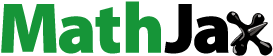
ABSTRACT
The long-term application of chemical fertilisers in calcareous soil causes the stable P in soil to accumulate and not be effectively utilised. In this study, whether acidic compost tea (CT) could increase the available P in calcareous soil was evaluated through a pot experiment. The P forms of the samples were analysed using Hedley sequential P fractionation. CT had significant effects on different soil P fractions and P uptake (p < 0.05). Compared with organic fertiliser (OF), acidic CT increased the availability of H2O-P and NaHCO3-P by 15.9% and 12.6%, respectively, it mainly comes from the dissolution of HCl-P and residue-P. CT also promoted the growth and yield of cotton and significantly increased P use efficiency by 13.8% and 31.6% (p < 0.05), respectively, compared with OF, because the H+ in CT reduced the pH of the calcareous soil, promoted the dissolution of stable P in soil, and reduced the fixation of P from the fertiliser. However, the organic matter in CT did not contribute much to the desorption of P. These findings provide a new idea for the activation and utilisation of P in calcareous soil.
Introduction
Water-soluble fertilisers are common fertilisers used to solve P deficiency in soil and improve crop yield through the drip irrigation system in Xinjiang. With the application of a large amount of phosphate fertiliser and almost no organic fertiliser (OF), a substantial portion of P fertiliser is usually ‘fixed’ into insoluble forms by a series of soil-dependent reactions in calcareous soils. The recovery of the applied fertiliser rarely exceeds 20%, mostly due to the conversion of soluble P into plant-unavailable forms (Ikram et al. Citation2019). Therefore, P deficiency has been reported in many calcareous soils.
P has the lowest mobility and availability among other nutrients in soil, especially in calcareous soil (Song et al. Citation2017). The P uptake of crops depends on the desorption characteristics of P. The P forms in soil are closely related to soil properties, such as pH, organic carbon (OC), calcium carbonate, and iron aluminum oxide (Giles et al. Citation2015; Yan et al. Citation2018). Soil pH changes the solubility of secondary Fe, Al, and Ca-P minerals in soil; changes the adsorption capacity of soil; affects the mineralisation of organic P (Po); and plays a critical role in maintaining P in soil (Sato et al. Citation2005). Besides, organic amendments are the source of water-soluble C, which could directly affect the solubility of P via four mechanisms. Organic molecules may (1) specifically sorb soil minerals and compete with P for sorption sites; (2) combined with surface-bound aluminum or iron, soluble organometallic compounds are formed to replace and release previously adsorbed P; (3) the adsorption on soil particles at non-specific adsorption sites increases the negative charge on the surface of particles, resulting in decreased electrostatic attraction of P on the soil surface; and (4) the formation of cation bridge leads to increased P adsorption sites (Ohno et al. Citation2007; Debicka et al. Citation2016). Thus, for calcareous soils with higher pH, reducing pH and the increasing organic matter (OM) content could improve soil P availability and the efficiency of phosphate fertiliser use (Braschi et al. Citation2003).
The high cost and inconvenience of fertilisation caused Xinjiang farmers to rarely use OFs. Thus, an acid liquid OF, namely, acidic compost tea (CT), suitable for drip irrigation systems was proposed in the present study. An ordinary CT is a liquid extract of a compost (Gross et al. Citation2008). It contains all the soluble nutrients, and a large percentage of organisms, including bacteria, fungi, protozoa, and nematodes, are extracted into the tea. However, previous studies on CT only focused on fertiliser efficiency and the prevention of pests and diseases (Kim et al. Citation2015; Samet et al. Citation2019; Corato, Citation2020; Li et al. Citation2020). Few studies have combined CT as a fertiliser and a nutrient activator. Different from traditional CT, acidic CT was created using phosphoric acid (pH = 1) as the extractant to extract chicken manure. The OM and acid in acidic CT may have a positive effect on the available P content in soil. Significant changes in labile inorganic P (Pi) and Po associated with the long-term use of organic and mineral fertilisers in calcareous soils have been reported (Pizzeghello et al. Citation2011). Yang et al. (Citation2019) found that adding OM could efficiently enhance P availability by reducing the strength of P adsorption and the maximum phosphate buffering capacity and increasing the desorption of P to some extent. Besides, reducing soil pH could increase P availability by reducing the formation of less soluble Ca phosphate in calcareous sandy soils (Eissa Citation2019). Several studies on the effect of organic acids on P availability have also been reported (Mihoub et al. Citation2017). Organic acid increased the P availability in soils by solubilising Pi fractions and releasing the P fractions bound to the Fe, Al, and fulvic acids of mineral surfaces (Miller and Fox Citation2011; Oburger et al. Citation2011; Oral and Uygur Citation2018). Thus, acidic CT contains OM, soluble OM, and the essential nutrients for crops. H+ could also alleviate the fixation of P in calcareous soils and increase the uptake of P by plants.
