Abstract
Many scientists consider Si to be a ‘quasi-essential’ element for plants. Farmers in a number of countries now apply Si-containing fertilizers to the soil in order to improve crop yield. The adsorption of Si onto soil particles is an important process affecting the availability of Si to plants, yet little is known about the fate of Si after it is added to the soil. The objective of this paper was to study the adsorption of Si in three soils at different initial Si concentrations, temperatures (293 and 303 K), and reaction times. Si-adsorption behavior varied significantly between soil types. Si adsorption and adsorption rate were highest in the Yellow Drab soil and lowest in the Lou soil. In all the three soils, the slopes of the adsorption isotherms remained constant as initial Si concentrations increased from 0 to 50 mg Si/L, but declined rapidly as initial Si concentrations increased from 50 to 200 mg Si/L. This suggests that these soils contained multiple Si-adsorption sites. Langmuir, Freundlich, and Temkin equations all described the adsorption of Si as a function of Si concentration reasonably well, however their goodness of fit varied according to soil type. The soils also showed significant differences in buffering capacities, supply parameters, and percent saturation. The reaction kinetics for Si adsorption in the Yellow Drab and Purple Paddy soils could be divided into two stages, an initial fast reaction (0~2 h) followed by a slow reaction (2~12 h). Si adsorption in the Lou soil was slow but steady throughout the reaction period. The correlation coefficients (r 2 ) for all the equations used in this study were significant at p<0.05 significant levels. Among the kinetic equations used in this study, the parabolic diffusion, bi-constants function, Langmuir, and Elovich equations were the best for describing the relationship between reaction time and the amount of Si adsorbed onto soil particles. An analysis of activation energy (E a ) suggested that the rate-limiting step for Si adsorption in the Yellow Drab soil and the Purple Paddy soil was a diffusion-controlled process, while Si adsorption in the Lou soil seemed to be a chemically controlled process. This study shows that there are significant differences in the adsorption of Si between soil types and highlights the importance of future studies to investigate the mechanisms for Si adsorption in soil.
Introduction
Silicon is the second most abundant element on the surface of the earth and accounts for up to 31% of the earth's crust by weight. Silicon concentrations in the soil solution range between 3 and 17 mg/kg (Savant et al., Citation1997; Sommer et al., Citation2006). Silicon has not been considered to be an essential element for plants and has not been included in any standard formulation of nutrient solutions or fertilizers for a long time. Many plants contain a relatively high concentration of Si (Epstein, Citation1994; Marschner, Citation1995). However, within a given plant specie, tissue levels of silicon vary in relation to Si availability in the soil (Datnoff et al., Citation1991). In the last two or three decades, Si has been shown to be a beneficial element for many, and, under certain conditions, perhaps most terrestrial plants (Epstein, Citation1994; Marschner, Citation1995). The beneficial effects of adequate Si include decreased susceptibility to fungal pathogens (and insects), amelioration of abiotic stresses, increased growth in some plants, and enhanced nutrition uptake (Miyake & Takahashi, Citation1983; Ma & Takahashi, Citation1990a ,Citationb; Epstein, Citation1994; Marschner, Citation1995; Wiese et al., Citation2005), and enhanced tolerance for heavy metals in contaminated soil (Liang et al., Citation2005). In recent years, Si-containing fertilizers have been widely used in China, Japan, and Korea for rice and sugarcane production and in Europe for the production of some greenhouse crops. As a result, Si is now considered a ‘quasi-essential’ element for plant growth and development (Epstein, Citation1999).
The adsorption of Si at the soil particle–water interface has an important effect on the transport, bioavailability, and fate of Si added to soil. The adsorption of Si by mineral oxides has been investigated by a number of researchers. Jones & Handreck (Citation1963) found that reagent-grade iron and aluminium oxide materials adsorbed moderate amounts of Si. McKeague & Cline (Citation1963) reported that aluminium and iron oxides adsorbed high amounts of Si, haematite, and goethite showed moderate adsorption, and silicate minerals, alkaline earth carbonates, and reagent-grade aluminium hydroxide exhibited little or no adsorption. An investigation conducted by Herbillon & Vinh An (Citation1969) indicated that Si was adsorbed by two distinct processes, chemisorption and polymerization.
