Abstract
Two new (1 and 2) and seven known phenolic compounds were isolated from a methanol extract of the rhizomes and roots of Gentiana scabra. Their structures were identified by spectroscopic analysis and comparing with reported values. The inhibitory effects of each compound on soluble epoxide hydrolase (sEH) were evaluated. Among them, compounds 2, 6, and 9 potently inhibited sEH activity.
The genus Gentiana, family Gentianaceae, comprises over 400 species that are widely distributed in alpine habitats within the temperate regions of Asia, Europe, and America.Citation1) Gentiana scabra Bunge, a perennial herbaceous plant, grows mainly in temperate climates, such as China, Japan, South Korea, Russia, and North America. Rhizomes and roots of G. scabra are used in traditional Chinese medicine for the treatment of inflammation, anorexia, indigestion, and gastric infections.Citation2) Additionally, G. scabra has been shown to protect the liver, inhibit liver dysfunction, and promote gastric acid secretion, rendering it a popular ingredient in Chinese herbal medicine and health products.Citation3) Various secondary metabolites of G. scabra, such as secoiridoids, triterpenoids, flavonoids, xanthones, and alkaloids, have also been reported.Citation4–9) Current research on G. scabra focuses on its primary functional components, including gentiopicroside, swertiamarin, and sweroside.Citation7) These compounds exhibit smooth muscle relaxation, as well as antibacterial and free radical scavenging activities.Citation10,11) Although much research has focused on the biological activity of G. scabra, its soluble epoxide hydrolase (sEH) inhibitory activity has not yet been fully elucidated. In this study, the inhibitory effects of each isolated compound on sEH were evaluated.
sEH is a component of the arachidonic acid cascade, which plays an important role in the metabolism of eicosanoid epoxides.Citation12) sEH catalyzes the hydrolysis of epoxyeicosatrienoic acids (EETs) into the corresponding dihydroxyeicosatrienoic acidsCitation13) and is expressed in various cells and tissues, including the liver, kidney, vascular endothelium, leukocytes, and adipocytes.Citation14–16) EETs have four major isomers: 5,6-EET, 8-9-EET, 11,12-EET, and 14,15-EET, all of which are produced from arachidonic acid by cytochrome P450s (CYPs) in the eicosanoid pathway.Citation17) These isomers are endothelium-derived hyperpolarizing factors with a variety of biological functions, such as anti-inflammatory, antihypertensive, cardioprotective, vasodilatory, and angiogenic effects.Citation18,19) Moreover, several studies have reported other potential anti-inflammatory effects of EETs, in animal models, including decreased production of nitric oxide, cytokines, and pro-inflammatory mediators.Citation18,20) Therefore, sEH inhibitors that decrease EETs levels could serve as novel agents for the treatment of hypertension and inflammation. Recent studies have developed several urea-type sEH inhibitors such as 12-(3-adamantan-1-yl-ureido)-dodecanoic acid butyl ester (AUDA), 1-trifluoromethoxyphenyl-3-(1-acetylpiperidin-4-yl) urea (TPAU), trans-4-[4-(3-adamantan-1-yl-ureido)-cyclohexyloxy]-benzoic acid (t-AUCB), and sorafenib.Citation18) However, several problems have arisen during clinical trials, such as poor solubility and rapid metabolism in microsomes. Therefore, novel sEH inhibitors from natural products that overcome these problems are urgently needed. In the study described herein, we report the isolation of nine phenolic compounds from the rhizomes and roots of G. scabra, as well as their sEH inhibitory activities.