Many studies have reported P sorption inhibition that resulted from site competition and metal complexation by organic acids, animal and green manures, and biosolids (Jordán et al. Citation2020; Kim et al. Citation2015; Brucker et al. Citation2020). However, little is known about the effect of acidic CT on soil P lability, transformation rate, and forms. Thus, the present research aimed to investigate the effects of acidic CT on (1) different soil P fractions, (2) the uptake and utilisation efficiency by cotton, (3) the transformation of P forms in soil and fertiliser, (4) and the characteristics of CT that caused the P forms change.
Materials and methods
Acidic CT preparation and experimental design
OF and phosphoric acid (pH = 1) were prepared in this study. The OF was an organic chicken manure produced by ZeShang Biotechnology Co., Ltd (Shihezi City, Xinjiang), and it contained the following: OM content, 471.3 g/kg; total nitrogen, 302 g/kg; total P (TP), 201 g/kg; and total potassium, 3 g/kg.
The extract-to-OF mass ratio of CT was 5:1 (w/w). The OF was extracted using phosphoric acid (pH = 1) for 48 h, stirred every 8 h, and then filtered with a nylon mesh (400 mesh). The temperature was maintained at 25 °C throughout the preparation period. The properties of CT are shown in .
Table 1. Physical and chemical properties of acid compost tea.
In the pot experiments, the inner diameter and height of the pot were 30 and 30 cm, respectively, with a total of four repetitions. Each pot contained 10 kg of soil. Three cottons were planted in each pot. One unfertilised control treatment and three fertilisation treatments were described as follows: (1) OF, organic fertiliser; (2) CT, acidic CT; (3) HP, phosphoric acid (pH = 1); and (4) CK, unfertilised control. Fertilisation was conducted in accordance with the total fertilisation amounts of N, P2O5, and K2O. These amounts were all 2.4 g per pot in the fertilisation treatments (urea, N ≥ 46%; monoammonium phosphate, P2O5 ≥ 61%; and potassium sulfate, K2O ≥ 51%). The OM contents in the OF and CT treatments were the same, whereas the HP treatment had no OM. Chemical fertilisers were added to ensure that the total amount of nutrients in all fertilisation treatments was the same. The fertiliser was divided into 10 parts, and the fertilisation cycle was 10 days. The OF was manured once in the OF treatment at the beginning of the experiment. The CT, chemical fertilisers, and phosphoric acid were manured using a medical drip bag (0.5 L) at the root of cotton every 10 days.
Soil physical and chemical characteristics
A total of 10 fertilizations were carried out every 10 days from August 16, 2020, to November 16, 2020. Soil samples were collected at the bubbling period, and the sampling time was the third day after the final fertilisation.
The pot experiment was carried out at the Shihezi University Experimental Station (44°23′ N, 85°41′ E). The soil was a cultivated grey desert soil (calcaric fluvisol). Some of the soil properties at the beginning of the pot experiment were as follows: soil pH, 8.2; OM, 11.2 g/kg; total nitrogen, 1.0 g/kg; TP, 1.0 g/kg; available P, 10.9 mg/kg; and available potassium, 173.9 mg/kg.
Sequential P fractionation and chemical analysis
Sequential P fractionation was performed in accordance with Hedley et al. (Citation1982), as modified by Sharpley and Moyer (Citation2000). Dried soil samples (0.5000 and 0.149 mm) were extracted in 50 mL deionised H2O (H2O-P) and then in 30 mL 0.5 M NaHCO3 (NaHCO3-P), 30 mL 0.1 M NaOH (NaOH-P), and 30 mL 1 M HCl (HCl-P), each for 16 h. Each mixture was shaken on an end-to-end shaker at 100 times per min for 16 h and then centrifuged at 10,000 rpm at 4 °C for 10 min. The supernatant was filtered through a 0.45 μm acetate cellulose filter, and P was analysed in all extracts as described above. The residual P in soil after sequential extraction was determined by digestion with concentrated H2SO4 and HClO4, and it was termed residual P (residue-P). For the NaHCO3 and NaOH extracts, the TP concentration was determined after digestion with 0.5 g ammonium persulfate and 10 mL 0.9 M H2SO4. The Po in these two extracts was calculated as the difference between TP and Pi. The Pi content in the extracts was determined by colorimetry using ascorbic acid.
The P form in soil was divided into three categories: P that could be absorbed and used by crops, (1) labile-P: H2O-P and NaHCO3-P; P that is difficult to be used by crops, (2) moderately labile-P: NaOH-P and HCl-P; and (3) stable-P: residue-P.
Soil pH was measured at a soil-to-distilled water ratio of 1:5. Soil OM was determined using potassium dichromate oxidation method (Walkley and Black Citation1934). TP was digested with HClO4 and H2SO4 and determined via colorimetric method using ascorbic acid. The alkaline phosphatase activity was determined via diphenyl sodium phosphate method (Bao Citation2010), and total nitrogen was determined using Kjeldahl method (Jackson Citation1973). The Olsen-P in soil was determined using 0.5 m NaHCO3 (soil-to-liquid ratio of 1:20) and ascorbic acid (Olsen et al. Citation1954).