However, previous studies focused mainly on the adsorption of Si by mineral oxides and offer little useful information about the fate of Si added to the soil as fertilizer. The objective of this research was to investigate the adsorption behavior of Si in three different soils. The thermodynamic and dynamic characteristics of Si adsorption were determined by fitting the data using the thermodynamic and kinetic equations.
Materials and methods
The soils used in this study were collected from the surface of three sites in western China. According to the Chinese classification system, the soils are a Lou soil (Yangling, Shaanxi Province), a Yellow Drab soil (Hanzhong City, Shaanxi Province), and a Purple Paddy soil (Deyang City, Sichuan Province). The three soils are Anthrosols, Argosols, and Cambisols according to International Soil Taxonomy (FAO/ISRIC/ISSS, Citation1998), respectively. Soil samples were air-dried, crushed to pass through a 1-mm sieve, and stored in plastic containers until analysis. The physical and chemical characteristics of the three soils are listed in .
Table I. Physical and chemical properties of the soils used in this study.
Adsorption of Si as a function of initial Si concentration
Adsorption isotherms for Si were used to illustrate the relation between the amount of Si adsorbed and the Si concentrations in solution of each soil type. Twenty milliliters of Si solution (0, 5, 10, 20, 40, 60, 80, 100, 150, and 200 mg Si/L) were added to 1.00 g soil samples in 80-mL polyethylene centrifuge tubes. Aqueous solutions were prepared in 0.01 mol/L NaNO3. The soil solutions were equilibrated for 24 h at 293±1 K on a reciprocating air-bath shaker. After equilibration, the mixtures were centrifuged at 4000 g for 10 min and filtered. The Si concentration in the supernatant of each sample was determined according to methods described by Nayar et al. (Citation1977). The amount of Si adsorbed by the soil was calculated as the difference between the Si concentration in the original and equilibrium solutions.
The Langmuir [Equation (Equation1)], Freundlich [Equation (Equation2)], and Temkin [Equation (Equation3)] equations were used to interpret Si adsorption for each soil type.
Q is the amount of adsorbed Si (mg/kg);
Q m is the adsorption maximum (mg/kg);
K is the bonding-energy constant;
k is a constant related to the adsorption capacity of the soil;
1/n is a constant related to the adsorption intensity of the soil;
A and B are equation constants.
For the Langmuir adsorption equation, correlation coefficients between C and Q were calculated and the lines fitted by regression analysis. Line slopes and intercepts were used to calculate the adsorption maxima (Q m ) and bonding energy constants (K).
The differential buffering capacity (B) of each soil was calculated by Equation (Equation5):
b is the slope of the adsorption isotherm;
C is the equilibrium concentration of Si in the soil solution (mg/L).
The supply parameter (SP) for Si in each soil was calculated using the relationship suggested by Khasawneh & Copeland (Citation1973) for the supply of P in soils, Equation (Equation6):
Percent saturation (PS) represents the amount of adsorbed Si at any initial Si concentration compared with the maximum amount of Si that could be absorbed by the soil. It was calculated by Equation (Equation7):
The thermodynamic parameters for Si adsorption were calculated by Equation (Equation8):
Adsorption of Si as a function of time and temperature
Adsorption kinetic experiments were conducted using conventional batch methods. We added 20 mL of Si solution (50 mg Si/L) to 1.00-g soil samples in 80-mL polyethylene centrifuge tubes. The mixtures were equilibrated for 0.5, 1, 2, 4, 8, 12, 16, and 24 h at 293±1 K and 303±1 K on a reciprocating air-bath shaker, centrifuged at 4000 g for 10 min, and filtered. The Si concentration in the supernatant of each sample was determined and the amount of adsorbed Si was calculated using the methods described in the previous section.
The kinetic data for the Si adsorption reactions were calculated using the Equations given in . The effects of temperature on Si adsorption by each soil were examined using the Arrhenius equation to determine the rate constants. The Arrhenius equation is given by Equation (Equation9):
Table II. Kinetic equations used in this study.
