Abstract
This study aimed to assess the extractability of cadmium (Cd) and Cd uptake by rice after applying soil amendments of lime (Ca(OH)2) and sepiolite. A rice (Oryza sativa) cultivation experiment was conducted in a Cd-contaminated paddy field. After applying lime, sepiolite, and a mixture of the two as soil amendments, soil pH showed a significant increase (p<0.05), and Cd in soil extracted with NaNO3, CaCl2, and DTPA, was reduced by 61–100%, 52–98%, and 12–15%, respectively (p<0.05). The amount of exchangeable Cd showed a significant decrease in all treatments (p<0.05), and we found increases in the proportion of forms of carbonate-bound, Fe/Mn oxide-bound, and residual Cd in the soils, as measured by a sequential extraction method. The grain or rice straw biomass was neither significantly influenced by any amendment in the experiment, while the uptake of Cd by rice significantly decreased in all treatments (p<0.05). The results reveal no advantage in applying a mixture of sepiolite and lime compared with applying sepiolite alone in immobilizing Cd in the soil. The use of sepiolite or sepiolite mixed with lime was more effective than the use of lime alone in the immobilization of Cd in the soil. We therefore recommend sepiolite as a soil amendment to remediate Cd-contaminated paddy soil.
Keywords:
Introduction
Cadmium (Cd) is a toxic heavy metal that poses a threat to the environment and to human health. As a result of rapid industrialization in many countries, Cd-polluted soil has become a major global concern, particularly in China. In situ immobilization of Cd in contaminated soils is a cost-effective and environmentally friendly remediation technique (Zhao & Saigusa, Citation2007). This approach is based on the addition of amendments to soil to increase the metal component of the amendment solid phase, either by precipitation or increased metal sorption (Brown et al., Citation2005; Gray et al., Citation2006), thereby reducing the pool of heavy metal in the soil available for root uptake (Cheng & Hseu, Citation2002; Basta & McGowen, Citation2004).
Lime is widely used as a soil amendment, as it acts to reduce the soluble and exchangeable fraction of Cd and reduces the uptake of Cd by crops (Basta & Sloan, Citation1999; Hong et al., Citation2007). Many clay minerals are effective amendments in immobilizing Cd in soils, including palygorskite (Álvarez-Ayuso & García-Sánchez, Citation2003a), bentonite (Cheng & Hseu, Citation2002; van Herwijnen et al., Citation2007), montmorillonite (Badora et al., Citation1998), and Penghu soil (Cheng & Hseu, Citation2002). Previous studies have reported that sepiolite has high sorption capacities of Cd and the ability to reduce the mobility of Cd in soil polluted by mining activities (Álvarez-Ayuso & García-Sánchez, Citation2003b; Shirvani et al., Citation2006a ,Citationb), thereby indicating its potential use in remediating Cd-polluted soil.
Few field trials have evaluated the effectiveness of sepiolite in immobilizing heavy metals in soils, yet in situ field experiments are essential before this approach could be applied to real situations. Therefore, the objectives of the present study are to (i) evaluate the change in the bioavailability of Cd following the addition of lime and sepiolite to a Cd-polluted paddy soil; (ii) explain the influence of soil amendments on the speciation of Cd in the soil, as evaluated by sequential fractionation; and (iii) evaluate the uptake of Cd in rice.
Materials and methods
Field experiment
This study was conducted in a paddy soil in Zhuzhou City, Hunan Province, China (113°01′E, 27°49′N) since July 2007. The soil at the study site is classified as acidic Ultisol derived from Quaternary red clay, and became contaminated by Cd via the drainage of wastewater from a nearby electroplating factory. The factory was shut down by the authorities in April of 2006 for its high risk of contamination.
Representative samples of surface soil (0–20 cm) were collected to determine the soil properties prior to the study. The soil characteristics were as follows: pH, 5.34; cation exchange capacity (CEC), 10.78 cmol kg−1; organic carbon, 25.12 g kg−1; total N, 2.45 g kg−1; Olsen-P, 8.15 mg kg−1; available K, 123.92 mg kg−1; CaCO3, 0.67 g kg−1; dithionite–citrate–bicarbonate extracted Fe (DCB-Fe), 16.51 g kg−1; DTPA-extracted Cd, 1.33 mg kg−1; and total Cd, 2.98 mg kg−1.
