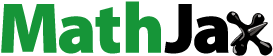
ABSTRACT
The application of insufficient amounts of mineral fertiliser, coupled with unreliable precipitation, has caused a drastic reduction in the yield of sorghum in the smallholder-farming areas of Zimbabwe. This calls for innovative interventions to improve production under changing climatic conditions. This study evaluated the effect of contour-based rainwater-harvesting methods and the use of cattle manure + N nutrient amendment on sorghum yield. A split-split plot experiment was conducted where rainwater-harvesting practice, sorghum variety, cattle manure + N and distance from rainwater-harvesting structure were treatment factors. The results showed that the yield of sorghum was significantly higher under the tied contour and infiltration pits than standard contour at all distances from rainwater-harvesting practices and seasons. In all seasons sorghum varieties Macia and Sc Sila showed higher yield under the tied contour and infiltration pits than standard contour. However, regardless of the distance from rainwater-harvesting practice and season the sorghum variety, Macia showed a higher grain yield than Sc Sila. At each incremental level of N application to cattle manure, Macia had a higher grain yield than Sc Sila, and no significant grain yield benefits were shown in each variety at N application to cattle manure above 50 kg N ha−1.
Introduction
Smallholder agriculture faces significant challenges in increasing food production without significantly increasing the area under cultivation (Stevenson et al. Citation2013). Even the production of crops traditionally recommended for semi-arid regions, such as sorghum, has been on the decline. This decline in yields has been attributed to the reduction in soil fertility and droughts as a result of climate change (Nyamangara et al. Citation2014). In addition, poor agronomic practices, particularly inadequate application of both mineral and organic fertilisers, have also resulted in drastic reduction in yields (Chianu et al. Citation2012). However, the use of livestock manure remains an integral component of soil fertility management in southern Africa, with a potential to increase crop productivity in the smallholder-farming sector. It is widely accepted that the addition of livestock manure is essential to maintain soil health (Katyal Citation2000). Occasionally the positive effects of using livestock manure are limited due to the poor quality of organic materials used by smallholder farmers and also limited moisture conditions as a result of unreliable rainfall.
Organic inputs are a major source of energy and nutrients for soil microbial communities, which promote soil aggregation and nutrient buffering capacity (Lal et al. Citation2007). However, the use of organic materials to increase nutrient reserve requires large amounts of annual additions. Rufino et al. (Citation2011) suggested that around 7–10 t ha−1 year−1 is needed to maintain soil organic carbon and sufficient nutrient level for crop production. In addition, it is difficult to build or maintain adequate soil organic matter levels for nutrient provision under smallholder farming conditions due to competing issues such as livestock and firewood (Mhlanga and Muoni Citation2014). Moreover, crop responses to manure application observed in the farmers’ fields are highly variable because of the differences in the chemical composition of manure, rates and frequency of manure application (Chivenge et al. Citation2011). The nutrient contents of manure differ because of variations in animal diet and ways in which manure is collected and stored. Many crop residues and animal manure are of low quality as they fall below critical nitrogen contents of 1.8−2.0%; hence, they immobilise nitrogen temporarily, potentially exacerbating the nitrogen deficiency (Nyamangara et al. Citation2005).
The addition of low-quality organic inputs may, over time, increase soil organic carbon, but without necessarily increasing the productivity of the cropping systems (Rufino et al. Citation2011). Low-quality organic materials do not provide soluble carbon and nitrogen to enhance the soil microbial activity and may immobilise N, markedly reducing crop production compared to levels before the addition of the organic materials (Nyamangara et al. Citation2005). To address and offset these potentially negative effects, an option can be to combine organic manures with mineral nitrogen fertiliser: integrated nutrient management (INM).
An integrated nutrient management system can improve soil fertility status, water infiltration, and nutrient availability (Lal Citation2004; Mahajan et al. Citation2008). Integrated nutrient management strategies should build soil carbon and enhance biological processes to increase nutrient availability and cycling efficiency. Research findings have shown that neither mineral fertilisers nor organic sources alone can result in sustainable productivity (Godara et al. Citation2012). A combination of both inorganic and organic fertilisers is the best remedy for soil fertility (Chivenge et al. Citation2011; Woldesenbet and Tana Citation2014). The inorganic fertiliser provides readily available nutrients and the organic fertiliser mainly increases soil organic matter and improves soil structure and buffering capacity of the soil (Godara et al. Citation2012). This, in turn, will narrow the C:N ratio and increase soil microbial activity, thereby increasing nutrient availability and soil buffering capacity. The application of livestock manure, in combination with small amounts of mineral nitrogen fertiliser inputs, can enhance nitrogen availability and nutrient cycling efficiency (Chivenge et al. Citation2011). Besides improving the physical and chemical properties of soils integrated nutrient management does not have only additive effects but real interaction, which significantly affects crop yield and water-use efficiency (Ouedraogo and Mando Citation2010).