The P use efficiency (PUE) in the fertilisation treatments was calculated as follows:
The agronomic efficiency of P (AEP) in the fertilisation treatments was calculated as follows:
Fertiliser fixation rate (PFR) in the fertilisation treatments was calculated as follows:
The soil P activation rate (PAR) in the fertilisation treatments was calculated as follows:
where Labile P denotes the labile P in soil + P uptake, and Total P refers to the total P in soil + P uptake.
Plant sampling and analysis
Cottons were harvested after physiological maturity, and the growth index and yields were recorded. The plant samples were dried and digested with H2SO4 and H2O2, and vanadyl molybdate yellow colorimetric method was used determine the P concentration in the digestion solution (Song et al. Citation2017).
Statistical analysis
Significance was expressed in terms of probability (p) level. p values higher than 0.05 were considered not significant (NS). When ANOVA showed differences in response to the strategy, Duncan’s multiple range test was used to compare all possible treatment responses at p = 0.05. The test results showed the precision of the measurement results in terms of arithmetic mean and standard deviation. ANOVA was performed on SPSS 19.0 software. Redundancy analysis (RDA) and Pearson coefficient (r) were calculated and plotted in R version 4.0.3 (The R Foundation for Statistical Computing) using the ggplot2 and vegan, ggrepel, and corrplot packages. Data statistics and mapping were conducted on Microsoft Excel 2010 software and R Studio.
Results
Influence of different fertilisation treatments on soil P fraction
The P forms were significantly affected by fertilisation (). The dominant P fraction in soil was HCl-P (77.0–91.9%), followed by residue-P (10.3–10.9%), and they were the moderately labile and stable pools of P. H2O-P and NaHCO3-P accounted for 0.7% and 3.3% of TP, respectively, in the CK treatment, and they were the labile pools of P (). The effect of different fertilisation treatments was significant, and different P components changed significantly. CT treatment had almost no effect on the water-P fraction compared with OF treatment. By contrast, the NaHCO3-Pi fraction increased by 17.3%, and 56.0% at the CT and HP treatments, respectively, while the NaHCO3-Po fraction increased by 39.0% and 0.4%, respectively.
Figure 1. Percentage of phosphorus form contents in different fertilisation treatments.
Note: Data are shown as means of the percentage of different phosphorus forms in each treatment, n = 4. Means followed by the same letter are not significantly different (p ≥ 0.05) based on Tukey test for Duncan. OF, organic matter fertiliser treatment; CT, acid compost tea treatment; HP, phosphoric acid (pH = 1) treatment; CK, no fertiliser treatment. Water-P, Water-soluble phosphorus; NaHCO3-Pi, inorganic phosphorus extracted from NaHCO3; NaHCO3-Po, organic phosphorus extracted from NaHCO3; NaOH-Pi, inorganic phosphorus extracted from NaOH; NaOH-Po, organic phosphorus extracted from NaOH; HCl-P, phosphorus extracted from HCl; Residue-P, phosphorus digested from the residue.
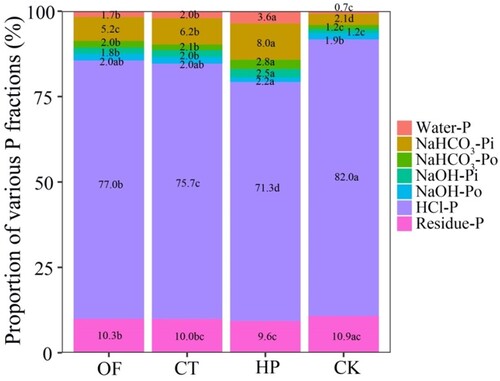
Table 2. Content of various phosphorus forms in different fertilisation treatments (mg/kg).
The HP treatment significantly changed the labile-P fractions (). HP was 61.8% higher than OF (in total) and 39.8% higher than CT. Correspondingly, the content of moderately labile-P decreased slightly (e.g. HCl-P decreased by 1.7% and 7.4% in the CT and HP treatments, respectively). This finding indicated that the dissolution and mineralisation of P possibly occurred. Acidic CT and phosphoric acid contain several H+, leading to a decrease in HCl-P content (Teng et al. Citation2020). The above analysis suggested that under the action of CT and phosphoric acid, P was transferred from stable P pools to more labile P pools.
Influence of fertilisation on soil pH and alkaline phosphatase activity and OM
The soil pH in the fertiliser treatment decreased significantly. The OF, CT, and HP treatments showed soil pH values of 1.2%, 2.3%, and 2.3% lower than the CK treatment, respectively, suggesting that the application of OF and acid could significantly reduce the pH of calcareous soil. In addition, the HP treatment could significantly reduce the pH of soil compared with OF treatment by 1.1%. All fertilisation treatments improved the activity of soil phosphatase, but the differences were NS. Compared with CK treatment, OF and CT treatments showed no significant change in the soil OM content, whereas phosphoric acid significantly reduced the soil OM by 4.4%.