Statistical analysis
Regression and other statistical analyses were conducted using SAS statistical software (SAS Inst., Citation1989).
Results and discussion
Adsorption of Si as a function of initial Si concentrations
The adsorption of Si increased greatly as the Si concentration in the equilibrium solution increased (). The slopes of the isotherms were very steep when Si concentrations were low (0~50 mg Si/L) but decreased markedly when Si concentrations were higher than 50 mg Si/L. This indicates that the adsorption of Si in soils may include multiple adsorption sites. The Yellow Drab soil adsorbed more Si than the did Lou and Purple Paddy soils at the same initial Si and equilibrium Si concentrations. Si adsorption was lowest in the Lou soil. At the highest initial Si concentration (200 mg Si/L), the Yellow Drab soil adsorbed 52% of the Si applied to the soil; the Lou soil adsorbed 17% and the Purple Paddy soil adsorbed 34% of the added Si. These results suggest that the Yellow Drab soil may not have reached its maximum adsorption potential at the levels of Si added to the soil in this study. The results in further indicate that the highest adsorption percentage for each soil occurred when initial Si concentrations were low, which agrees with the changes in the slope of the isotherms that we observed. Differences in Si adsorption among the three soils may be attributed to the unique properties of each soil. Soil pH and CEC are the main factors controlling Si-adsorption patterns. With higher pH and lower CEC, organic matter, and carbonate contents, the Lou soil adsorbed less Si than did the Yellow Drab and Purple Paddy soils, which have rather lower pH and higher CEC, organic matter, and carbonate contents. Meanwhile, soil minerals also exert significant control over Si adsorption. Quartz and feldspar in Lou soil contribute to less Si adsorption, while hydromica and montmorillonite contribute to more adsorption of Si in Yellow Drab and Purple Paddy soils.
Figure 1. Relationship between the equilibrium Si concentration and the amount of Si adsorbed at 293 K.
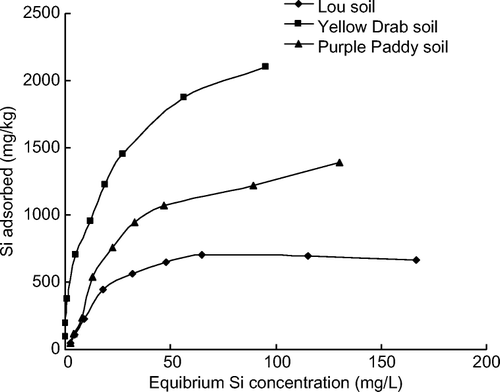
The statistical significance of the correlation coefficient between the equilibrium Si concentration and the amount of Si adsorbed by each soil was used to test the fit of the data to the adsorption equations. The Langmuir, Freundlich, and Temkin adsorption equations were all able to describe the adsorption of Si reasonably well, but the accuracy of the adsorption equations varied with the soil type (). The correlation coefficients (r 2 ) of the adsorption equations suggested that the Langmuir equation was better at describing Si adsorption in the Lou and Yellow Drab soils compared with the Purple Paddy soil. The Freundlich equation described Si adsorption better for the Yellow Drab and Purple Paddy soils compared with the Lou soil. The Temkin equation was most accurate in describing Si adsorption in the Lou and Purple Paddy soils. The ▵G-values of adsorption, a relative measure of the change in free energy during the process of Si adsorption, were all lower than zero, indicating that the Si-adsorption reactions were spontaneous in the three soil types. The values of ▵G in the three soils ranged from −15.17 to −19.83 kJ/mol and declined in the following order: Purple Paddy soil > Lou soil> Yellow Drab soil ().
Table III. Adsorption parameters and r 2 of the Langmuir, Freundlich, and Temkin equations and ▵G-values for the adsorption reaction in the three soils.