The experiment was designed to study the effects of lime (consisting mainly of Ca(OH)2) and sepiolite (particle diameter ≤ 74 µm; composition on an oxide basis as follows: SiO2, 52%; MgO, 15%; Al2O3, 7%; Fe2O3, 3%; H2O−, 13.3%; H2O+, 8.1%; collected from Xiangtan City, Hunan province, China) amendments on the immobilization of Cd in soil. We employed a 2×2 factorial design with two levels of lime and sepiolite, making a total of four treatments, denoted CK (unamended), L (150 g lime per m2), S (2250 g sepiolite per m2), and LS (150 g lime mixed with 2250 g sepiolite per m2). The field was tilled to a depth of 15–20 cm before the plots were separated. Each plot was 8 m long and 2.5 m wide, and adjacent plots were separated by a ridge (0.3 m height and 0.3 m wide) covered with plastic film. The plots were arranged in three randomized blocks, and amendments were hand-scattered over the surface. The plots were tilled again once the amendments had been added, and 7 days later we transplanted 30-day-old seedlings of rice (Oryza sativa cv. Jinyou 207) at a spacing of 0.20×0.25 m and three seedlings per hill.
Sampling
Rice was harvested at the maturity stage (border rows removed and the remainder harvested), at which time we recorded the fresh weights of grain and straw in each plot and measured water content by oven-drying. To determine cadmium concentrations, plant samples were separated into grain (separated into brown rice and hull after drying) and straw, dried at 40°C to constant weight, and ground to pass a sieve of 0.3 mm. Each soil sample was a composite of about 8 cores collected randomly from the experimental plot at a depth of 0–20 cm. Air-dried samples were sieved through a 2 mm screen and analyzed to determine extracted Cd.
Analysis
We analyzed the composition of lime and sepiolite using an X-ray diffractometer (D8 ADVANCE, Bruker, Germany) equipped with a Cu-filter and operated at 40.0 kV, 40.0 mA, and scanned (2κ) between 5.000° and 65.000° at a rate of 0.02° S−1.
Plant materials (1.000 g dried weight) were digested (open system) using a mixture of 15 ml HNO3 and 3 ml HClO4. Soil samples from different treatments were collected and evaluated for bioavailability and fractional redistribution of Cd by single and sequential extraction. The single-extraction method was as follows: (i) NaNO3 extraction method (Gupta & Aten, Citation1993): 16.00 g of dried and 2-mm-sieved soil in 40 ml of 0.1 M NaNO3, shaken for 2 h; (ii) CaCl2 extraction method (Novozamsky et al., Citation1993): 4.00 g of soil in 40 ml of 0.01 M CaCl2, shaken for 3 h; and (iii) DTPA extraction method (Lindsay and Norvell, Citation1978): 20.00 g of soil in 40 ml of 0.05 M DTPA + 0.01 M CaCl2+0.01 M TEA, at pH 7.3, shaken for 2 h.
Sequential extraction was performed for air-dried soil samples from each plot according to the method described by Tessier et al. (Citation1979). The procedure was conducted in five steps, based on the following forms of extracted Cd:
-
Fraction 1 (water-soluble plus exchangeable Cd: EX): 1.000 g soil (dried weight) was extracted with 8 ml 1 M MgCl2 solution, shaken for 1 h at 25±1°C, and the extract was separated from the solid residue by centrifugation at 3000 g for 20 min.
-
Fraction 2 (carbonate-bound Cd: CB): to the residue of Fraction 1, we added 8 ml of 1 M CH3COONa solution (pH = 5, adjusted with acetic acid). The mixture was shaken for 5 h at 25±1 °C and centrifuged to obtain the extract as per step 1.
-
Fraction 3 (Fe/Mn oxide-bound Cd: OX): to the residue of Fraction 2, we added 20 ml of 0.04 M NH2OH · HCl in 25% acetic acid. The mixture was refluxed at 96±3°C with occasional agitation for 6 h, and centrifuged to obtain the extract as per step 1.