Despite several researchers, such as Angachew (Citation2009), Chivenge et al. (Citation2011), Farah et al. (Citation2014), Kugedera et al. (Citation2018), Kugedera et al. (Citation2020), Mahajan et al. (Citation2008), Mahinda et al. (Citation2018) and Masvaya et al. (Citation2017), demonstrate the beneficial effect of integrated nutrient management in mitigating the deficiency of nutrients. The productivity of INM in semi-arid regions is lowered by low soil moisture status due tounpredictable rainfall. Hence, there is a need to identify soil water management practices and inorganic nitrogen fertiliser application rates that are agro-ecologically specific. Contour-based rainwater-harvesting practices can offer an opportunity to enhance the productivity of integrated nutrient management, but their contribution has not been fully exploited (Nyamadzawo et al. Citation2013). Information regarding the effects of contour rainwater-harvesting and cattle manure + N fertility management on sorghum productivity in the smallholder-farming system is still limited. Thus, the objective of this study was to determine the effect of two contour-based rainwater-harvesting techniques (tied contour and infiltration pits) and the use of cattle manure + N on sorghum production in smallholder semi-arid farming areas.
Materials and methods
Study site
The experiment was conducted in Mt Zonwe smallholder-farming area of Mutare district in Zimbabwe (19° 11ʹ 30″ S 32° 03ʹ 28″ E 835 m above the sea level) during 2016/17 to 2018/19 cropping seasons. The area is located in the agro-ecological region IV, characterised by a low and erratic rainfall. The rainfall pattern is unimodal, with October–March rain season receiving an annual average rainfall <650 mm on a long-term basis and a mean annual temperature of 27°C. The soils are chiefly sand on a general slope of 3%, and soils are inherently deficient in nitrogen and phosphorus. The predominant cropping pattern is mainly monoculture of sorghum, millets and cotton.
Soil sampling and analysis
A total of 15 soil samples were collected prior to planting in the experimental field, measuring 90 × 45 m from a depth of 0–30 cm in a zig-zig manner using a soil auger. A composite sample was prepared for analysis to determine the physico-chemical properties of the experimental site. The composited soil sample was air-dried, ground and sieved to pass with a 2 mm sieve. Total nitrogen was determined following the Kjeldahl procedure, soil pH by the CaCl2 method, organic carbon by the wet digestion method, available phosphorous by the Olsen method and soil texture by the Bouyoucos Hydrometer method. Summary data for soil physico-chemical characteristics of the study site are presented in .
Table 1. Physico-chemical characteristics of soil from experimental sites.
Experimental design
A split-split plot experimental design replicated three times over three years was used. The experimental units consisted of three successive contour lines spaced at 15 m interval, each measuring 90 m long. The contour length was divided into three 30 m of rainwater-harvesting practices: tied contour, infiltration pits and standard contour (). The rainwater-harvesting practices were the main plot factor. The tried contour was made of cross ties made at intervals of 5 m along the contour, creating miniature dams measuring 5 m long × 0.5 m wide × 0.5 m deep. Infiltration pits were dug along the contour channel measuring 2 m long × 0.5 m wide × 0.5 m deep spaced at intervals of 0.5 m along the contour. A 30 m length standard contour was left as a control. A distance of 2 m wide was left between each rainwater-harvesting practice along the contour marking the end of a water harvesting practice. Sub-plot factors consisted of two sorghum varieties, Sc Sila and Macia, grown under each rainwater-harvesting practice. Each sub-plot factor measured 15 m long and 4.5 m wide, where cattle manure + N rates were used as sub-sub-plot factors. The sub-sub-plot factor of cattle manure + N measured 2 m long × 4.5 m wide replicated three times down the slope within each rainwater-harvesting practice. The replications were categorised into three distances from rainwater-harvesting practice, namely 0–5, 5−10 and 10−15 m measured from the centre of the rainwater-harvesting structure. Sub-sub-plot treatment factor of cattle manure (t ha−1) + N (kg N ha−1) was as follows 5 + 0 N; 5 + 50 N; 5 + 70 N; 5 + 100 N; 5 + 130 N and 5 + 170N kg N ha−1 ().