The differential buffering capacity of soils is an indication of the resistance to change in the ion concentration of the soil solution when ions are added to or removed from it. When the initial Si concentration was 5~200 mg/L, the differential buffering capacity of the Yellow Drab soil ranged from 37.8 to 1.2 and was higher than that for the other two soil types (). The buffering capacities of the Lou and Purple Paddy soils were similar at initial Si concentrations of 5~20 mg/L, but the buffering capacity in the Lou soil was significantly lower than in the Purple Paddy soil when initial Si concentrations were between 40 and 200 mg/L. In all soils, there was a negative correlation between the initial Si concentration and the differential buffering capacity. The correlation coefficients were −0.825 (p =0.0062) for the Lou soil, -0.915 (p =0.0005) for the Yellow Drab soil, and −0.869 (p =0.0024) for the Purple Paddy soil.
Table IV. Influence of initial Si concentration on the differential buffering capacity (B), supply parameter (SP) and percent saturation (PS).
The concept of supply parameter (SP) was introduced by Khasawneh & Copeland (Citation1973) to integrate quantity, intensity, and buffering capacity for phosphorus in soil. This index can be used to estimate the chemical fate of other ions in soil from different aspects as well. In this study, we used the concept to describe Si adsorption. Supply parameters were lower in the Yellow Drab soil compared with the Lou and Purple Paddy soils (). Supply parameters for the Lou and Purple Paddy soils followed a pattern similar to our observations for the differential buffering capacity. Specifically, the supply parameters for the Lou and Purple Paddy soils were similar at low initial Si levels (5~80 mg Si/L) but at higher initial Si levels (100~200 mg Si/L) the supply parameters were lower in the Lou soil compared with the Purple Paddy soil. Generally, the supply parameters increased as the initial Si concentrations or equilibrium Si concentrations in the soil solution increased. In contrast to the differential buffering capacity, there was a significant positive correlation between the supply parameter and the initial Si concentration (data not shown). The correlation coefficients were 0.9982 (p <0.0001) for the Lou soil, 0.9884 (p <0.0001) for the Yellow Drab soil, and 0.9996 (p <0.0001) for the Purple Paddy soil.
The percent saturation (PS) of Si increased as initial Si concentrations increased (). The percent saturation in the Lou soil was significantly greater than in the Yellow Drab and Purple Paddy soils when initial Si concentrations were 0~100 mg/L. In contrast, when initial Si concentrations were 150~200 mg/L the Yellow Drab soil had the highest percent saturation. Of the three soil types, the Purple Paddy soil always had the lowest percent saturation of Si. The correlation coefficients between percent saturation and initial Si concentrations were significant (p<0.01) for all three soils. These results indicate that the application of Si increased the quantity of Si adsorbed by the soil particles. Because quantity and intensity are in equilibrium, the addition of Si would also result in an increase in the amount of Si available to plants.
The results also show that the adsorption maximum, bonding-energy constant, and buffering capacity were higher for the Yellow Drab soil compared with the Lou and Purple Paddy soils ( and ). This indicates that the Yellow Drab soil has a greater affinity for added Si compared with the other two soil types.
Adsorption of Si as a function of reaction time and temperature
The effect of reaction time on the amount of Si adsorbed can be reflected by the relationships between adsorption time and Si concentration in the soil solution at different reaction temperature ( and ). Silica adsorption reached equilibrium within 24 h in all three soils (); however, the adsorption patterns differed significantly from each other. The Yellow Drab soil always adsorbed more Si compared with the two other soil types, indicating that Yellow Drab soil was apt to adsorb Si. The Yellow Drab soil adsorbed 679 mg Si/kg at 293 K and 716 mg Si/kg at 303 K. In contrast, Si adsorption was lowest in the Lou soil, which adsorbed 275 mg Si/kg at both temperatures.
Figure 2. Relationship between adsorption reaction time and Si concentration in the soil solution at 293 K.
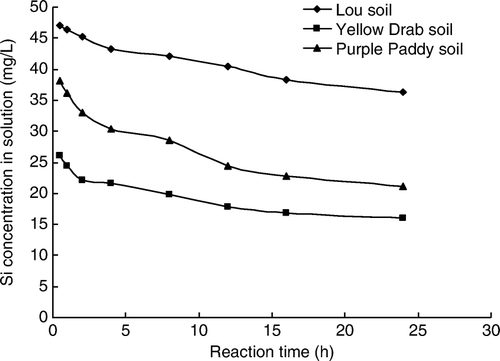
Figure 3. Relationship between adsorption reaction time and Si concentration in the soil solution at 303 K.
