Abstract
Improving phosphorus (P) fertilizer efficiency while minimizing environmental impacts requires better understanding of the dynamics of applied P in soils. This study assessed the fate of fertilizer P applied in Quebec Humaquepts. A pot experiment with five textural Humaquepts, each receiving 0 (P0), 10 (P10), 20 (P20) and 40 (P40) mg P kg−1 soil was conducted under barley (Hordeum vulgare L.)-soybean (Glycine max L.) rotations. A modified Hedley procedure was used for soil P fractionation. The clayey soils reached a plateau of dry matter at less P applied than the coarser-textured soils. Plant P uptake, soil labile inorganic P (resin-P + NaHCO3-Pi) and moderately labile inorganic P (NaOH-Pi) increased proportionally with P rate. The coarser-textured soils had lower contents of labile and moderately labile Pi, but a larger increase in labile Pi than the finer-textured soils after receiving P additions. The applied P was retained primarily as soil labile Pi, accounting for 43–69% of total soil recovery of applied P, compared to 20–30% recovered as moderately labile Pi, and 7–29% assumed to be sparingly soluble P (HCl-P + H2SO4-P). The labile Pi recovery of applied P was linearly depressed with clay content, compared to a quadratic relation for the moderately labile Pi recovery. The results suggest the importance of accounting for soil texture along with soil P adsorption capacity when assessing the efficiency of applied P, P accumulation in soils and subsequently P nutrient management.
Introduction
Phosphorus (P) is one of the major plant nutrients. In the last decade, however, P has been shown to be a potential pollutant of water courses (Sharpley & Tunney, Citation2000). As P nutrient management shifts from voluntary practices to mandatory compliance for farmers, policies for proper P management are being introduced.
Soil texture is one of the soil constituents affecting the availability, accumulation and transport of applied P in soils. Huffman et al. (Citation1996) reported that soil texture had a greater effect on soil labile P than the combined effects of crop residues and nutrient additions. Studies of soils with a high degree of weathering indicated that a given amount of fertilizer P resulted in a much greater increase in soil solution P in coarse-textured than fine-textured soils (Johnston et al., Citation1991; Cox, Citation1994; Zheng et al., Citation2003). As soil P adsorption and buffering capacity are often associated with clay fraction (O'Halloran et al., Citation1985), soil texture has been used as a relative index of P adsorption capacity and/or buffering capacity in improving fertilizer P recommendations, particularly on soils with similar chemical properties (Lins & Cox, Citation1989). These investigations indicated that soil texture affects the amount and the availability of soil P to the plant. However, the findings were not incorporated in current phosphorus nutrient management plans. For example, in Quebec, a single value of increase of Mehlich III extractable P (M3P) per unit applied P is used, irrespective of soil type, to manage P inputs on agricultural soils (Ministry of Environment of Quebec, Citation1999).
Due to the financial and practical limitations of using P radioisotopes, most studies on P cycling rely on the alternative method of measuring changes in soil P fractions. One technique is to sequentially separate soil P into various inorganic P (Pi) and organic P (Po) fractions based on their plant-availability and biological associations. This technique has been used to assess P status in a wide range of soils (Wager et al., Citation1986; Beck & Sanchez, Citation1994; Linquist et al., Citation1997a). It is hypothesized that this technique improves fertilizer evaluation via a fractionation of soil P into various P pools of differing plant-availability. This information is essential to the development of agro-environmental P management plans. The objective of this study was therefore to assess plant P uptake and the apparent recovery of applied P into soil P pools of differing plant-availability on Humaquepts from the province of Quebec, Canada.
Materials and methods
Pot experiment
Five agricultural soils (humic Humaquepts) with comparable pH and M3P values but a gradient in clay content from 10 to 65%, were selected from the Ap horizon (0–20 cm) of the Fleury (sandy over loamy, mixed, mesic, Typic Humaquept), Aston (sandy over clayey, mixed, mesic, Typic Humaquept), Saint-Aimé (coarse-silt, mixed, mesic Typic Humaquept), Providence (fine clayey, mixed, mesic, Aeric Humaquept), and St-Urbain (very fine clayey, mixed, mesic, Typic Humaquept) series in the St. Lawrence lowlands in the province of Quebec, Canada. The soils were sieved through a 6-mm sieve and dried for use in a pot experiment. The M3P contents categorized as medium to adequate for barley and soybean growth. The selected physical and chemical properties related to P status are shown in . Detailed description of soil properties was introduced in Zheng et al. (Citation2003).