Dried rhizomes and roots (2.5 kg) of G. scabra were extracted with MeOH (10 L × 3) under reflux. The MeOH extract (670.0 g) was suspended in water and partitioned with CHCl3 and n-BuOH. The CHCl3 fraction (90.0 g) was subjected to silica gel (8 × 30 cm) column chromatography with n-hexane–EtOAc (20:1, 5:1), CHCl3–acetone (5:1), and CHCl3–MeOH–H2O (6:1:0, 3:1:0.1), resulting in five fractions (Fr. 1A–1E). Fraction 1D (28.6 g) was then subjected to silica gel (4 × 30 cm) column chromatography with CHCl3–MeOH (20:1, 10:1, 7:1, 5:1, 3:1) elution solvent to yield eight subfractions (Fr. 1D-1–1D-8). Fraction 1D-1 was separated using YMC (1.5 × 80 cm) column chromatography with a MeOH–acetone–H2O (1:1:1–4:4:1) elution solvent to yield compound 6 (23.7 mg; 0.0035%). Fraction 1D-2 was subjected to silica gel (1.0 × 80 cm) column chromatography with CHCl3–MeOH (25:1 to 20:1) elution solvent to yield compounds 2 (39.6 mg; 0.0059%) and 9 (27.0 mg; 0.0040%). Fraction 1D-8 was separated using YMC (1.5 × 80 cm) column chromatography with a MeOH–acetone–H2O (0.3:0.3:1–1:1:1) elution solvent to yield compounds 5 (187.0 mg; 0.028%) and 8 (87.6 mg; 0.013%). The n-BuOH fraction (190.0 g) was subjected to silica gel (8 × 30 cm) column chromatography with CHCl3–MeOH–H2O (12:1:0, 6.5:1:0, 4:1:0, 2.5:1:0.1) to yield four fractions (Fr. 2A–2D). Fraction 1B (15.0 g) was subjected to YMC (3 × 80 cm) column chromatography with a MeOH–H2O (0.05:1–0.95:1) elution solvent to yield eight subfractions (Fr. 2B-1–2B-8). Fraction 1B-3 was subjected to silica gel (2.5 × 80 cm) column chromatography with CHCl3–MeOH (15:1–8:1) elution solvent to yield compounds 1 (9.3 mg; 0.0014%), 3 (169.0 mg; 0.025%), 4 (105.0 mg; 0.016%), and 7 (39.1 mg; 0.0058%).
Compound 1 was obtained as a yellowish powder. The molecular structure of 1 was inferred to be C18H24O9 from a pseudomolecular ion peak [M + Na]+ at m/z 407.1353 in the HR-ESI-MS spectrum (calcd for C18H24O9Na, 407.1313). The 1H-NMR spectrum of 1 (Table ) revealed two aromatic proton signals at δH 7.55 (brs, H-2) and 7.88 (brs, H-6), an olefinic proton signal at δH 5.26 (t, J = 6.8 Hz, H-8), an anomeric proton signal at δH 5.57 (d, J = 6.5 Hz, H-1′), a methylene group signal at δH 3.20 (d, J = 6.8 Hz, H-7), two methyl group signals at δH 1.51 (s, H-10, 11), and among other signals (δH 3.97–4.43) belonging to a sugar moiety. The sugar moiety was identified as d-glucose by acid hydrolysis. The 13C NMR spectrum showed 18 signals, including a carboxyl group [δC 174.9 (COOH)], a benzene ring [δC 117.9 (C-5), 122.2 (C-2), 123.9 (C-6), 130.7 (C-1), 146.6 (C-3), 152.1 (C-4)], a glucosyl group [δC 61.7 (C-6′), 70.8 (C-4′), 74.7 (C-2′), 78.1 (C-3′), 78.5 (C-5′), 103.6 (C-1′)], and a prenyl group [δC 17.5 (C-11), 25.5 (C-10), 33.8 (C-7), 124.0 (C-8), 131.8 (C-9)]. Analyses of the 1H and 13C NMR spectral data indicated that compound 1 was a tetra-substituted phenolic glycoside. The position of substituent groups was determined by HMBC spectrum. Key correlations between H-7 (δH 3.20)/C-2 (δC 122.2), C-6 (δC 123.9), and C-1 (δC 130.7) indicated that the prenyl group was located at C-1. Correlations between H-2 (δH 7.55)/C-3 (δC 146.6) and H-1′ (δH 5.57)/C-3 (δC 146.6) indicated that the glucosyl group was located at C-3. Both H-2 (δH 7.55) and H-6 (δH 7.88) exhibited correlations with C-4 (δC 152.1); however, only H-6 (δH 7.88) correlated with the carboxyl group (δC 174.9), indicating that the carboxyl group was located at C-5 and the hydroxy group was located at C-4 (Fig. (B)). Based on these data, compound 1 was characterized as 4-hydroxy-1-prenyl-5-(3-O-β-d-glucopyranosyl)-benzoic acid.