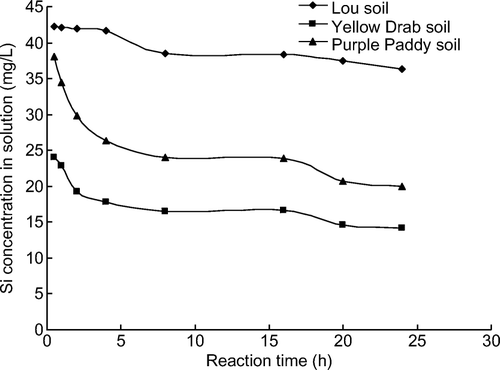
When initial Si concentrations were 50 mg Si/L, calculations showed that 27.5% of the added Si had been adsorbed by the Lou soil at equilibrium, compared with 67.9% for the Yellow Drab and 57.7% for Purple Paddy soils at 293 K (). At 303 K, the Lou, Yellow Drab, and Purple Paddy soils adsorbed 27.5, 71.6, and 60.1% of the added Si respectively (). These results show that among the three soils, the Lou soil adsorbed the smallest percentage of the added Si.
Silicon adsorption was slowest in the Lou soil. Si adsorption in the Yellow Drab and Purple Paddy soils followed a typical kinetic pattern at both temperatures, with a rapid initial decrease in the Si concentration followed by a period of slow decrease and/or quasi-equilibrium. This indicates that the adsorption of Si in the Yellow Drab and Purple Paddy soils consisted of a multiple-rate process (). The reaction kinetics of Si adsorption could be divided into an initial fast reaction (0~2 h) followed by a slow reaction (2~12 h). This multiple-rate adsorption may be related to the heterogeneity of the adsorption sites, which are believed to result from differences in the accessibility of surface pores and adsorption sites in the soil, each of which have different adsorption affinities and bonding strengths.
The kinetic and empirical equations outlined in the Methods section were applied to the Si-adsorption data for each soil type at 293 K and 303 K. The goodness of fit of the equations to the data was evaluated by using the correlation coefficients (r 2 ), probabilities (p), and standard deviations (SE) from linear regression analyses. The results from the fitting of these equations are shown in . The correlation coefficients (r 2 ) of all the equations were significant at p<0.05. This is consistent with the existing literature dealing with heterogeneous systems, where it has often been shown that a number of kinetic and empirical equations seem to describe adsorption-rate data satisfactorily when r 2 , p, and SE are used as indices to judge goodness of fit. During the testing of the eight equations, the SE-values were normalized to the same unit (amount adsorbed per unit mass) since it is related to the closeness of the equation predictions with the observed, untransformed data (Saha et al., Citation2004). The r 2 - and p-values also gave a fair measure of the relationship between the dependent variable (reaction time) and independent variable (amount of Si adsorbed) of each equation (). In this case either one or both of the variables is generated through the transformation of the original data. These results show that the parameters of the equations are useful tools for comparing the rates of the adsorption processes on the soil particles.
Table V. The correlation coefficients (r 2 ), probability (p), and standard deviation (SE) of different equations for the three soils at 293 and 303 K.
It has been shown that the fitness of equations that used to calculate anion adsorption in soil varied with soil properties (Nychas & Kosmas, Citation1984; Alves & Lavorenti, Citation2004; Borggaard et al., Citation2004). In our study, the fitness of three ordered equations (zero-order, first-order, and second-order) varied with the temperature and soil type (). On the whole, the second-order equation gave relatively higher r 2 -, and lower p- and SE-values compared with the zero- and first-order equations at both temperatures. However, among the eight equations used in the study, the parabolic diffusion, bi-constants function, Langmuir, and Elovich equations were best at describing the relationship between reaction time and the amount of Si adsorbed onto soil particles. These four equations all gave rather high r 2 - and low p- and SE-values.
