushalepensis seedlings subjected to drought. Soil Til Res. 50(3–4):295–304. doi:10.1016/S0167-1987(99)00023-9.
- Khamssi NN, Golezani KG, Zehtab S, Najaphy A. 2014. Canopy growth, irrigation water use efficiency and relative water content of chickpea under accumulation to gradual water deficit conditions. IJPAES. 4:324–331.
- Khodadadi D. 2017. Effect of superabsorbent polymer on salt and drought resistance of Eucalyptus globulus. Appl Ecol Environ Res. 15(4):1791–1802. doi:10.15666/aeer/1504_17911802.
- Mikiciuk G, Mikiciuk M, Hawrot-Paw M. 2015. Influence of superabsorbent polymers on the chemical composition of strawberry (Fragariaananassa Duch.) and biological activity in the soil. Folia Hort. 27(1):63–69. doi:10.1515/fhort-2015-0015.
- Naylor D, DeGraaf S, Purdom E, Coleman-Derr D. 2017. Drought and host selection influence bacterial community dynamics in the grass root microbiome. ISME J. 11(12):2691–2704. doi:10.1038/ismej.2017.118.
- Qiao Q, Wang F, Zhang J, Chen Y, Zhang C, Liu G, Zhang H, Ma C, Zhang J. 2017. The variation in the rhizosphere microbiome of cotton with soil type, genotype and developmental stage. Sci Rep. 7(1):3940. doi:10.1038/s41598-017-04213-7.
- Sajad K, Mehdi S, Fardin G. 2013. Induction of drought tolerance in sweet basil (ocimumbasilicum L.) by salicylic acid. Int J Agr Food Res. 2:18–26. doi:10.24102/ijafr.v2i2.149.
- Saneoka H, Moghaieb REA, Premachandra GS, Fujita K. 2004. Nitrogen nutrition and water stress effects on cell membrane stability and leaf water relations in Agrostispalustris huds. Environ Exp Bot. 52(2):131–138. doi:10.1016/j.envexpbot.2004.01.011.
- Santos-Medellín C, Edwards J, Liechty Z, Nguyen B, Sundaresan V. 2017. Drought stress results in a compartment-specific restructuring of the rice root-associated microbiomes. mBio. 8(4):e00764–17. doi:10.1128/mBio.00764-17.
- Sarapatka B, Dudova L, Kroskova M. 2004. Effect of pH and phosphate supply on acid phosphatase activity in cereal roots. Biologia Bratislova. 59:127–131.
- Schloss PD, Westcott SL, Ryabin T, Hall JR, Hartmann M, Hollister EB, Lesniewski RJ, Oakley BB, Parks DH, Robinson CJ, et al. 2009. Introducing mothur: open-source, platform independent, community supported software for describing and comparing microbial communities. Appl Environ Microbiol. 75(23):7537–7541. doi:10.1128/AEM.
- Sojka RE, Entry JA. 2000. Influence of polyacrylamide application to soil on movement of microorganisms in runoff water. Environ Pollut. 108(3):405–412. doi:10.1016/S0269-7491(99)00194-3.
- Shakya M, Gottel N, Castro H, Yang ZK, Gunter L, Labbé J, Wellington M, Bonitos G, Vilgalys R, Tuskan G, et al. 2013. A multifactor analysis of fungal and bacterial community structure in the root microbiome of mature populus deltoides trees. PLoS ONE. 10:1371. doi:10.1016/j.tim.2009.12.004.
- Vollmer W, Seligman SJ. 2010. Architecture of peptidoglycan: more data and more models. Trends Microbiol. 18(2):59–66. doi:10.1016/j.tim.2009.12.004.
- Wang H, Bai W, Peng X, Han W, He J, Song J, Lv G. 2019. Effect of super absorbent polymer sodium polyacrylate on the bacterial community and associated chemistry of loessial soil. Arch Agro Soil Sci. 1:70–82. doi:10.1080/03650340.2019.1597268.
- Wang Z, Liu L, Chen Q, Wen X, Liao Y. 2016. Conservation tillage increases oil bacterial diversity in the dryland of northern China. Agron Sustain Dev. 36(2):28–36. doi:10.1007/s13593-016-0366-x.
- Weidenmaier C, Peschel A. 2008. Teichoic acids and related cell wall glycopolymers in gram-positive physiology and host interactions. Nat Rev Microbiol. 6(4):276–287. doi:10.1038/nrmicro1861. Epub 2008 Mar 10.
- Wiegel J, Tanner R, Rainey FA. 2006. An introduction to the family Clostridiaceae. Prokaryotes. 4:654–678. doi:10.1007/0-387-30744-3_20.
- Xi L, Ji-Zheng H, Yu-Rong L, Yuan-Ming Z. 2013. Effects of super absorbent polymers on soil microbial properties and Chinese cabbage (Brassicachinensis) growth. J Soils Sediments. 13(4):711–719. doi:10.1007/s11368-013-0657-7.
- Yin C, Mueth N, Hulbert S, Schlatter D, Paulitz TC, Schroeder K, Prescott A, Dhingra A. 2017. Bacterial communities on wheat grown under long-term conventional tillage and no-till in the pacific northwest of the United States. Phytobiomes. 1(2):83–90. doi:10.1094/PBIOMES-09-16-0008-R.
- Zhalnina K, Dias R, de Quadros PD, Davis-Richardson A, Camargo FAO, Clark IM, McGrath SP, Hirsch PR, Triplett EW. 2015. Soil pH determines microbial diversity and composition in the park grass experiment. Microb Ecol. 69(1):395–406.
- Zhao J, Zhang R, Xue C, Xue W, Sun L, Xu Y, Shen Q. 2014. Pyrosequencing reveals contrasting soil bacterial diversity and community structure of two main winter wheat cropping systems in China. Microb Ecol. 67(2):443–453. doi:10.1007/s00248-013-0322-0.