-
Fraction 4 (organic-bound Cd: OC): to the residue of Fraction 3, we added 3 ml of 0.02 M HNO3 and 5 ml of 30% H2O2; the pH was adjusted to 2.0 with HNO3. The mixture was refluxed at 85±2°C for 2 h. A second 5 ml aliquot of 30% H2O2 (pH 2) was then added and the sample was again refluxed for 3 h. After cooling, we added 5 ml of 3.2 M ammonium acetate in 20% (v/v) HNO3. The sample was diluted to 20 ml, agitated continuously for 30 min, and centrifuged to obtain the extract as per step 1.
-
Fraction 5 (residual: RES): the residue of Fraction 4 was digested (open system) using a mixture of 15 ml aqua regia and 3 ml HClO4.
Cadmium concentrations in solution were determined by ICP-MS (GBC, Australia) and AAS (GBC, Australia). In all cases, the calibration method for ICP-MS employed an external calibration procedure with indium (In) as internal standard.
CEC was determined by the ammonium acetate method (Chapman, Citation1965). Organic C and total N were measured by dry combustion in a CN auto-analyzer (Vario MAX C/N, Germany), and Olsen-P and available K were extracted with 0.5 M NaHCO3 and 1 M NH4OAC, respectively. Soil samples (1.000 g dried weight) were digested (open system) using a mixture of 5 ml HNO3, 15 ml HCl, and 3 ml HClO4, and concentrations of Cd in digested solution were determined by AAS (GBC, Australia) to calculate the total Cd content. Soil pH was determined in water using a pH meter at a soil:solution ratio of 1:2.5 (w/v). CaCO3 content was determined using a calcimeter by measuring the volume of water displaced by CO2 evolving from soil by reacting with 6 M HCl. Free iron compounds (DCB-Fe) were extracted with DCB solution (Mehra & Jackson, Citation1960).
Analysis of variance for comparisons among means was conducted using SPSS statistical software (SPSS 11.5 for Windows).
Results
Mineralogy of lime and sepiolite
shows the X-ray diffraction patterns of lime and sepiolite. Remarkable diffraction peaks derived from Ca(OH)2 and CaCO3 are observed in the lime sample; the peak for quartz is not observed. CaCO3 is considered to have been derived from the hydration of Ca(OH)2 and the subsequent absorption of CO2. In the sepiolite sample, we observed peaks assigned to sepiolite (Mg4SiO15(OH)2H2O and 2MgOSiO2H2O) and quartz as an accessory mineral.
Temporal changes in soil pH, CEC, CaCO3, and DCB-Fe, and single extraction of Cd
Soil pH was significantly influenced by the application of lime and sepiolite (p <0.05), but we observed no significant interaction between the lime and sepiolite treatments ( and ). Following application of the various amendments, we observed a significant increase in soil pH from 5.4 in the control soil (CK) to 5.9 in treatment L, 6.3 in S, and to 6.8 in LS. Soil CEC increased significantly with the application of sepiolite (p <0.05). Compared with CK, soil CEC in the L, S, and LS treatments increased by 8–15%. The CaCO3 content in the soil showed a significant increase in the L and S treatments (p <0.001), and we found a significant interaction between the two treatments (p <0.001). Compared with the CK treatment, CaCO3 content in the L, S, and LS treatments increased by 120%, 223%, and 536%, respectively. No significant change in DCB-Fe was observed for any of the treatments.
Figure 2. Relationships between soil pH and Cd concentrations in soil extracted by 0.1M NaNO3 (A), 0.01 M CaCl2 (B) and DTPA (C). (◊) CK: Control (unamended); (▴) L: Lime applied at 150 g m−2; (▪) S: Sepiolite applied at 2250 g m−2; (•) LS: Lime applied (at 150 g m−2) in a mixture with sepiolite (at 2250 g m−2). ***Significant at p <0.001; *Significant at p <0.05.
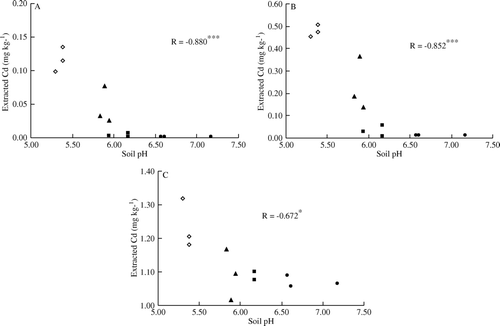
Table I. Mean values of pH, CEC, CaCO3, DCB-Fe and single-extractable Cd in plough-layer soils treated by different amendments (n=3 for each treatment).