Cotton growth and cumulative diagram of P content and absorption by cotton under different fertilisation treatments
Different fertilizations led to significant changes in cotton growth (). The application of CT significantly increased the plant height and number of leaves by 30.9% and 67.8% compared with CK treatment, respectively. The dry matter in the fertilisation treatments was significantly higher than that in CK treatment, but the difference between CT and HP was NS. Fertiliser application significantly increased the yield of cotton compared with CK treatment, and CT treatment significantly increased the yield by 22.9% compared with OF treatment.
Table 3. Cotton growth and yield under different fertilisation treatments.
The P concentration in cotton () was significantly affected by the content of labile-P in soil (). The P concentrations of CT and HP were significantly higher than those of CK, but the difference between CT and OF was NS. The total P accumulation of cotton was similar to the P content, and significant differences were found in the total P accumulation among all the treatments. This difference could be attributed to the accumulation of dry matter () and P concentration (), and they were both decreased by HP > CT > OF > CK.
Figure 2. Phosphorus concentration (a) and absorption (b) of cotton under different fertilisation treatments.
Note: n = 4. Vertical bars represent the average standard deviation of the means. The different colors of the column represent the nutrient uptake of different organs (stem, leaf, capsule wall, seed, and fibre, from bottom to top). Different letters indicate significant difference among treatments based on Tukey test for Duncan (p < 0.05). OF, organic matter fertiliser treatment; CT, acid compost tea treatment; HP, phosphoric acid (pH = 1) treatment; CK, no fertiliser treatment.
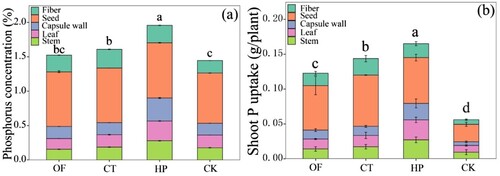
Utilisation and activation of nutrients in different fertilisation treatments
shows that the P input mainly came from fertilisation, ignoring other sources of P, such as irrigation and rainfall. In addition, without considering the leaching loss of greenhouse, only plant uptake was used to evaluate the output of P. Thus, the P input of the treatments was far greater than its yield, and the P in soil continued to accumulate and be fixed.
Table 4. P balance, PUE (%), AEP (kg/kg), PFR (%), and PAR (%) values under different treatments.
PUE is usually very low in agricultural systems, rarely more than 20% (Dobermann et al. Citation1996; Hashmi et al. Citation2017). This finding was also observed in the present study, and the same trend appeared for AEP. In the OF treatment, the fertiliser fixation rate exceeded 100%. This result did not mean that all the P in the fertiliser was fixed, because the content of available P in soil increased after fertilisation and the original labile P part of soil also transformed into stable-P (Helyar Citation1998). The CT and HP treatments significantly reduced the fixation of P (72.3% – 204.4%). OF was lower than 0 for PAR, indicating that it had no positive effect on the activation of the original P in soil. However, the application of phosphoric acid converted 4.4% of the P in soil into labile forms, suggesting that continuous application of CT may have a positive effect on reducing P fixation in calcareous soil.
Discussion
Acidic CT releases P from stable P in soil
This study showed an increase in labile-P (H2O-P and NaHCO3-P) and moderately labile-P (NaOH-P) in CT and HP phosphoric acid treatments compared with those in the OF treatment (). The labile-P in the HP treatment (pH = 1) was significantly higher than that in the OF treatment (p < 0.05), but only the NaHCO3-Pi in the CT treatment (pH = 2.7) was significantly higher than that in the OF treatment. In addition, H2O-P only slightly increased. Therefore, the more acidic the fertilisation treatment is, the better the effect on the increase in labile-P in soil. This finding was related to the H+ in CT and phosphoric acid and the decrease in soil pH (Andersson et al. Citation2015). It changed the solubility of secondary Fe, Al, and Ca-P minerals in soil and changed the adsorption capacity of soil (Sato et al. Citation2005). However, the HCl-P in the CT and HP treatments were significantly lower than that in the OF treatment (p < 0.05). Therefore, the increase in labile P in the two treatments was likely from the dissolution of HCl-P. These findings indicated that HCl-P was the source of unstable P in the CT and HP treatments.
H+ in composted tea is the main factor causing changes in P composition
The results of redundant analysis (RDA) for different P components and soil physicochemical properties of the four treatments are shown in . The main canonical axis explained 87% of the P pool variation. This axis was mainly controlled by changes in OM and pH, while the second axis explained only 1.7% of the changes in the P pools.
Figure 3. Results of redundancy analysis for P fractions and soil physicochemical properties in different fertilisation treatments.