Table 1. The 1H and 13C NMR spectroscopic data of compounds 1 and 2.
Fig. 1. Structures of compounds 1–9 (A) and 1H-1H COSY and HMBC correlations (B) of compounds 1 and 2.
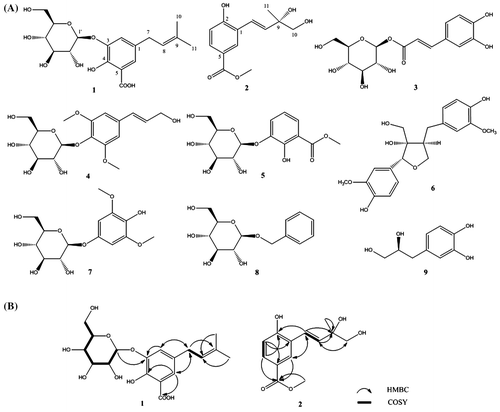
Compound 2 was obtained as a yellowish gum. The molecular formula was defined as C13H16O5 from a pseudomolecular ion peak [M + H]+ at m/z 253.1072 (calcd. 253.1071) in the HR-ESI-MS spectrum. The 1H NMR spectrum of 2 (Table ) revealed three aromatic proton signals at δH 7.11 (d, J = 8.0 Hz, H-3), 7.98 (dd, J = 8.0, 2.0 Hz, H-4), and 8.53 (d, J = 2.0 Hz, H-6), belonging to an ABX system. Two olefinic proton signals at δH 7.01 (d, J = 16.0 Hz, H-8) and 7.80 (d, J = 16.0 Hz, H-7) indicated the presence of a trans double bond. Moreover, an oxymethylene group [δH 3.96 (m, H-10)], a methoxyl group [δH 3.74 (s, OCH3)], and a methyl group [δH 1.67 (s, H-11)] were also observed. Correspondently, the 13C NMR spectrum (Table ) exhibited 13 signals, including a carbonyl group signal at δC 166.9 (COCH3), a benzene ring signal at δC 116.1 (C-3), 123.0 (C-5), 129.0 (C-6), 130.1 (C-4), 130.8 (C-1), and 160.4 (C-2), two olefinic carbon atoms at δC 122.8 (C-7), and 137.5 (C-8), an oxygenated quaternary carbon atom at δC 73.8 (C-9), an oxygenated methylene carbon atom at δC 70.9 (C-10), a methoxyl carbon atom at δC 51.4 (OCH3), and a methyl carbon atom at δC 25.4 (C-11). The HMBC spectrum revealed correlations between H-7 (δH 7.80)/C-9 (δC 73.8), H-8 (δH 7.01)/C-9 (δC 73.8), C-10 (δC 70.9), and C-11 (δC 25.4), and H-11 (δH 1.67)/C-9 (δC 73.8) and C-10 (δC 70.9), which were indicative of a 1,2-dihydroxy-2-methyl-3-butenyl group in compound 2. The absolute configuration of 2 was determined as R by comparing the sign of the optical rotation (+62.8°) with the literature value.Citation21,22) The position of three substituent groups was determined by HMBC spectrum analysis. Key correlations between H-7 (δH 7.80)/C-6 (δC 129.0), C-1 (δC 130.8), and C-2 (δC 160.4) indicated that the 1,2-dihydroxy-2-methyl-3-butenyl group was located at C-1. Correlations between H-4 (δH 7.98) and H-6 (δH 8.53)/C = O (δC 166.9) indicated that the carboxyl methyl ester group was attached to C-5. In addition, correlations between H-4 (δH 7.98), H-6 (δH 8.53), and H-7 (δH 7.80)/C-2 (δC 160.4) revealed that the hydroxy group was located at C-2 (Fig. (B)). Accordingly, the structure of compound 2 was determined to be (R)-2-hydroxy-1(1,2-dihydroxy-2-methyl-3-butenyl)-5-benzoic acid methyl ester.