Table I. Selected chemical and physical properties (n=4) of five Quebec Humaquepts.
Each of the tested soils received 0, 10, 20 and 40 mg P as NH4H2PO4 per kg soil (represented by P0, P10, P20 and P40) respectively, mixed and placed in 2-litre pots (2.40±0.16 kg soil per pot). Each pot received NH4NO3 in varying quantities depending on the N amount applied with the P source of NH4H2PO4 to give an amount equivalent to 60 mg N per kg soil. Each pot also received 60 mg K as KCl, 10 mg Mg as MgSO47H2O, 0.4 mg Cu as CuSO45H2O, 0.4 mg Zn as ZnSO47H2O, 0.4 mg Mn as MnSO42H2O, and 0.04 mg Mo as (NH4)6Mo7O244H2O per kg soil, respectively. The pots were distributed in an environment-controlled chamber (6 m×15 m) according to a completely randomized design in four replicates. Soil moisture was adjusted daily in the morning to 85% of field capacity (−33 kPa) by weighing the pots and adding de-ionized water as required. The air temperature was 22±3°C, and fluorescent lighting was maintained daily for 16 h at an intensity of 150 W m−2. Twelve spring barley (Hordeum vulgare L. cv. Chapais) seeds were planted in each pot and the seedlings were thinned to six plants per pot one week after emergence. The plants were harvested by cutting near the soil surface after two months of growth. The soils were then frozen (1 week), and thawed (1 week) to mimic field conditions. Thereafter, soils were separated from pots and visible roots removed. Fertilizer P and other nutrients were added and mixed thoroughly at the same rates as those for spring barley except the basal N nutrient, which was reduced to 40 mg N kg−1 to account for the N fixed by soybean. Soils were then put back into the pots. Eight soybean (Glycine max L. cv. Maple Glen) seeds were planted in each pot, thinned to three plants per pot one week after emergence, and cut after two months growth. Daily management was similar to that of the barley phase. The barley and soybean were grown sequentially in the two rotation cycles (one growth phase of barley plus one of soybean was defined as one rotation cycle). Barley-soybean is a common crop rotation in the region. The soils were sampled after the two cycles of barley-soybean rotation.
Plant and soil analysis
Shoots and visible roots were dried at 65°C for 48 h, weighed and milled. The tissues were digested with concentrated H2SO4 and H2O2, and P concentrations in digestates were determined by Richards (Citation1993). The plant P uptake was estimated as the product of dry matter yield and tissue P concentration. Soil samples were air-dried, crushed to pass through a 100-mesh sieve, and sequentially extracted according to Hedley et al. (Citation1982) procedure as modified by O'Halloran (Citation1993). This procedure uses a series of increasingly stronger extracting agents (anion exchange resin in Cl− form, 0.5 M NaHCO3, 0.1 M NaOH, 1 M HCl and 0.9 M H2SO4) to sequentially desorb labile P fractions (resin-P and NaHCO3-Pi/Po) at first, followed by moderately labile P (NaOH-Pi/Po) and sparingly soluble fractions (HCl-P and H2SO4-P). The analytical data showed that P additions had no significant influence on labile Po (NaHCO3-Po and NaOH-Po) and sparingly soluble P pools in the short-term; hence, this paper focuses mainly on the contribution of applied P on labile Pi and moderately labile Pi, but it did take account of other functional groups when interpretation was necessary. Total soil P was directly measured by digesting soil in an autoclave (103.4 kPa, 121°C for 1.5 h) with 0.9 M H2SO4 acidified K2S2O8 oxidation (Environmental Protection Agency, Citation1971). The P concentrations in extracts were determined colorimetrically by the molybdate-blue method (Murphy & Riley, Citation1962).