Extractable Cd in soil was removed by three kinds of extractant (0.1 M NaNO3, 0.01 M CaCl2, and DTPA) for samples from each treatment. The relationship between soil pH and the concentration of extractable Cd is shown in and . Extractable Cd concentrations with 0.1 M NaNO3 and 0.01 M CaCl2 were significantly influenced by the application of lime and sepiolite, and there exists a strong interaction between lime and sepiolite treatments. DTPA-extracted Cd concentrations were significantly influenced by the application of sepiolite, but not significantly affected by the application of lime or interaction between lime and sepiolite treatments. It was equally effective in reducing the amounts of Cd extracted by 0.1 M NaNO3 and 0.01 M CaCl2 in soils subjected to the S and LS treatments; in this regard, these two treatments were more effective than the L treatment. Compared with CK, significantly less DTPA-extracted Cd was obtained in the S and LS treatments. Furthermore, the concentration of Cd extracted with 0.1 M NaNO3, 0.01 M CaCl2, or DTPA showed a significant negative correlation with soil pH.
Temporal changes in the chemical fractionation of Cd in treated soil
shows the distribution of Cd in the control (CK) and amended soils (L, S, and LS), as assessed by sequential extraction. Without soil additives (CK), the amount of Cd in the various fractions followed the order of EX > OX > OC > RES ≈ CB. Cd occurred mainly as exchangeable and Fe/Mn oxide-bound forms in the soils, indicating a high availability for plant uptake in this contaminated soil. For amended soil, all treatments acted to strongly modify the partitioning of Cd from available to less-available phases. Compared with CK, the exchangeable Cd decreased by 20%, 26%, and 37% for the L, S, and LS treatments, respectively, while Cd bound to carbonate increased by 48%, 50%, and 121%, Cd bound to Fe/Mn oxides increased by 5%, 12%, and 12%, and residual forms in the soils increased by 25%, 46%, and 25%, respectively. The amount of Cd bound to organic matter was not significantly influenced by addition of the amendments.
Figure 3. Distribution of cadmium (%) in soil affected by different amendments (□) EX: Water-soluble plus exchangeable Cd; (
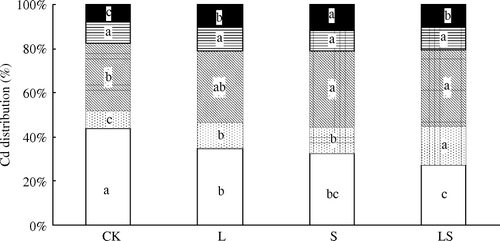
Biomass production and plant uptake of Cd
We observed no significant impact of lime, sepiolite, or interaction between the two treatments on grain yield or rice straw production (); however, rice uptake of Cd showed a significant decrease in the L, S, and LS treatments (p<0.05) (). Compared with the CK treatment, Cd contents in brown rice, hull, and straw were significantly reduced by the L, S and LS treatments (p <0.05). Compared with the CK treatment, Cd contents in brown rice of the L, S, and LS treatments showed decreases of 20%, 37.5%, and 34%; decreases in the hull were 37.5%, 47%, and 50%, and those in straw were 30%, 41%, and 38%, respectively. The addition of S or LS was equally effective in reducing the Cd content of brown rice, hull, and straw. The Cd content of brown rice in the LS and S treatments was significantly lower than that in the L treatment (p > 0.05), and the Cd content of hull in the LS treatment was lower than that in the L treatment.
Discussion
As one of the most important factors affecting the availability of heavy metals, soil pH plays an important role in determining the solid–solution equilibrium of metals in soil (Gerriste & Van Driel, Citation1984; Hooda & Alloway, Citation1998). For the Cd-contaminated paddy soil analyzed in the present study, the application of amendments of lime, sepiolite, or a mixture of the two caused a significant increase in soil pH ( and ). An increase in soil pH has also been reported in previous studies in which lime or other clay minerals (e.g., bentonite) were used as soil amendments (Chen et al., Citation2000; Gray et al., Citation2006; Hong et al., Citation2007). These changes reflect the high pH of the applied amendments relative to that of the studied soils.