By comparing our physical and spectroscopic data with those reported in the literature, the other known compounds were identified as 1-O-caffeoyl glucose (3),Citation23) siringin (4),Citation24) 3-(β-d-glucopyranosyloxy)-2-hydroxy-benzoic acid methyl ester (5),Citation4) berchemol (6),Citation25) koaburaside (7),Citation26) benzyl-O-β-d-glucopyranoside (8),Citation27) and (2S)-3-(4-hydroxy-3-methoxyphenyl)-1,2-propanediol (9).Citation28) Here, we report for the first time the isolation of compounds 6–9 from G. scabra (Fig. (A)).
The inhibitory activity of isolated compounds against sEH was also evaluated. The amount of 6-methoxy-2-naphthaldehyde produced from the substrate (PHOME) was quantified in the presence or absence of compounds 1–9 using a fluorescence photometer at wavelengths of 330 and 465 nm, respectively.Citation29,30) AUDA served as a positive control (IC50 = 14.2 ± 3.2 nM). First, the inhibitory activity of all nine compounds was evaluated against 50 μM sEH. Compounds 2, 6, and 9 exhibited the highest inhibitory activities, with inhibition effects of 73.2 ± 1.3, 114.3 ± 2.9, and 68.8 ± 4.5% of the control value, respectively. In contrast, compounds 1, 3–5, and 7 demonstrated moderate inhibitory activity, with inhibition effects ranging from 41.1 to 53.3% of the control value. Compound 8 lacked inhibitory activity in the bioassay system (Table ). Compounds 2, 6, and 9 were further selected to examine IC50 values using a dose-dependent evaluation. Results showed that compounds 2, 6, and 9 exerted sEH inhibitory activity in dose-dependent manner, with IC50 values of 27.3 ± 0.8, 8.2 ± 2.1, and 26.9 ± 1.7, respectively (Table ; Supplementary Material: Fig. S1). Interestingly, upon examination of the structure–activity relationship of isolated phenolic compounds, we found that compounds 2, 6, and 9, which exhibited highest inhibitory activities, contain more hydroxy groups than the other compounds. Correspondently, compounds 1, 3–5, and 7, which showed moderate inhibitory effects, contain only one or two hydroxy groups. In contrast to compound 8, which lacks a hydroxy group, does not exhibit inhibitory activity. Therefore, the number of hydroxy groups in these structures may represent a key functional element.
Table 2. The sEH inhibitory activities of compounds 1–9.
Lineweaver–Burk plots were used to determine the inhibitory mode of compounds 2, 6, and 9. Compounds 2 and 6 demonstrated changes in the apparent Vmax and km values, indicating that these compounds induce a mixed type of inhibition (Supplementary Material: Fig. S2-A and B), whereas compound 9 only changed the apparent Vmax value (Supplementary Material: Fig. S2-C). Therefore, we conclude that 9 is a noncompetitive inhibitor. Moreover, Ki (free enzyme) and Kis (enzyme–substrate complex) binding constants were obtained from the secondary replots of slope and y-intercept vs. [I], which were fitted to Equations (2) and (3) (Supplementary Material: Fig. S2). Table indicates their values as follows: compound 3, Ki = 23.7 ± 2.1 μM and Kis = 2.1 ± 0.7 μM; compound 6, Ki = 4.2 ± 1.1 and Kis = 0.5 ± 1.3 μM; compound 9 Ki = 11.7 ± 2.7 μM).