Statistical analysis
To simplify analysis, dry matter yield and plant P uptake in this study were considered in cumulative amounts produced over the study time of two cycles of barley-soybean rotation, expressed as production per kg of tested soils. Total soil recovery of applied P was defined as the increase in total P in fertilized treatment relative to control (zero-P). The recovery of soil P pools of differing plant-availability was calculated as the amount of either labile Pi or moderately labile Pi in the fertilized treatment minus that in the control, and was expressed relative to the percentage of total soil recovery of applied P. The analysis of variance was carried out with a completely randomized model to determine treatment effects. Regression analysis was preformed to identify the relationships between the planned variables. Statistical analyses were performed using SAS procedures (SAS Inst., Citation1990).
Results and discussion
Dry matter yield and plant P uptake
There was good establishment of plant growth in all P treatments. Phosphorus deficiency was observed in the control in the second cycle of the barley-soybean rotation, but no luxuriant plant growth was recorded in the treatment with the highest P rate. The cumulative dry matter yield was significantly higher in clay and heavy clay than in three coarser-textured soils fertilized with P10 and P20, but not in the P40 treatment (). The dry matter yield with P addition apparently fitted the Mitscherlich growth curve, but the clayey soils reached a plateau with less P applied than the coarser-textured ones (). This is attributable to their higher initial labile Pi (resin-P + NaHCO3-Pi) compared with the coarser-textured soils (). The observation was also supported by the cumulative plant P uptake with a greater increase at P20 over P10 and smaller increase at P40 over P20 in the fine-textured than the coarser-textured soils (). The statistical analysis also indicated that P uptake by plants was complicated by a soil texture×P regime interaction (data not shown). In fact, P uptake by plants is not only controlled by the quantity and intensity factors, such as the amount of P added, soil P content and the concentration of soil solution P, but also by diffusion factors governing P movement from soil solid phase to plant roots, such as water content, aeration and pore continuity conditions in the rhizosphere (Barber, Citation1995). In spite of this interaction, plant P uptake was more closely related to applied P (r=0.96, P<0.001) than to clay content (r=0.57, P<0.05), which is in agreement with the results reported by Ivarsson (Citation1990).
Soil labile and moderately labile Pi
After two cycles of the barley-soybean rotation, the resultant labile Pi pool was much higher in two clayey soils than in three coarser-textured soils, regardless of P rate (), being inconsistent with the higher potential P adsorption capacities represented by higher soil Alox and Feox contents (Tran et al., Citation1988) in clayey soils compared with the coarser-textured soils (). This is because resin-P and NaHCO3-Pi are comprised of Pi adsorbed on surfaces of more crystalline P compounds, sesquioxide or carbonates (Mattingly, Citation1975), with less association to Al and Fe phosphates. The positive correlation between labile Pi and soil clay content was previously reported on other Quebec Appalachian soils (mostly Humic Humaquepts) (O'Halloran et al., Citation1985; Beauchemin & Simard, Citation2000).
Figure 3. Labile Pi contents in soils and their increments over control after two crop rotation cycles of barley-soybean, as influenced by P rate and soil texture on five Quebec Humaquepts (bar = standard deviations).