Experimental procedure
The experimental field was ploughed using an ox-drawn plough to a depth of 20 cm in all the seasons and planted in December after receiving adequate rainfall of the season. Furrows were opened by an ox-drawn plough spaced at 0.75 m in each treatment and cattle manure was spread along the furrow as the basal organic fertiliser at 5 t ha−1. Cattle manure was analysed before applying to determine nutrient content (). Sorghum varieties, Macia (open pollinated) and Sc Sila (hybrid), were planted into the furrows at a seed rate of 12 kg ha−1 . Two weeks later crop emergence thinning was done, leaving individual sorghum plants spaced at 10 cm along the furrow, giving a target plant population of 1,33,333 plants ha−1. The nitrogen levels of 0; 50; 70; 100; 130 and 170 kg N ha−1 were randomly applied as sub-sub-plot factors in each sorghum variety using ammonium nitrate (34.5%) top dressing fertiliser after 5 weeks of crop emergence. A 0 kg N ha−1 treatment was left as a control in each sub-plot factor. Weed control was done using hand hoes in all the plots as weeds emerged. Incidence of fall army worm (Spodoptera frugiperda) was recorded and controlled using Ecoterex (Deltamethrin and Pirimiphos methyl) pesticide, and bird scaring was erected at the heading stage to harvest maturity.
Table 2. Nutrient composition of cattle manure applied.
Rainfall was recorded by the farmer using a rain gauge installed at the experimental site. The total rainfall received during the growing seasons ranged from 780 to 964 mm above the long-term average of 650 mm for the agro-ecological region (IV). The highest rainfall was recorded in 2016/17, while the 2018/19 season had the least rainfall total (). Despite high rainfall during the seasons, intermittent dry spell periods were experienced during the season with an average of 16 days of dry spell being experienced in January and February.
Data collection
Monthly soil moisture measurements were done using the gravimetric method in the field under each rainwater-harvesting practice. Soil moisture was assessed to a depth of 30 cm at varying distances of 0–5, 5−10 and 10−15 m from the rainwater-harvesting practices. Sorghum heads were cut at harvest maturity and sun-dried for threshing. Grain yield (kg ha−1) was recorded after harvesting from the net plot of 1 m × 1 m = 1 m2. Grain yield was adjusted to 13.5% moisture content after measuring moisture using a digital moisture meter (Dickey-john model) and converted to t ha−1 for statistical analysis.
where M is the measured moisture content in grain and D is the designated moisture content (13.5%).
Data analysis
Soil moisture content and sorghum grain yield data were subjected to the statistical analysis of variance for split-split plot design using GenStat statistical package. The least significant difference test at 0.05 was performed to separate significant treatment means.
Results
Soil moisture content
Significant (p < 0.05) soil moisture reduction was observed as the distance from rainwater-harvesting practices increased. Soil moisture conditions under the rainwater-harvesting practices at distances of 0−5 and 5−10 m significantly (p < 0.05) decreased in the order tied contour > Infiltration pits > standard contour, while there was no soil moisture difference between the rainwater-harvesting practices at 10−15 m distance from the rainwater-harvesting practice (). Soil moisture decreased in the order 0−5 > 5−10 > 10−15 m under tied contour and infiltration pits, while there were no soil moisture differences between the distances from rainwater-harvesting practices under the standard contour.
Gain yield
The results showed a significant effect (p < 0.05) of all the main treatments on sorghum yield (). However, significant interaction effects (p < 0.05) on sorghum yield were observed among the treatments and used to explain the yield differences.
Table 3. Effects of Rainwater-harvesting, sorghum variety, cattle manure + N, distance from RWH practice and season on sorghum grain yield.