In this study, nine phenolic compounds (1–9) were isolated from G. scabra. Their inhibitory effects on sEH were further evaluated. To our knowledge, this is the first report on the phenolic components of G. scabra and their inhibitory effects on sEH. Notably, our data suggest that phenolic components may represent new bioactive components of this plant and provide a scientific basis for the complement of the active components in G. scabra. In particular, compounds 2, 6, and 9 may have potential as natural sEH inhibitors or as lead molecules for developing new inhibitors sEH.
Supplemental material
The supplemental material for this paper is available at http://dx.doi.org/10.1080/09168451.2014.1002451.
Supplemental Materials
Download MS Word (133.3 KB)Additional information
Funding
References
- Wang Z, Wang C, Su T, Zhang J. Antioxidant and immunological activities of polysaccharides from Gentiana scabra Bunge roots. Carbohydr. Polym. 2014;112:114–118.10.1016/j.carbpol.2014.05.077
- Ikeshiro Y, Tomita Y. A new bitter secoiridoid glucoside from Gentiana scabra var. buergeri. Planta Med. 1983;48:169–173.10.1055/s-2007-969915
- Zhang HL, Xue SH, Pu F, Tiwari RK, Wang XY. Establishment of hairy root lines and analysis of gentiopicroside in the medicinal plant Gentiana macrophylla. Russ. J. Plant Physiol. 2010;57:110–117.10.1134/S1021443710010152
- Ikeshiro Y, Mase I, Tomita Y. A secoiridoid glucoside from Gentiana scabra var. buergeri. Planta Med. 1990;56:101–103.10.1055/s-2006-960897
- Tan RX, Wolfender JL, Zhang LX, Ma WG, Fuzzati N, Marston A, Hostettmann K. Acyl secoiridoids and antifungal constituents from Gentiana macrophylla. Phytochemistry. 1996;42:1305–1313.10.1016/0031-9422(96)00149-5
- Bergeron C, Marston A, Gauthier R, Hostettmann K. Iridoids and secoiridoids from Gentiana linearis. Phytochemistry. 1997;44:633–637.10.1016/S0031-9422(96)00636-X
- Kakuda R, Iijima T, Yaoita Y, Machida K, Kikuchi M. Secoiridoid glycosides from Gentiana scabra. J. Nat. Prod. 2001;64:1574–1575.10.1021/np010358o
- Kakuda R, Iijima T, Yaoita Y, Machida K, Kikuchi M. Triterpenoids from Gentiana scabra. Phytochemistry. 2002;59:791–794.10.1016/S0031-9422(02)00021-3
- Kim JA, Son NS, Son JK, Jahng Y, Chang HW, Jang TS, Na M, Lee SH. Two new secoiridoid glycosides from the rhizomes of Gentiana scabra Bunge. Arch. Pharm. Res. 2009;32:863–867.10.1007/s12272-009-1608-0
- Rojas A, Bah M, Rojas JI, Gutiérrez DM. Smooth muscle relaxing activity of gentiopicroside isolated from Gentiana spathacea. Planta Med. 2000;66:765–767.10.1055/s-2000-9774
- Kumarasamy Y, Nahar L, Sarker SD. Bioactivity of gentiopicroside from the aerial parts of Centaurium erythraea. Fitoterapia. 2003;74:151–154.10.1016/S0367-326X(02)00319-2
- Amano Y, Yamaguchi T, Tanabe E. Structural insights into binding of inhibitors to soluble epoxide hydrolase gained by fragment screening and X-ray crystallography. Bioorg. Med. Chem. 2014;22:2427–2434.10.1016/j.bmc.2014.03.001
- Newman JW, Morisseau C, Hammock BD. Epoxide hydrolases: their roles and interactions with lipid metabolism. Prog. Lipid Res. 2005;44:1–51.10.1016/j.plipres.2004.10.001
- Pacifici GM, Temellini A, Giuliani L, Rane A, Thomas H, Oesch F. Cytosolic epoxide hydrolase in humans: development and tissue distribution. Arch. Toxicol. 1988;62:254–257.10.1007/BF00332483
- Spector AA, Fang X, Snyder GD, Weintraub NL. Epoxyeicosatrienoic acids (EETs): metabolism and biochemical function. Prog. Lipid Res. 2004;43:55–90.10.1016/S0163-7827(03)00049-3
- Shen HC, Hammock BDJ. Discovery of inhibitors of soluble epoxide hydrolase: a target with multiple potential therapeutic indications. J. Med. Chem. 2012;55:1789–1808.10.1021/jm201468j
- Sudhahar V, Shaw S, Imig JD. Epoxyeicosatrienoic acid analogs and vascular function. Curr. Med. Chem. 2010; 17:1181–1190.