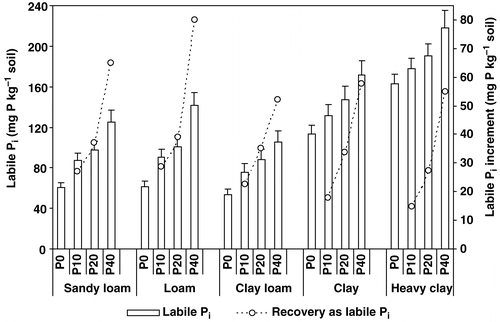
The labile Pi increment, calculated as the difference between fertilized and unfertilized treatments, increased proportionally to P rate, with larger increase in the coarser-textured than the finer-textured soils, particularly at high P application levels (). Similar results were reported by Johnston et al. (Citation1991) for 54 South African soils from five soil orders. Cox (Citation1994) also observed that the increase in M3P per unit of P applied after one year was 0.7 units for soils with only 10% clay, and decreased to <0.2 units for soils with >50% clay. The higher initial labile Pi in clayey soils compared to the coarser-textured soils overshadowed to some extent their increments of labile Pi. Also, the diffusion-related mechanism was speculated to explain the smaller increase in labile P per unit of applied P in clayey soils. Fertilizer P is initially adsorbed on the periphery of soil aggregates and subsequently diffuses very slowly into aggregates as a result of very small intra-aggregate diffusion coefficients. As clay content increases, more water-stable aggregates, coupled with the increased diffusion coefficients due to decreased tortuosity and increased buffering capacity induced by more Al and Fe oxides, result in more diffusion of adsorbed P into aggregates which depress the readily available P as slow diffusion of P out of aggregates limits the availability of interior aggregated P (Linquist et al., Citation1997b). Most of the currently practical P nutrient management plans were established based on one recommended soil P test in the region (Gartley et al., Citation2002). For example, the Ministry of the Environment of Quebec (Citation1999) introduced a calculation to predict the M3P amount in the provincial agro-environmental fertilization plans. A single value of 3.5 kg fertilizer P per unit of M3P increase in excess of plant uptake was retained. The findings in the present study that applied P raised labile Pi more in the coarser-textured than finer-textured soils, suggest that a correction based on soil texture should be introduced to better reflect P accumulation on Humaquepts of the St. Lawrence lowlands. As the increase in clay content is often accompanied by increases in soil P adsorption and buffering capacities (Kamprath & Waston, Citation1980), the observations of present study, along with previous reports of Johnston (Citation1991) and Cox (Citation1994), suggest that clay content with soil P adsorption capacity and/or P buffering capacity would be useful criteria to group soils for P nutrient management.
NaOH extractable Pi (NaOH-Pi) is acknowledged as the P chemisorbed on amorphous Al and Fe minerals (Tiessen et al., Citation1984). The pool was considered as moderately labile P since it can act as a source of plant available P in absence of P inputs, and as a sink for P added in case of application in excess of crop removal (Beck & Sanchez, Citation1994). The moderately labile Pi contents in two clayey soils were much higher than those in three coarser-textured soils (). This is related to their larger Alox and, particularly, Feox contents (). The moderately labile Pi increment over control at different P inputs was however complicated by soil texture. The increments were larger in loamy sand and heavy clay than the other three soils, especially at P40 (). This indicated that soil factors other than clay content contributed to accumulation of moderately labile Pi, but the exact cause remains uncertain in this study. In addition, the concomitant increase in labile Pi and moderately labile Pi along with P addition suggests that fertilizer P was added in excess of plant removal in this study.
Labile and moderately labile Pi recovery of applied P
The labile Pi and moderately labile Pi recovery of applied P, as respectively expressed relative to total soil recovery of applied P, were constantly reduced with increasing P addition, but the standard deviations signify that the extent of reduction varied from soil to soil (). However, the averaged labile Pi recovery of applied P across P rates linearly declined with clay content, in contrast to a quadratic relation for the recovery of applied P as moderately labile Pi (). The negative relationship between labile Pi recovery and clay content is in agreement with the results noted earlier of the depressive effects of clay content in raising labile P in soils. Fertilizer P was primarily retained as labile Pi in the tested soils, accounting for 43–69% of total soil recovery of applied P, compared with 20–30% recovered as moderately labile Pi (). This trend tended to be more evident in the coarser-textured than the finer-textured soils. This is consistent with the results obtained in Canadian Chernozemic soils which showed more soil recovered fertilizer P was as labile P (48%), followed by the moderately (43%) and sparingly soluble (9%) P pools (Wager et al., Citation1986). However, this was in contradiction to the results obtained in Ultisols with high P fixation capacity in tropical climates, on which considerably more P was recovered as moderately labile Pi rather than as labile Pi (Beck & Sanchez, Citation1994; Linquist et al., 1996). Summing the labile and moderately labile Pi recoveries, the data also indicate that a considerable proportion of applied P (7–29%) was presumably recovered as sparingly soluble P (HCl-P + H2SO4-P) in the tested soils. This is more pronounced in two clayey (average 27%) than in three coarser-textured soils (average 14%). This could be due to soil P transformations and redistribution among P pools of differing plant-availability. The formation of sparingly soluble P at expense of more labile P can be promoted by high C content and enhanced microbial activities in clayey soils (Zheng et al., Citation2002). However, the contribution of P additions in this study did not cause a significant increase in sparingly soluble P, apparently because it only represented about 1.4–3.7% of total size of this pool.