We determined the range and mean values of r 2 and SE for each equation as it was applied to different soil types and at different temperatures (). The results also indicated that, among the eight equations, the above four equations (parabolic diffusion, bi-constants function, Langmuir, and Elovich equations) had the higher mean r 2 -values coupled with lower mean SE-values. Furthermore, the ranges of these parameters showed that, for both the upper and lower limits, the Langmuir equation had the highest r 2 - and lowest SE-values.
The increase in temperature led to a great increase in the rate constants for Si adsorption in the Lou soil. This may be attributed to the availability of more energy for bond-breaking and -formation at the higher reaction temperature. The rate constant of the Lou soil increased by about four times when the temperature increased from 293 to 303 K. In contrast, the increase in temperature caused a slight reduction in the Si-adsorption rate constants of the Yellow Drab and Purple Paddy soils. Accordingly, the increase in reaction temperature also resulted in differences in the E a - and A-values among different soils ().
Table VI. The rate constant (K a ), activation energy (E a ), and pre-exponential factor (A) for the adsorption of Si by the three soils.
Activation energy is a useful value, since it indicates the size of the energy barrier that must be crossed for the reaction to proceed. The three soils had substantially different E a -values for the adsorption reaction, which contributed to the difference of Si adsorption in the soils shown in . The Lou soil had the highest E a , indicating that the Lou soil had a higher energy barrier to overcome in order to adsorb Si from the soil solution. On the other hand, Yellow Drab and Purple Paddy soils had negative E a -values, suggesting that Si was easily adsorbed in these soils because there was no need to cross an energy barrier. Thus the low amount of Si adsorbed from the soil solution can be attributed to the high E a in the Lou soil, while the relatively high amount of Si adsorbed in the Yellow Drab and Purple Paddy soils can be attributed to their low E a -values. These results agreed with the results presented in .
The activation energy of a diffusion-controlled process in solution is about 25 kJ/mol (Sparks, Citation1999). However, in heterogeneous systems (soil–water interfaces), diffusion occurs not only in the bulk solution but also in micropores and macropores, in the films around solid particles, on the solid surface, and inside solid particles. Therefore, the activation energy for diffusion processes is higher in heterogeneous systems than in solutions. Diffusion often has an activation energy lower than 42 kJ/mol, hence low activation energy (< 42 kJ/mol) is indicative of a diffusion-controlled process while high activation energy (> 42 kJ/mol) indicates a chemically controlled process (Sparks, Citation1995 ,Citation1999). The E a -values from this study are listed in . The rate-limiting step for Si adsorption in the Yellow Drab soil and Purple Paddy soil was a diffusion-controlled process (E a <42 kJ/mol in both soils); meanwhile the reaction in the Lou soil was a chemically controlled process (E a >42 kJ/mol). The E a -values of the three soils suggested that the adsorption of Si on the soil particles in the Yellow Drab and Purple Paddy soils required less energy than in the Lou soil. This is also supported by the fact that the Yellow Drab and Purple Paddy soils adsorbed more Si than did the Lou soil.
The pre-exponential factor, an index of the frequency of collision of Si with adsorption sites on soil particles, was much lower for the Purple Paddy soil and much higher for the Lou soil compared with the Yellow Drab soil (). This may be attributed to the distribution of adsorption sites on the soil particles, a factor that largely depends on the physical structure and mineral composition of the soil.
Acknowledgements
This study was supported by the The Knowledge Innovation Program of CAS (KZCX2-YW-441) and West Light Program of CAS. The authors thank the reviewers of this paper for their useful suggestions.
References
- Alves , M.E. and Lavorenti , A. 2004 . Sulfate adsorption and its relationships with properties of representative soils of the São Paulo State, Brazil . Geoderma , 118 : 89 – 99 .
- Borggaard , O.K. , Szilas , C. , Gimsing , A.L. and Rasmussen , L.H. 2004 . Estimation of soil phosphate adsorption capacity by means of a pedotransfer function . Geoderma , 118 : 55 – 61 .
- Datnoff , L.E. , Raid , R.N. , Snyder , G.H. and Jones , D.B. 1991 . Effect of calcium silicate on blast and brown spot intensities and yields of rice . Plant Disease , 75 : 729 – 732 .