Effect of rainwater-harvesting × distance from rainwater-harvesting practice × season on sorghum grain yield
A significant interaction effect (p < 0.05) of rainwater-harvesting practice × distance from rainwater-harvesting × season was shown on the sorghum grain yield. Tied contour and infiltration had no yield difference with significantly higher yield than standard contour at 0−5 m distance from rainwater-harvesting practice in the 2016/17 season (). As the distance from rainwater-harvesting practice increases to 5−10 m in the same season, a different trend was shown with a tied contour, exhibiting higher yield, while infiltration pits and standard contour had no yield differences. Variable yields were shown in the 2016/17 season under the rainwater-harvesting practices further away (10−15 m) from the rainwater-harvesting practice. In the 2017/18 season tied contour and infiltration pits had no yield difference with significantly higher yield than the standard contour at each distance from the rainwater-harvesting practice (). In the 2018/19 season, at 0–5 m distance from rainwater-harvesting practice, sorghum yield significantly decreased under the rainwater-harvesting practices in the order tied contour > infiltration pits > standard contour. When the distance from rainwater-harvesting practice was >5 m tied contour showed higher yield while infiltration pits and standard contour had no yield differences (). However, the standard contour had consistently low yield at each distance from rainwater-harvesting practice in all seasons compared with tied contour and infiltration pits.
Effect of rainwater-harvesting × sorghum variety × season on sorghum grain yield
The influence of rainwater-harvesting practice on yield varied with sorghum variety and season. Tied contour and infiltration pits had no yield difference in 2016/17 and 2017/18 under sorghum variety Macia, while in 2018/19 the yield response to rainwater-harvesting decreased in the order tied contour > infiltration pits > standard contour (). Under sorghum variety Sc Sila, tied contour had significantly higher yield than infiltration pits and standard contour in the 2016/17 season. Tied contour and infiltration pits had no yield difference in the 2017/18 season, with significantly higher yields than standard contour in the same variety. The effect of rainwater-harvesting practice on yield was not significant in the 2018/19 season under sorghum variety Sc Sila. The standard contour showed significantly (p < 0.05) low grain yield across all seasons in the same sorghum variety, and the yield of Macia was generally higher than Sc Sila in each rainwater-harvesting practices across all seasons.
Effect of sorghum variety × distance from rainwater-harvesting practice × season on sorghum grain yield
Sorghum variety × distance from rainwater-harvesting practice × season had a significant interaction effect on yield (p < 0.05). Regardless of the season and distance from rainwater-harvesting practice sorghum variety, Macia showed higher yield than Sc Sila at each distance from rainwater-harvesting practice except in the 2016/17 season where there was no yield difference at 10−15 m distance from rainwater-harvesting practice (). The yield of Macia at each distance from rainwater-harvesting practice was in the range of 0.96–2.4 t ha−1 while the yield of Sc Sila ranged from 0.36 to 0.99 t ha−1 across the treatment combinations.
Effect of sorghum variety x fertility amendment (cattle manure + N) on sorghum grain yield
Significant interactive effects of variety and cattle manure + N on yield (p < 0.05) were observed. Cattle manure with no N had a low yield in both sorghum varieties compared with cattle manure + N at application rate above 5 t ha−1 cattle manure +50 kg N ha−1 (). Sorghum variety Macia had a higher yield than Sc Sila at each incremental level of N application under the integrated nutrient management system. However, the addition of nitrogen > 50 kg N ha−1 to 5 t ha−1 of cattle manure resulted in no yield benefit in both varieties and there was a strong correlation in each sorghum variety between fertility management (cattle manure + N) and yield.
Discussion
Rainwater-harvesting using tied contour and infiltration pits improved soil moisture compared to the standard contour (). Significantly higher moisture benefits shown by tied contour and infiltration pits at a distance from rainwater-harvesting practices up to 10 m were attributed to their ability to collect water, making it available to crops. The standard contour had low moisture at all distances due to water loss through run-off. The results are in tandem with Mhizha and Ndiritu (Citation2013), who reported a higher moisture content under rainwater-harvesting practises compared to the conventional farming system. A significant gradual decrease in moisture content was shown by the rainwater-harvesting practices (). This was probably due to water interception and the resistive nature of soil particles to the lateral flow of water, resulting in differential moisture content with an increase in distance from rainwater-harvesting practices (Mhizha and Ndiritu Citation2013; Mupangwa et al. Citation2012).