Conclusions
Despite uncertainties remaining on the distinction between applied P and soil indigenous P, soil fractionation data are likely to be helpful to fertilizer evaluation. Soil texture affected plant P uptake and the recovery of applied P as soil labile Pi and moderately labile Pi in this study. The potential implications of the observations for improving short- and long-term P management strategies are significant. The applied P is predominately retained as labile Pi in the coarser-textured soils, which indicates that the coarse-textured soils are particularly susceptible to P leaching loss. As clay content increases, more adsorbed P fertilizers on the outside of soil aggregates diffuse into aggregates and fix as Al and Fe phosphates and become less available for immediate plant uptake, but long-term residual P benefits will be high in clayey soils. Grouping soils by texture (clay content), along with soil P adsorption capacity and/or P buffering capacity, seems to be a valuable option for sound P nutrition management. This is likely to be of significance for further reviews of the agro-environmental nutrient management policy in the region.
Acknowledgments
We gratefully acknowledge Mr. Mario Deschenes for soil selection and Dr. Michel C. Nolin for soil classification. The authors also acknowledge financial support from the Natural Sciences and Engineering Research Council of Canada, and facility support from Soils and Crops Research and Development Centre, Agriculture and Agri-Food Canada. Thanks are extended to Mr. J. Brian Sanderson for critical review of this manuscript.
References
- Barber , S. A. 1995 . Soil nutrient bioavailability: a mechanistic approach , 2nd edn , 133 – 156 . New York, NY : John Wiley and Sons .
- Beauchemin , S. and Simard , R. R. 2000 . Phosphorus status of intensively cropped, neutral to calcareous soils of the St. Lawrence lowlands . Soil Science Society of America Journal , 64 : 659 – 670 .
- Beck , M. A. and Sanchez , P. A. 1994 . Soil phosphorus fraction dynamics during 18 years of cultivation on a Typic Paleudult . Soil Science Society of America Journal , 58 : 1424 – 1431 .
- Cox , F. R. 1994 . Predicting increase in extractable phosphorus from fertilizing soils of varying clay content . Soil Science Society of America Journal , 58 : 1249 – 1253 .
- Gartley , K. L. , Sims , J. T. , Olsen , C. T. and Chu , P. 2002 . Comparison of soil test extractions used in mid-Atlantic United States . Communications in Soil Science and Plant Analysis , 33 : 873 – 895 .
- Environmental Protection Agency . 1971 . Methods of chemical analysis of water and waste , Cincinnati, OH, , USA : US EPA .
- Hedley , M. J. , Stewart , J. W. B. and Chauhan , B. S. 1982 . Changes in inorganic and organic phosphorus induced by cultivation practices and laboratory incubations . Soil Science Society of America Journal , 46 : 970 – 976 .
- Huffman , S. A. , Cole , C. V. and Scott , N. A. 1996 . Soil texture and residue addition effects on soil phosphorus transformation . Soil Science Society of America Journal , 60 : 1095 – 1101 .
- Ivarsson , K. 1990 . The long-term soil fertility experiments in southern Sweden. IV. Changes in inorganic and organic soil phosphorus fractions after a pot trial . Acta Agriculturae Scandinavica , 40 : 205 – 215 .
- Johnston , M. A. , Miles , N. and Thibaud , G. R. 1991 . Quantities of phosphorus fertilizer required to raise the soil test value . South African Journal of Plants and Soils , 8 : 17 – 21 .
- Kamprath , E. J. and Waston , M. E. 1980 . “ Conventional soil and tissue tests for assessing the phosphorus status of soils ” . In The role of phosphorus in agriculture , Edited by: Khasawneh , F. H. 433 – 469 . Madison, , WI : ASA, CSSA, and SSSA .