Note: n = 16. pH, soil pH; OM: organic matter content; TN, soil total nitrogen; TP, soil total phosphorus; APT, soil alkaline phosphatase activity. OF, organic matter fertiliser treatment; CT, acid compost tea treatment; HP, phosphoric acid (pH = 1) treatment; CK, no fertiliser treatment. Water-P, water-soluble phosphorus; NaHCO3-Pi, inorganic phosphorus extracted from NaHCO3; NaHCO3-Po, organic phosphorus extracted from NaHCO3; NaOH-Pi, inorganic phosphorus extracted from NaOH; NaOH-Po, organic phosphorus extracted from NaOH; HCl-P, phosphorus extracted from HCl; Residue-P, phosphorus digested from the residue.
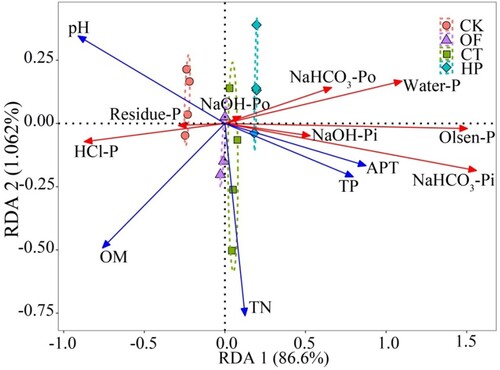
CT had the same composition (N, P, K, and OM) as OF except for lower pH (2.7). However, CT treatment significantly increased the content of labile-P in soil, indicating that the lower pH of fertilisers caused the change in P forms in soil. Phosphoric acid has a lower pH (1) than CT, but it has no OM input. However, the content of labile-P in the HP treatment still significantly increased (), indicating that the acid was the main factor affecting the soil P form in CT. Besides, RDA analysis showed that the interpretation rates of soil pH and OM were 70.1% and 46.7%, respectively. This finding revealed that the pH of CT was the main factor that caused the transformation of P in soil compared with OM, because a large amount of H+ promoted the dissolution of Ca-P in calcareous soil and reduced the formation of less soluble Ca phosphate (Eissa Citation2019), thereby increasing the content of labile-P in soil (Yan et al. 2016). Alkaline phosphatase was generally considered to be related to the increase in Po. In the present study, fertilisation significantly increased the activity of alkaline phosphatase ((b)), and pH was negatively correlated with the alkaline phosphatase activity in soil. However, this activity almost had no association with Po (). In addition, an increase in NaHCO3-Po and NaOH-Po was found in CT and HP treatments, and this result may increase the mineralisation of Po compounds by the soil microbial community (Fransson and Jones Citation2007). A significant negative correlation was observed between OM and labile-P (p < 0.05). This result was not consistent with the findings that OMs could increase the availability of P in P-fixing soils (Guppy et al. Citation2005; Yang et al. Citation2019), because the application of phosphoric acid reduced the content of OM in soil ((c)) and increased labile-P. In addition, the effect of acid on P was far greater than the desorption of OM on P solubility. Therefore, compared with OM, the acid in CT was the main factor affecting the forms of soil P.
Figure 4. Soil pH (a), alkaline phosphatase activity (b), and organic matter (c) under different fertilisation treatments.
Note: n = 4. Vertical bars represent the average standard deviation of the means. Values followed by different letters indicate significant difference among treatments (p <0.05). OF, organic matter fertiliser treatment; CT, acid compost tea treatment; HP, phosphoric acid (pH = 1) treatment; CK, no fertiliser treatment.
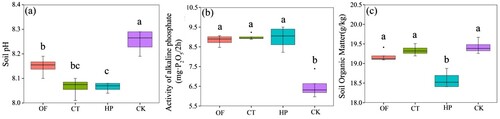
Acidic CT could reduce soil fixation of P in fertiliser
Rhizosphere acidification is an important mechanism for plants to increase the availability of P in soil solutions (Mihoub et al. Citation2017). When phosphoric acid and acidic CT were applied, a large amount of H+ was added to soil, and the soil pH decreased by 0.19 compared with that in CK, inevitably causing P conversion (Sun et al. Citation2020). In the present research, a part of P, which was not absorbed by cotton, applied to soil in the OF treatment transformed into stable-P, leading to reduced PUE (), while the application of CT increased the content of available P in soil (H2O-P + NaHCO3-P) by 13.2%. PFR was also reduced by 42.0%, indicating that the OM in the fertiliser had a slight effect on P fixation and activation, and the pH of the fertiliser had a greater contribution. In addition, the HP treatment with no OM and lower pH had higher P utilisation efficiency and lower P fixation rate than the CT treatment, while the PAR was significantly higher than that in CT, also indicating that low pH was the main factor affecting P activation by fertilisers.