NaNO3 and CaCl2 mainly dissolve the cation-exchangeable fraction (Pueyo et al., Citation2004), and DTPA acts to release a considerable amount of the fraction bound to Fe/Mn oxide and carbonate (Hammer & Keller, Citation2002). These extractants are commonly adopted in predicting the bioavailability of heavy metals in soils (Haq et al., Citation1980; Gupta & Aten, Citation1993; Houba et al., Citation2000; McBride et al., Citation2004). The application of lime, sepiolite, or a mixture of the two could act to reduce the amount of extractable Cd in soils. In the present case, lime mixed with sepiolite was the most effective treatment. Several other studies have also reported a similar decrease in extractable Cd concentrations in contaminated soils after the application of amendments such as lime and sepiolite. For example, Álvarez-Ayuso and García-Sánchez (Citation2003b) reported that the application of 4% sepiolite reduced the amount of extractable Cd by 84%, while Hong et al. (Citation2007) reported that the application of 8 Mg ha−1 lime decreased the amount of extractable Cd from 1.51 to 0.70 mg kg−1. As stated above, when considering the significant negative correlation between soil pH and amount of extractable Cd in the soil, the decrease in extractable Cd in the amended plots is primarily attributed to the increase in soil pH resulting from application of the amendments.
In our study, the concentration of exchangeable Cd decreased in proportion to the increasing fractions of Cd bound to carbonate, bound to Fe/Mn oxides, and residual Cd following the application of amendments. This finding is in agreement with the results of single extraction. A comparable redistribution of Cd in soil has been reported by previous studies in the presence of lime (Brallier et al., Citation1996; Fernandes et al., Citation1999; Hong et al., Citation2007) and other clay minerals (e.g., bentonite; Chen et al., 2000). The findings of these previous studies suggest that the addition of lime or clay minerals to treated soils shifts the solid phases of metals away from mobile forms to immobile or less bio-available forms.
The results of the present study clearly reveal that pH increase, caused by the addition of lime, sepiolite, or a mixture of the two, and played a major role in the redistribution of Cd among different fractions in soil. The increase in soil pH resulted in a significant increase in the contents of CaCO3 and soil CEC, which possibly led to Cd precipitation or adsorption due to an increase in the surface negative charge (Naidu et al., Citation1994; Gray et al., Citation1998). Furthermore, the sepiolite represented a new adsorptive surface that possibly immobilizes Cd in the soil via specific adsorption or chemisorption. For example, several studies have demonstrated the capacity of sepiolite to absorb Cd, and contaminated soil amended with sepiolite showed a significant reduction in the extractable fraction of Cd and leaching of Cd (Álvarez-Ayuso & García-Sánchez, Citation2003b; Shirvani et al., Citation2006a ,Citationb). Once Cd substitutes for Mg on the edges of the octahedral sheet in clay minerals and is sorbed to the mineral surface, complexation of Cd may occur on surface functional groups. In addition, Zhao and Saigusa (Citation2007) noted that the effects of soil additives on Cd forms in soil were dependent on the properties of the additives. The high DCB-Fe content of the soil analyzed in the present study could possibly explain the significant increase in the Fe/Mn oxide-bound fraction in soil following addition of the amendments ().
The recorded decreases in Cd concentrations within brown rice, hull, and straw were related to changes in the Cd fractions in the soil. For example, Cd-extracted with NaNO3, CaCl2 and DTPA decreased by 61–100%, 52–98% and 12–15%, respectively, in soils treated with the various amendments (); correspondingly, the concentration of Cd decreased by 20–37.5% in brown rice, 37.5–50% in hull, and 30–41% in straw, respectively, compared with the control (). This finding suggests that the bioavailability of Cd has an important effect on the plant uptake of Cd. Although we observed a significant decrease in extractable Cd with the increase in soil pH that accompanied the application of lime, sepiolite, and a mixture of the two, the yields of grain and straw biomass showed no change. Previous studies have also reported the insignificant effect of Cd pollution on rice yield (Yu et al., Citation2006). Because rice could take up large amounts of Cd without showing any phytotoxic symptoms, Cd in the food chain should be considered a potentially serious hazard to human health.