Significantly higher sorghum grain yield was attained under tied contour and infiltration pits at all distances and most seasons, while the standard contour had a low yield at each distance in all seasons (). This was attributed to greater water storage capacity shown by the tied contour and infiltration pits, as evidenced by a higher volumetric water content than the standard contour (). Water collected by the rainwater-harvesting practices infiltrates the soil, increasing subsurface soil water storage, thereby allowing crops to evade the dry spell period of the season. Similar findings were reported by Mandumbu et al. (Citation2020), Mhizha and Ndiritu (Citation2013) and Nyagumbo et al. (Citation2019), who reported the efficiency of rainwater-harvesting practices in concentrating water to the root zone through lateral flow, improving water availability and yield. The low sorghum grain yield in the standard contour at each distance and season () was due to low water retention (). Similar results were reported by (Nyamadzawo et al. Citation2013), who found 50% water loss in standard contours.
The grain yield of sorghum variety Macia and Sc Sila was improved by tied contour and infiltration pits rainwater-harvesting practices compared with the standard contour in all the seasons except in the 2018/19 season where there was no significant yield difference under Sc Sila (). Improved crop yields in different rainwater-harvesting practices and crops were demonstrated Mandumbu et al. (Citation2020) and Mupangwa et al. (Citation2016). However, the yield of sorghum variety Macia was higher than Sc Sila in each rainwater-harvesting practice (). The difference in grain yielding ability shown by the sorghum varieties Macia and Sc Sila under the rainwater-harvesting practice across seasons may be explained by their differential response to moisture due to genetic differences among the varieties (Hadebe et al. Citation2017; Purushothaman et al. Citation2016).
Regardless of distance from rainwater-harvesting practice and season sorghum variety Macia had a significantly higher yield than Sc Sila (). Given the low amount of rainfall received in sorghum growing areas and the erratic nature of rainfall, the sorghum variety Macia may be an adapted sorghum variety to the semi-arid farming environment, while Sc Sila proved to be less adapted. This confirms the importance of varietal selection to realise the full benefits of rainwater-harvesting in semi-arid farming environments (Singh Citation2017). In Hadebe et al. (Citation2017) farmers indicated that an ideal sorghum variety must mature within sixty to ninety days. The sorghum variety Macia is an open-pollinated variety resilient to semi-arid conditions compared to Sc Sila, a hybrid variety that showed no resilience to the prevailing environmental conditions. This concurs with the findings by Li et al. (Citation2010), Srinivasarao et al. (Citation2014) and Mitran et al. (Citation2016) who concluded that the use of adapted sorghum varieties improves grain yield and facilitates the translocation of nutrients to the economic part of the crop, thus increasing the efficiency of the applied nutrients (Ghosh et al. Citation2015).
The addition of mineral nitrogen fertiliser to cattle manure increased sorghum yield; however, there was a significant interactive effect of sorghum variety and cattle manure + N nutrient amendment on grain yield (). The greater grain yield observed in sorghum variety Macia than Sc Sila at each cattle manure + N nutrient amendment was probably due to varietal differential response to nutrient uptake and utilisation due to genetic differences. Yield differences between 5 t ha−1 (41 kg ha−1 total N) cattle manure with no N applied and treatments with cattle manure + N applied indicates the importance of nitrogen addition to cattle manure in both varieties (). The results concur with Mahinda et al. (Citation2018), where the addition of Nitrogen to farmyard manure resulted in a remarkable increase in sorghum yield in the above-average rainfall season, while Nyamangara et al. (Citation2003) and Nyamangara et al. (Citation2005) reported higher nitrogen uptake by maize under cattle manure and mineral nitrogen treatment than the no-nitrogen treatment. Kumar et al. (Citation2017), Parihar et al.(Citation2010) and Woldesenbet and Tana (Citation2014) also reported similar results where the incorporation of inorganic to organic fertilisers significantly increased grain yield in rice, food barley and pearl millet by improving the physical and chemical properties of the soil. Chivenge et al. (Citation2009) showed that the application of mineral nitrogen fertiliser provides start-up nitrogen for use by plants and microbial nitrogen for the mineralisation of cattle manure. This reduces net immobilisation of nitrogen as microbial nitrogen uptake is faster than plant uptake of nitrogen, which induces temporary nitrogen deficiency to the plants (Chivenge et al. Citation2011). The slow N mineralisation rate from the manure (Murwira and Kirchmann Citation1993) means that some mineral N should be applied at planting to prevent N deficiency during early plant growth (Nyamangara et al. Citation2003).