Although the application of CT had almost no effect on the activation of P in soil (PAR = −0.6%), it significantly reduced the fixation rate of P (41.9%) compared with that of OF, indicating its positive effect on keeping the availability of nutrients from the fertilisers and that adding acid could increase the available P in soil and reduce P fixation (Guertal and Howe Citation2013; Akhtar et al. Citation2016). RDA analysis showed that soil pH was negatively correlated with soil H2O-P, NaHCO3-P, and NaOH-P and significantly and positively correlated with HCl-P and residue-P (0.72 and 0.53, respectively). This finding indicated that lowering soil pH could keep the P from fertilisers in labile-P and NaOH-P and the P activated by acid in HP treatment mainly originated from the dissolution of HCl-P and residue-P in soil. This was also consistent with the report that the addition of acid could increase the available P in soil and reduce P fixation (Guertal et al. 2013; Akhtar et al. Citation2016).
Other substances in acidic CT may promote the growth of cotton and increase yield
Soil pH decreased after CT and HP treatments, and the concentration and accumulation of P by cotton were significantly higher than those after CK treatment (p < 0.05), indicating that the increase in available P content led to an increase in P absorption by cotton (). In addition, the P utilisation efficiency and yield significantly improved (p < 0.05). Correlation analysis () also showed that the P content and total accumulation of P in cotton had a significant negative correlation with soil pH (0.42 and 0.62, respectively) and a significant positive correlation with soil labile-P (0.95–0.77). This finding agreed with a previous report indicating that decreased rhizosphere soil pH resulted in an increase in available P concentration in soil, P uptake, and aboveground biomass of cotton (Wang et al. Citation2018; Shen et al. Citation2019). However, compared with HP treatment, CT treatment showed increased yield and agronomic efficiency of cotton by 9.4% and 18.0%, respectively (). This finding indicated that compared with phosphoric acid, the other ingredients (e.g. OM and trace elements) in CT may have a positive effect on the absorption and transport of P in cotton, except for increasing the concentration of labile P by decreasing the soil pH (Wandruszka Citation2006). Therefore, the application of acidic CT has a great potential for the activation and utilisation of soil stable P in calcareous soils with high total P content but low available P.
Figure 5. Pearson correlation coefficients (r values) between different phosphate fractions and soil properties and phosphorus uptake by cotton in fertilisation treatments.
Note: n = 12 (OF, CT, and HP);*, p < 0.05; **, p < 0.01; ***, p < 0.005. pH, soil pH; OM, soil organic matter; PC, P concentration in Plants; PA, P accumulation in plants. OF, organic matter fertiliser treatment; CT, acid compost tea treatment; HP, phosphoric acid (pH = 1) treatment; CK, no fertiliser treatment. Water-P, water-soluble phosphorus; NaHCO3-Pi, inorganic phosphorus extracted from NaHCO3; NaHCO3-Po, organic phosphorus extracted from NaHCO3; NaOH-Pi, inorganic phosphorus extracted from NaOH; NaOH-Po, organic phosphorus extracted from NaOH; HCl-P, phosphorus extracted from HCl; Residue-P, phosphorus digested from the residue.
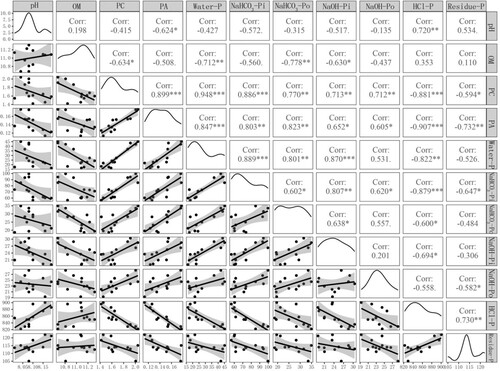
The results showed that the application of CT could reduce soil pH and increase the content of labile-P in soil. In addition, the H+ contained in CT was the main factor causing the change in P composition, while the contribution of OM was almost negligible. H+ not only could reduce the soil P fixation in fertilisers but also transform a part of HCl-P into labile-P in soil. CT also increased the accumulation of P in cotton and improve the utilisation efficiency of P. In addition, soil pH was the main factor affecting the availability of P in calcareous soil, and acidic CT was the optimal fertiliser for improving the utilisation efficiency of P in calcareous soil. Further studies should focus on the effect of long-term application of CT on the transformation of P pools in soil and the effect of soil microbial environment and physical structures.
Acknowledgements
We thank to Prof. Junhua Li and Prof. Changzhou Wei for their valuable help, and Key Laboratory of Oasis Eco-agriculture provide the laboratories and instruments.
Disclosure statement
No potential conflict of interest was reported by the author(s).
Additional information
Funding
Notes on contributors
Tong Luo
Tong Luo, is a Ph.D. from Shihezi University College of Agriculture. His research interests include new organic fertilizers, crop fertilization technology, and efficient use of nutrients in cotton.
Yuanji Zhu
Yuanji Zhu, is a undergraduate of Shihezi University College of Agriculture, His research interests include drip irrigation fertilization technology, field nutrient management, etc.