In conclusion, we therefore recommend the application of sepiolite, rather than sepiolite mixed with lime, to remediate Cd-contaminated paddy soil. And, further studies are required to understand the long-term effects of their application on the phytoavailability of Cd in soil.
Acknowledgements
This study was supported by the National Key Technologies R &D Program of China (2006BAD05B01, 2008 BADA7B02).
References
- Álvarez-Ayuso , E. and García-Sánchez , A. 2003a . Palygorskite as a feasible amendment to stabilize heavy metal polluted soils . Environmental Pollution , 125 : 337 – 344 .
- Álvarez-Ayuso , E. and García-Sánchez , A. 2003b . Sepiolite as a feasible soil additive for the immobilization of cadmium and zinc . Science of the Total Environment , 305 : 1 – 12 .
- Badora , A. , Furrer , G. , Grünwald , A. and Schulin , R. 1998 . Immobilization of zinc and cadmium in polluted soils by polynuclear Al13 and Al-montmorillonite . Journal of Soil Contamination , 7 ( 5 ) : 573 – 588 .
- Basta , N.T. and McGowen , S.L. 2004 . Evaluation of chemical immobilization treatments for reducing heavy metal transport in a smelter-contaminated soil . Environmental Pollution , 127 : 73 – 82 .
- Basta , N.T. and Sloan , J.J. 1999 . Bioavailability of heavy metals in strongly acidic soils treated with exceptional quality biosolids . Journal of Environmental Quality , 28 : 633 – 638 .
- Brallier , S. , Harrison , R.B. , Henry , C.L. and Xue , D.S. 1996 . Liming effects on availability of Cd, Cu, Ni and Zn in a soil amended with sewage sludge 16 years previously . Water, Air, and Soil Pollution , 86 : 195 – 206 .
- Brown , S. , Christensen , B. , Lombi , E. , McLaughlin , M. , McGrath , S. , Colpaert , J. and Vangronsveld , J. 2005 . An inter-laboratory study to test the ability of amendments to reduce the availability of Cd, Pb, and Zn in situ . Environmental Pollution , 138 : 34 – 45 .
- Chapman , H.D. 1965 . “ Cation exchange capacity ” . In Methods of soil analysis , Edited by: Black , C.A. 891 – 901 . American Society of Agronomy, American Society for Testing and Materials, USA .
- Chen , Z.S. , Lee , G.J. and Liu , J.C. 2000 . The effects of chemical remediation treatments on the extractability and speciation of cadmium and lead in contaminated soils . Chemosphere , 41 : 235 – 242 .
- Cheng , S.F. and Hseu , Z.Y. 2002 . In-situ immobilization of cadmium and lead by different amendments in two contaminated soils . Water, Air, and Soil Pollution , 140 : 73 – 84 .
- Fernandes , M.L. , Abreu , M.M. , Calouro , F. and &Vaz , M.C. 1999 . Effect of liming and cadmium application in an acid soil on cadmium availability to Sudan grass . Communications in Soil Science and Plant Analysis , 30 : 1051 – 1062 .
- Gerriste , R.G. and Van Driel , W. 1984 . The relationship between adsorption of trace metals, organic matter, and pH in temperate soils . Journal of Environmental Quality , 13 : 197 – 204 .
- Gray , C.W. , Dunham , S.J. , Dennis , P.G. , Zhao , F.J. and McGrath , S.P. 2006 . Field evaluation of in situ remediation of a heavy metal contaminated soil using lime and red-mud . Environmental Pollution , 142 : 530 – 539 .
- Gray , C.W. , McLaren , R.G. , Roberts , A.H.C. and Condron , L.M. 1998 . Sorption and desorption of cadmium from some New Zealand soils: effect of pH and contact time . Australian Journal of Soil Research , 36 : 199 – 216 .
- Gupta , S.K. and Aten , C. 1993 . Comparison and evaluation of extraction media and their suitability in a simple model to predict the biological relevance of heavy metal concentrations in contaminated soils . International Journal of Environmental Analytical Chemistry , 51 : 25 – 46 .