- Epstein , E. 1994 . The anomaly of silicon in plant biology . Proceedings of the National Academy of Sciences of the United States of America , 91 : 11 – 17 .
- Epstein , E. 1999 . Silicon . Annual Review of Plant Physiology and Plant Molecular Biology , 50 : 641 – 664 .
- FAO/ISRIC/ISSS 1998 . World Reference Base for Soil Resources . World Soil Resources Reports, Rome .
- Herbillion , A.J. and Vinh An , J.T. 1969 . Heterogeneity in silicon-iron mixed hydroxides . Journal of Soil Science , 20 : 223 – 235 .
- Jones , L.H.P. and Handreck , K.A. 1963 . Effects of iron and aluminum oxides on silica in soil solution in soil . Nature , 198 : 852 – 853 .
- Khasawneh , F.E. and Copeland , J.P. 1973 . Cotton root growth and uptake of nutrients: Relation of phosphorus uptake to quantity, intensity and buffering capacity . Soil Science Society of America Journal , 37 : 250 – 254 .
- Liang , Y.C. , Wong , J.W.C. and Wei , L. 2005 . Silicon-mediated enhancement of cadmium tolerance in maize (Zea mays L.) grown in cadmium contaminated soil . Chemosphere , 58 : 475 – 483 .
- Ma , J.F. and Takahashi , E. 1990a . Effect of silicon on the growth and phosphorus uptake of rice . Plant and Soil , 126 : 115 – 119 .
- Ma , J.F. and Takahashi , E. 1990b . The effect of silicic acid on rice in a P-deficient soil . Plant and Soil , 126 : 121 – 125 .
- Marschner , H. 1995 . Mineral Nutrition of Higher Plants , London : Academic Press .
- McKeague , J.A. and Cline , M.G. 1963 . Silica in soil solution. II. The adsorption of monosilicic acid by soil and by other substances . Canadian Journal of Soil Science , 43 : 83 – 96 .
- Miyake , Y. and Takahashi , E. 1983 . Effect of silicon on the growth of solution-cultured cucumber plant . Journal of Plant Nutrition and Soil Science , 29 : 71 – 83 .
- Nayar , P.K. , Misra , A.K. and Patnack , S. 1977 . Evaluation of silica-supplying power of soils for growing rice . Plant and Soil , 47 : 487 – 494 .
- Nychas , A.E. and Kosmas , C.S. 1984 . Phosphate adsorption by dark alkaline vertisols in Greece . Geoderma , 32 : 319 – 327 .
- Saha , U.K. , Liu , C. , Kazok , L.M. and Huang , P.M. 2004 . Kinetics of selenite adsorption on Hydroxyaluminum- and Hydroxyaluminosilicate- Montmorillonite complexes . Soil Science Society of America Journal , 68 : 1197 – 1209 .
- SAS Institute 1989 SAS/STAT User's Guide , Version 6. 4th edn SAS Inst Cary, NC
- Savant , N.K. , Snyder , G.H. and Datnoff , L.E. 1997 . “ Silicon Management and Sustainable Rice Production ” . In Advances in Agronomy , Edited by: Sparks , D.L. 151 – 199 . New York : Academic Press .
- Sommer , M. , Kaczorek , D. , Kuzyakov , Y. and Breuers , J. 2006 . Silicon pools and fluxes in soils and landscapes-a review . Journal of Plant Nutrition and Soil Science , 169 : 310 – 329 .
- Sparks , D.L. 1995 . Environmental Soil Chemistry , San Diego, CA : Academic Press .
- Sparks , D.L. 1999 . “ Kinetics of Reactions in Pure and Mixed Systems ” . In Soil Physical Chemistry , 2nd edn , Edited by: Sparks , D.L. 83 – 178 . Boca Raton, FL : CRC Press .
- Wiese , J. , Wiese , H. , Schwartz , J. and Schubert , S. 2005 . Osmotic stress and silicon act additively in enhancing pathogen resistance in barley against barley powdery mildew . Journal of Plant Nutrition and Soil Science , 168 : 269 – 274 .