- Linquist , B. A. , Singleton , P. W. and Cassman , K. G. 1997a . Inorganic and organic phosphorus dynamics during a build-up and decline of available phosphorus in an Ultisol . Soil Science , 162 : 254 – 364 .
- Linquist , B. A. , Singleton , P. W. , Yost , R. S. and Cassman , K. G. 1997b . Aggregate size effects on the adsorption and release of phosphorus in an Ultisol . Soil Science Society of America Journal , 61 : 160 – 166 .
- Lins , I. D. G. and Cox , F. R. 1989 . Effect of extractant and selected soil properties on predicting the optimum phosphorus fertilizer rate for growing soybeans under field conditions . Communications in Soil Science and Plant Analysis , 20 : 319 – 333 .
- Mattingly , G. E. G. 1975 . Labile phosphate in soils . Soil Science , 119 : 369 – 375 .
- Ministry of the Environment of Quebec . 1999 . Agro-environmental fertilization guide . Quebec , QC , 28 pp .
- Murphy , J. and Riley , J. P. 1962 . A modified single solution method for the determination of phosphorus in natural waters . Anal. Chem. Acta , 27 : 31 – 36 .
- O'Halloran , I. P. , Kachanoski , R. G. and Stewart , J. W. B. 1985 . Spatial variability of soil phosphorus as influenced by soil texture and management . Canadian Journal of Soil Science , 65 : 475 – 487 .
- O'Halloran , I. P. 1993 . Effect of tillage and fertilizer on inorganic and organic phosphorus . Canadian Journal of Soil Science , 73 : 359 – 369 .
- Richards , J. E. 1993 . “ Chemical characterization of plant tissue ” . In Soil sampling and methods of analysis , Edited by: Carter , M.R. 115 – 139 . Boca Raton, FL : Canadian Society of Soil Science, Lewis Publishers .
- Ross , G. J. and Wang , C. 1993 . “ Extractable Al, Fe, Mn, and Si ” . In Soil sampling and methods of analysis , Edited by: Carter , M. R. 239 – 246 . Boca Raton, FL : Canadian Society of Soil Science, Lewis Publishers .
- SAS Institute . 1990 . SAS/STAT user's guide : vol. 1 and vol. 2. Version 6, 4th edn ., SAS Inst ., Cary, NC .
- Sharpley , A. N. and Tunney , H. 2000 . Phosphorus research strategies to meet agricultural and environmental challenges of 21st century . Journal of Environmental Quality , 29 : 176 – 181 .
- Sheldrick , B. H. and Wang , C. 1993 . “ Particle size distribution ” . In Soil sampling and methods of analysis , Edited by: Carter , M. R. 499 – 511 . Boca Raton, FL : Canadian Society of Soil Science, Lewis Publishers .
- Tiessen , H. , Stewart , J. W. B. and Cole , C. V. 1984 . Pathways of phosphorus transformation in soils of differing pedogenesis . Soil Science Society of America Journal , 48 : 853 – 858 .
- Tran , T. S. , Fardeau , J. C. and Giroux , M. 1988 . Effects of soil properties on plant-available P determined by the isotopic dilution 32P method . Soil Science Society of America Journal , 52 : 1382 – 1390 .
- Wager , B. I. , Stewart , J. W. B. and Moir , J. O. 1986 . Changes with time in the form and availability of fertilizer phosphorus on Chernozemic soils . Canadian Journal of Soil Science , 66 : 105 – 119 .
- van der Zee , S. E. A. T. M. , Gjaltema , A. , van Riemsdijk , W. H. and de Haan , F. A. M. 1988 . Spatial variability of phosphate adsorption parameters . Journal of Environmental Quality , 17 : 682 – 688 .
- Zheng , Z. , Simard , R. R. , Lafond , J. and Parent , L. E. 2002 . Pathways of soil phosphorus transformation after eight years of cultivation under contrasting cropping practices . Soil Science Society of America Journal , 66 : 999 – 1007 .
- Zheng , Z. , Simard , R. R. and Parent , L. E. 2003 . Anion Exchange and Mehlich III phosphorus in Humaquept varing in clay content . Soil Science Society of America Journal , 67 : 1287 – 1295 .