Increasing nitrogen application rate to cattle manure above 50 kg N ha−1 showed no significant yield difference between sorghum varieties () because other factors may have been limiting, resulting in no increase in grain yield with an increase in nitrogen fertiliser application to cattle manure. Soil factors, such as soil pH < 5.0, may limit micronutrient availability, limiting plant growth and yield (Mapfumo and Giller Citation2001). Woldesenbet and Tana (Citation2014) found out that the application of 5 t ha−1 farmyard manure combined with 75% recommended inorganic nitrogen (23 kg N ha−1) and phosphorous (46 kg P2O5 ha−1 produced better yield and beyond this cause yield decline in food barley (Hordeum vulgare L.).
It can be concluded that tied contour and infiltration pits improved soil moisture and sorghum yields in the integrated nutrient management system compared with standard contour. The moisture provided by the rainwater-harvesting practices across distances from rainwater-harvesting practice improved rainwater productivity hence yield. Higher yield benefits in sorghum variety Macia suggest a more adapted variety than Sc Sila. Adding mineral nitrogen fertiliser to cattle manure was more beneficial at the nutrient amendment of 5 t ha−1 (cattle manure) + 50 kg N ha−1. Mineral nitrogen fertiliser above 50 kg N ha−1 added to 5 t ha−1 cattle manure had no yield benefits as an increase in N application did not result in a corresponding increase in sorghum yields. The use of contour-based rainwater-harvesting and cattle manure + N nutrient amendment can be recommended to improve soil moisture and sorghum grain yield. The benefits are more profound when appropriate adaptive sorghum varieties and cattle manure + N application rates are used in the semi-arid farming environment.
Acknowledgements
We acknowledge partial funding from International Foundation for Science (IFS) grant number C4569-2.
Disclosure statement
No potential conflict of interest was reported by the author(s).
Additional information
Funding
Notes on contributors
Friday N. M. Kubiku
Friday N. M. Kubiku is a PhD student in Agricultural Sciences at Bindura University of Science Education in the Department of Environmental Science. He holds a Masters of Science in Agroforestry, Bindura University of Science Education. His research interest is on exploring sustainable agriculture farming systems.
George Nyamadzawo
George Nyamadzawo is a Professor of Soil Science and Environmental Science, Department of Environmental Science, Bindura University of Science Education, Zimbabwe. His research interest is on climate change adaptation and promoting marginalised crops through their cultivation, use and value addition. His current research is on the cultivation, use and value addition of sesame seed, and small grains on the nutrition and health of communities. In addition he is also carrying out work on crop intensification, rainwater harvesting and in its impacts on small grains and legumes in semi–arid regions of Zimbabwe; Optimization of in filed water harvesting for climate change adaptation in the semi–arid smallholder farming sector; Organic carbon dynamics, use of biochar in reducing soil carbon loss and sustainable utilization of seasonal wetlands (dambos) in smallholder farming areas of Zimbabwe.
Justice Nyamangara
Justice Nyamangara is a Professor and Vice Chancellor of Marondera University of Agricultural Sciences and Technology. He is a Soil Fertility and Environmental Pollution Specialist with more than 20 years experience in university lecturing and administration, and CGIAR research and development in Eastern and Southern Africa. His research interest is on exploring the latest questions and answers in soil fertility, soil analysis, plant nutrition, agronomy, nutrient cycling, conservation agriculture, soil chemistry, agriculture, environmental science and irrigation.
Ronald Mandumbu
Ronald Mandumbu is an Associate Professor in the Department of Crop Science, Bindura University of Science Education. His research interest is on biology, ecology and management of weeds and current research is on Ecology of strigolactone production in Sorghum arundinaceaum and Sorghum bicolour.
References
- Angachew G. 2009. Ameliorating effects of organic and inorganic fertilizers on crop productivity and soil properties on reddish-brown soils. Proceedings of the 10th Conference of the Ethiopian Society of Soil Science, Addis Ababa, Ethiopia.
- Chianu JN, Chianu JN, Mairura F. 2012. Mineral fertilizers in the farming systems of sub-Saharan Africa. A review. Agron Sustain Dev. 32(2):545–566.
- Chivenge P, Vanlauwe B, Gentile R, Wangechi H, Mugendi D, Van Kessel C, Six J. 2009. Organic and mineral input management to enhance crop productivity in Central Kenya. Agron J. 101(5):1266–1275.