- Hammer , D. and Keller , C. 2002 . Changes in the rhizosphere of metal accumulating plants evidenced by chemical extractants . Journal of Environmental Quality , 31 : 1561 – 1569 .
- Haq , A.U. , Bates , T.E. and Soon , Y.K. 1980 . Comparison of extractants for plant-available zinc, cadmium, nickel, and copper in contaminated soils . Soil Science Society of America Journal , 44 : 772 – 777 .
- Hong , C.O. , Lee , D.K. , Chung , D.Y. and Kim , P.J. 2007 . Liming effects on cadmium stabilization in upland soil affected by gold mining activity . Archives of Environmental Contamination Toxicology , 52 : 496 – 502 .
- Hooda , P.S. and Alloway , B.J. 1998 . Cadmium and lead sorption behaviour of selected English and Indian soils . Geoderma , 84 : 121 – 134 .
- Houba , V.J.G. , Temminghoff , E.J.M. , Gaikhorst , G.A. and van Vark , W. 2000 . Soil analysis procedures using 0.01M calcium chloride as extraction reagent . Communications in Soil Science and Plant Analysis , 31 : 1299 – 1396 .
- Lindsay , W.L. and Norvell , W.A. 1978 . Development of a DTPA soil test for zinc, iron, manganese, and copper . Soil Science Society of America Journal , 42 : 421 – 428 .
- McBride , M.B. , Richards , B.K. and Steenhuis , T. 2004 . Bioavailability and crop uptake of trace elements in soil columns amended with sewage sludge products . Plant and Soil , 262 : 71 – 84 .
- Mehra , O.P. and Jackson , M.L. 1960 . Iron oxide removal from soils and clays by dithionite-citrate systems buffered with sodium bicarbonate . Clays and Clay Minerals , 7 : 317 – 327 .
- Naidu , R. , Bolan , N.S. , Kookana , R.S. and &Tiller , K.G. 1994 . Ionic-strength and pH effects on the sorption of cadmium and the surface charge of soils . European Journal of Soil Science , 45 : 419 – 429 .
- Novozamsky , I. , Lexmond , T.H.M. and Houba , V.J.G. 1993 . A single extraction procedure of soil for evaluation of uptake of some heavy metals by plants . International Journal Environmental Analytical Chemistry , 51 : 47 – 58 .
- Pueyo , M. , López-Sánchez , J.F. and Rauret , G. 2004 . Assessment of CaCl2, NaNO3 and NH4NO3 extraction procedures for the study of Cd, Cu, Pb and Zn extractability in contaminated soils . Analytica Chimica Acta , 504 : 217 – 226 .
- Shirvani , M. , Kalbasi , M. , Shariatmadari , H. , Nourbakhsh , F. and Najafi , B. 2006a . Sorption-desorption of cadmium in aqueous palygorskite, sepiolite, and calcite suspensions: Isotherm hysteresis . Chemosphere , 65 : 2178 – 2184 .
- Shirvani , M. , Shariatmadari , H. , Kalbasi , M. , Nourbakhsh , F. and Najafi , B. 2006b . Sorption of cadmium on palygorskite, sepiolite and calcite: Equilibria and organic ligand affected kinetics . Colloids and Surfaces A: Physicochemical and Engineering Aspects , 287 : 182 – 190 .
- Tessier , A. , Campbell , P.G.C. and Bisson , M. 1979 . Sequential extraction procedure for the speciation of particulate trace metals . Analytical Chemistry , 51 ( 7 ) : 844 – 851 .
- van Herwijnen , R. , Hutchings , T.R. , Al-Tabbaa , A. , Moffat , A.J. , Johns , M.L. and Ouki , S.K. 2007 . Remediation of metal contaminated soil with mineral-amended composts . Environmental Pollution , 150 ( 3 ) : 347 – 354 .
- Yu , H. , Wang , J.L. , Fang , W. , Yuan , J.G. and Yang , Z.Y. 2006 . Cadmium accumulation in different rice cultivars and screening for pollution-safe cultivars of rice . Science of the Total Environment , 370 ( 2–3 ) : 302 – 309 .
- Zhao , X.L. and Saigusa , M. 2007 . Fractionation and solubility of cadmium in paddy soils amended with porous hydrated calcium silicate . Journal of Environmental Sciences , 19 : 343 – 347 .