Weidan Lu
Weidan Lu, is a postgraduate of Shihezi University's School of Agriculture. Her research interests include organic substitution, weight loss research on wheat, field nutrient management, etc.
Lili Chen
Lili Chen, is a postgraduate student at the Agricultural College of Shihezi University. Her research interests include the remediation of heavy metal pollution in the soil, the activation of cadmium by organic acids, etc.
Tao Min
Tao Min, is a postgraduate of Shihezi University's School of Agriculture. His research interests include the restoration of cadmium pollution in cotton fields, the influence of soluble organic matter on cadmium, etc.
Junhua Li
Junhua Li, is a professor at the Agricultural College of Shihezi University. His research interests include new fertilizers and modern fertilization techniques, organic fertilizers and soil fertility, etc.
Changzhou Wei
Changzhou Wei, is a professor at the Agricultural College of Shihezi University. His research interests include plant nutrition, modern fertilization technology, agricultural resource management and agricultural informatization.
References
- Akhtar M, Yaqub M, Naeem A, Ashraf M, Hernandez VE. 2016. Improving phosphorus uptake and wheat productivity by phosphoric acid application in alkaline calcareous soils. J Sci Food Agric. 96:3701–3707.
- Andersson KO, Tighe MK, Guppy CN, Milham PJ, McLaren TI. 2015. Incremental acidification reveals phosphorus release dynamics in alkaline vertic soils. Geoderma. 259-260:35–44.
- Bao SD. 2010. Soil agrochemical analysis, 3rd ed. Beijing: China Agricultural Press. (in chinese).
- Braschi I, Ciavatta C, Giovannini C, Gessa C. 2003. Combined effect of water and organic matter on phosphorus availability in calcareous soils. Nutr Cycl Agroecosyst. 67:67–74.
- Brucker E, Kernchen S, Spohn M. 2020. Release of phosphorus and silicon from minerals by soil microorganisms depends on the availability of organic carbon. Soil Biol Biochem. 143:107737.
- Debicka M, Kocowicz A, Weber J, Jamroz E. 2016. Organic matter effects on phosphorus sorption in sandy soils. Arch Agron Soil Sci. 62:840–855.
- De Corato U. 2020. Agricultural waste recycling in horticultural intensive farming systems by on-farm composting and compost-based tea application improves soil quality and plant health: a review under the perspective of a circular economy. Sci Total Environ. 738:1–22.
- Dobermann A, Cassman KG, Cruz PCS, Adviento MA, Pampolino MF. 1996. Fertilizer inputs, nutrient balance, and soil nutrient-supplying power in intensive, irrigated rice systems. II: effective soil K-supplying capacity. Nutr Cycl Agroecosyst. 46:11–21.
- Eissa MA. 2019. Efficiency of P fertigation for drip-irrigated potato grown on calcareous sandy soils. Potato Res. 62:97–108.
- Fransson AM, Jones DL. 2007. Phosphatase activity does not limit the microbial use of low molecular weight organic-P substrates in soil. Soil Biol Biochem. 39:1213–1217.
- Giles CD, Cade-Menun BJ, Liu CW, Hill JE. 2015. The short-term transport and transformation of phosphorus species in a saturated soil following poultry manure amendment and leaching. Geoderma. 257-258:134–141.
- Gross A, Arusi R, Fine P, Nejidat A. 2008. Assessment of extraction methods with fowl manure for the production of liquid organic fertilizers. Bioresour Technol. 99:327–334.
- Guertal EA, Howe AJ. 2013. Influence of phosphorus-solubilizing compounds on soil P and P uptake by perennial ryegrass. Biol Fertil Soils. 49:587–596.
- Guppy CN, Menzies NW, Moody PW, Blamey FPC. 2005. Competitive sorption reactions between phosphorus and organic matter in soil: a review. Soil Res. 43:189–202.
- Hashmi Z, Ul H, Khan MJ, Akhtar M, Sarwar T, Khan MJ. 2017. Enhancing phosphorus uptake and yield of wheat with phosphoric acid application in calcareous soil. J Sci Food Agric. 97:1733–1739.
- Hedley MJ, Stewart JWB, Chauhan BS. 1982. Changes in inorganic and organic soil phosphorus fractions induced by cultivation practices and by laboratory incubations. Soil Sci Soc Am. J. 46:970–976.
- Helyar KR. 1998. Efficiency of nutrient utilization and sustaining soil fertility with particular reference to phosphorus. Field Crop Res. 56:187–195.
- Ikram W, Akhtar M, Morel C, Rizwanm M, Ali S. 2019. Phosphate fertilizer premixing with farmyard manure enhances phosphorus availability in calcareous soil for higher wheat productivity. Environ Sci Pollut Res. 26:32276–32284.
- Jackson ML. 1973. Soil chemical analysis. 2nd ed. Englewood: Prentice-Hall.