- Chivenge P, Vanlauwe B, Six J. 2011. Does the combined application of organic and mineral nutrient sources influence maize productivity? A meta-analysis. Plant Soil. 342:1–30. doi:10.1007/s11104-010-0626-5.
- Farah GA, Dagash YMI, Yagoob SO. 2014. Effect of different fertilizers (bio, organic and inorganic fertilizers) on some yield components of rice (Oryza Sativa L.). Univ J Agric Res. 2(2):67–70.
- Ghosh B, Singh RJ, Mishra P. 2015. Soil and input management options for increasing nutrient use efficiency. In: Rakshit A, Singh HB, Sen A, editors. Nutrient use efficiency: from basics to advances. New Delhi: Springer India; p. 17–27.
- Godara A, Gupta U, Singh R. 2012. Effect of integrated nutrient management on herbage, dry fodder yield and quality of oat (Avena sativa L.). Forage Res. 38(1):59–61.
- Hadebe ST, Mabhaudhi T, Modi AT. 2017. Water use of sorghum (Sorghum bicolor L. Moench) in response to varying planting dates evaluated under rainfed conditions. Water SA. 43:91–103. doi:10.4314/wsa.v43i1.12.
- Katyal J. 2000. Organic matter maintenance: mainstay of soil quality. J Indian Soc Soil Sci. 48(4):704–716.
- Kugedera A, Kokerai L, Chimbwanda F. 2018. Effects of insitu rainwater harvesting and integrated nutrient management options on Sorghum production. GSJ. 6:12.
- Kugedera AT, Mango L, Kokerai LK. 2020. Evaluating the effects of integrated nutrient management and insitu rainwater harvesting on maize production in dry regions of Zimbabwe. Int J Agric Environ Food Sci. 4(3):303–310.
- Kumar S, Saha B, Saha S, Das A, Poddar P, Prabhakar M. 2017. Integrated nutrient management for enhanced yield. Nutrients uptake and their use efficiency in rice under intensive rice-wheat cropping system. Int J Curr Microbiol Appl Sci. 6:1958–1972.
- Lal R. 2004. Soil carbon sequestration impacts on global climate change and food security. Science. 304(5677):1623–1627.
- Lal R, Follett RF, Stewart BA, Kimble JM. 2007. Soil carbon sequestration to mitigate climate change and advance food security. Soil Sci. 172(12):943–956.
- Li Z, Liu M, Wu X, Han F, Zhang T. 2010. Effects of long-term chemical fertilization and organic amendments on dynamics of soil organic C and total N in paddy soil derived from barren land in subtropical China. Soil Tillage Res. 106(2):268–274.
- Mahajan A, Bhagat R, Gupta R. 2008. Integrated nutrient management in sustainable rice-wheat cropping system for food security in India. SAARC J Agri. 6(2):29–32.
- Mahinda A, Funakawa S, Shinjo H, Kilasara M. 2018. Interactive effects of in situ rainwater harvesting techniques and fertilizer sources on mitigation of soil moisture stress for sorghum (Sorghum bicolo r (L.) Moench) in dryland areas of Tanzania. Soil Sci Plant Nutr. 64(6):710–718.
- Mandumbu R, Nyawenze C, Rugare J, Nyamadzawo G, Parwada C, Tibugari H. 2020. Tied ridges and better cotton breeds for climate change adaptation. In: African handbook of climate change adaptation. Cham: Springer International Publishing; p. 1–15.
- Mapfumo P, Giller KE. 2001. Soil fertility management strategies and practices by smallholder farmers in semi-arid areas of Zimbabwe. FAO: International Crops Research Institute for the Semi-Arid Tropics (ICRISAT).
- Masvaya EN, Nyamangara J, Descheemaeker K, Giller KE. 2017. Tillage, mulch and fertiliser impacts on soil nitrogen availability and maize production in semi-arid Zimbabwe. Soil Tillage Res. 168:125–132.
- Mhizha A, Ndiritu J. 2013. Assessing crop yield benefits from in situ rainwater harvesting through contour ridges in semi-arid Zimbabwe. Phys Chem Earth, Parts A/B/C. 66:123–130.
- Mhlanga B, Muoni T. 2014. Crop residue management in conservation agriculture systems in Zimbabwe smallholder farming sector: importance, management challenges and possible solutions. Asian J Agric Rur Dev. 4:333–340.