- Jordán MM, Almendro-Candel MB, Navarro-Pedreño J, Pardo F, García-Sánchez E, Bech J. 2020. Bioavailability, mobility and leaching of phosphorus in a Mediterranean agricultural soil (ne Spain) amended with different doses of biosolids. Environ Geochem Health. 1:1–8.
- Kim MJ, Shim CK, Kim YK, Hong SJ, Park JH, Han EJ, Kim JH, Kim SC. 2015. Effect of aerated compost tea on the growth promotion of lettuce, soybean, and sweet corn in organic cultivation. Plant Pathol. J. 31:259–268.
- Li XX, Wang XH, Shi XY, Wang Q, Li XL, Zhang SW. 2020. Compost tea-mediated induction of resistance in biocontrol of strawberry verticillium wilt. J Plant Dis Prot. 127:257–268.
- Mihoub A, Bouhoun MD, Naeem A, Saker ML. 2017. Low-molecular weight organic acids improve plant availability of phosphorus in different textured calcareous soils. Arch Agron Soil Sci. 63:1023–1034.
- Miller BW, Fox TR. 2011. Long-term fertilizer effects on oxalate-desorbable phosphorus pools in a typic paleaquult. Soil Sci Soc Am J. 75:1110–1116.
- Oburger E, Jones DL, Wenzel WW. 2011. Phosphorus saturation and pH differentially regulate the efficiency of organic acid anion-mediated P solubilization mechanisms in soil. Plant Soil. 341:363–382.
- Ohno T, Hoskins BR, Erich MS. 2007. Soil organic matter effects on plant available and water soluble phosphorus. Biol Fertil Soils. 43:683–690.
- Olsen SR, Cole CV, Watanabe FS, Dean LA. 1954. Estimation of available phosphorus in soils by extraction with sodium bicarbonate. USDA Circ. 939:1–19.
- Oral A, Uygur V. 2018. Effects of low-molecular-mass organic acids on P nutrition and some plant properties of Hordeum vulgare. J Plant Nutr. 41:1482–1490.
- Pizzeghello D, Berti A, Nardi S, Morari F. 2011. Phosphorus forms and P-sorption properties in three alkaline soils after long-term mineral and manure applications in north-eastern Italy. Agric Ecosyst Environ. 141:58–66.
- Samet M, Karray F, Mhiri N, Kamoun L, Sayadi S, Gargouri-Bouzid R. 2019. Effect of phosphogypsum addition in the composting process on the physico-chemical proprieties and the microbial diversity of the resulting compost tea. Environ Sci Pollut Res. 26:21404–21415.
- Sato S, Solomon D, Hyland C, Ketterings QM, Lehmann J. 2005. Phosphorus speciation in manure and manure-amended soils using XANES spectroscopy. Environ Sci Technol. 39:7485–7491.
- Sharpley A, Moyer B. 2000. Phosphorus forms in manure and compost and their release during simulated rainfall. J Environ Qual. 29:1462–1469.
- Shen Y, Duan YH, McLaughlin N, Huang SM, Guo DD, Xu MG. 2019. Phosphorus desorption from calcareous soils with different initial Olsen-P levels and relation to phosphate fractions. J Soils Sediments. 19:2997–3007.
- Song K, Xue Y, Zheng X, Lv W, Qiao H, Qin Q, Yang J. 2017. Effects of the continuous use of organic manure and chemical fertilizer on soil inorganic phosphorus fractions in calcareous soil. Sci Rep. 7:1164.
- Sun BR, Gao YZ, Wu X, Ma HM, Zheng CC, Wang XY, Zhang HL, Li ZJ, Yang HJ. 2020. The relative contributions of pH, organic anions, and phosphatase to rhizosphere soil phosphorus mobilization and crop phosphorus uptake in maize/alfalfa polyculture. Plant Soil. 447:117–133.
- Teng ZD, Zhu J, Shao W, Zhang KY, Li M, Whelan MJ. 2020. Increasing plant availability of legacy phosphorus in calcareous soils using some phosphorus activators. J Environ Manage. 256:109952.1–109952.9.
- Walkley A, Black IA. 1934. An examination of the Degtjareff method for determining soil organic matter and a proposed modification of the chromic acid titration method. Soil Sci 37:29–38.
- Wandruszka RV. 2006. Phosphorus retention in calcareous soils and the effect of organic matter on its mobility. Geochem Trans. 7:1–8.
- Wang XX, Liu SL, Zhang SM, Li HB, Baidengsha M, Feng G, Rengel Z. 2018. Localized ammonium and phosphorus fertilization can improve cotton lint yield by decreasing rhizosphere soil pH and salinity. Field Crop Res. 217:75–81.
- Yan ZJ, Chen S, Dari B, Sihi D, Chen Q. 2018. Phosphorus transformation response to soil properties changes induced by manure application in a calcareous soil. Geoderma. 322:163–171.
- Yang XY, Chen XW, Yang XT. 2019. Effect of organic matter on phosphorus adsorption and desorption in a black soil from Northeast China. Soil Tillage Res. 187:85–91.