- Mitran T, Mani PK, Basak N, Mazumder D, Roy M. 2016. Long-term manuring and fertilization influence soil inorganic phosphorus transformation vis-a-vis rice yield in a rice–wheat cropping system. Arch Agron Soil Sci. 62(1):1–18.
- Mupangwa W, Twomlow S, Walker S. 2012. Dead level contours and infiltration pits for risk mitigation in smallholder cropping systems of southern Zimbabwe. Phys Chem Earth. 47–48: 166–172.
- Mupangwa W, Twomlow S, Walker S. 2016. Reduced tillage and nitrogen effects on soil water dynamics and maize (Zea mays L.) yield under semi-arid conditions. Int J Agric Sustain. 14:13–30. doi:10.1080/14735903.2015.1011393.
- Murwira H, Kirchmann H. 1993. Nitrogen dynamics and maize growth in a Zimbabwean sandy soil under manure fertilisation. Commun Soil Sci Plant Anal. 24(17-18):2343–2359.
- Nyagumbo I, Nyamadzawo G, Madembo C. 2019. Effects of three in-field water harvesting technologies on soil water content and maize yields in a semi-arid region of Zimbabwe. Agric Water Manag. 216:206–213. doi:10.1016/j.agwat.2019.02.023.
- Nyamadzawo G, Wuta M, Nyamangara J, Gumbo D. 2013. Opportunities for optimization of in-field water harvesting to cope with changing climate in semi-arid smallholder farming areas of Zimbabwe. SpringerPlus. 2(1):1–9.
- Nyamangara J, Mudhara M, Giller K. 2005. Effectiveness of cattle manure and nitrogen fertilizer application on the agronomic and economic performance of maize. S Afr J Plant Soil. 22(1):59–63.
- Nyamangara J, Nyengerai K, Masvaya E, Tirivavi R, Mashingaidze N, Mupangwa W, Dimes J, Hove L, Twomlow S. 2014. Effect of conservation agriculture on maize yield in the semi-arid areas of Zimbabwe. Exp Agric. 50(2):159–177.
- Nyamangara J. Piha M, Giller K. 2003. Effect of combined cattle manure and mineral nitrogen on maize N uptake and grain yield. Afr Crop Sci J. 11(4):389-300.
- Ouedraogo E, Mando A. 2010. Effect of tillage and organic matter quality on sorghum fertilizer use and water use efficiency in semi-arid West Africa. 19th World congress of soil science, soil solutions for a changing world, Brisbane.
- Parihar C, Rana K, Kantwa S. 2010. Nutrient management in pearlmillet (Pennisetum glaucum)–mustard (Brassica juncea) cropping system as affected by land configuration under limited irrigation. Indian J Agron. 55(3):191–196.
- Purushothaman R, Krishnamurthy L, Upadhyaya HD, Vadez V, Varshney RK. 2016. Genotypic variation in soil water use and root distribution and their implications for drought tolerance in chickpea. Funct Plant Biol. 44(2):235–252.
- Rufino MC, Dury J, Tittonell P, Van Wijk MT, Herrero M, Zingore S, Mapfumo P, Giller KE. 2011. Competing use of organic resources, village-level interactions between farm types and climate variability in a communal area of NE Zimbabwe. Agric Sys. 104(2):175–190.
- Singh R. 2017. Improving seed systems resiliency at local level through participatory approach for adaptation to climate change. Adv Plants Agric Res. 6(1):00–200.
- Srinivasarao C, Ramachandrappa B, Jakkula VS, Kundu S, Venkateswarlu B, Pharande A, Manideep VR, Naik RP, Venkanna K. 2014. Nutrient balance after thirteen years of organic and chemical nutrient management and yield sustainability of groundnut-fingermillet rotation in rainfed alfisols of semi-arid India. J Indian Soc Soil Sci. 62(3):235–247.
- Stevenson JR, Villoria N, Byerlee D, Kelley T, Maredia M. 2013. Green revolution research saved an estimated 18 to 27 million hectares from being brought into agricultural production. Proc Natl Acad Sci. 110(21):8363–8368.
- Woldesenbet M, Tana T. 2014. Effect of integrated nutrient management on yield and yield components of food barley (Hordeum vulgare L.) in Kaffa Zone, Southwestern Ethiopia. Sci Technol Arts Res J. 3(2):34–42.