- Imig JD, Hammock BD. Soluble epoxide hydrolase as a therapeutic target for cardiovascular diseases. Nat. Rev. Drug Discovery. 2009;8:794–805.10.1038/nrd2875
- Zhang W, Otsuka T, Sugo N, Ardeshiri A, Alhadid YK, Iliff JJ, DeBarber AE, Koop DR, Alkayed NJ. Soluble epoxide hydrolase gene deletion is protective against experimental cerebral ischemia. Stroke. 2008;39:2073–2078.10.1161/STROKEAHA.107.508325
- Schmelzer KR, Kubala L, Newman JW, Kim IH, Eiserich JP, Hammock BD. Soluble epoxide hydrolase is a therapeutic target for acute inflammation. Proc. Nat. Acad. Sci. USA. 2005;102:9772–9777.10.1073/pnas.0503279102
- Ishizaki M, Soai K, Yokoyama S. Asymmetric synthesis of the both enantiomers of tertiary aryl homoallyl alcohols and diols by diastereoselective addition of allyltrimethylsilane to chiral α-keto imides. Chem. Lett. 1987;16:341–334.
- Casolari S, D’Addari D, Tagliavini E. BINOL-Ti-catalyzed synthesis of tertiary homoallylic alcohols: the first catalytic asymmetric allylation of ketones. Org. Lett. 1999;1:1061–1063.10.1021/ol990858x
- Que M, Su YF, Yan SL, Zhou YH, Gao XM. Two new phenylpropanoid glycosides from the roots of Aruncus sylvester. J. Asian Nat. Prod. Res. 2014;16:158–162.10.1080/10286020.2013.841676
- Li W, Yang SY, Yan XT, Sun YN, Song SB, Kang HK, Kim YH. NF-κB inhibitory activities of glycosides and alkaloids from Zanthoxylum schinifolium stems. Chem. Pharm. Bull. 2014;62:196–202.10.1248/cpb.c13-00759
- Tan RX, Jakupovic J, Jia ZJ. Aromatic constituents from Vladimiria souliei. Planta Med. 1990;56:475–477.10.1055/s-2006-961015
- Yoshikawa K, Sugawara S, Arihara S. Phenylpropanoids and other secondary metabolites from fresh fruits of Picrasma quassioides. Phytochemistry. 1995;40:253–256.10.1016/0031-9422(95)00234-X
- Yan XT, Lee SH, Li W, Sun YN, Yang SY, Jang HD, Kim YH. Evaluation of the antioxidant and anti-osteoporosis activities of chemical constituents of the fruits of Prunus mume. Food Chem. 2014;156:408–415.10.1016/j.foodchem.2014.01.078
- Fan CQ, Yue JM. Biologically active phenols from Saussurea medusa. Bioorg. Med. Chem. 2003;11:703–708.10.1016/S0968-0896(02)00470-4
- Lee GH, Oh SJ, Lee SY, Lee JY, Ma JY, Kim YH, Kim SK. Discovery of soluble epoxide hydrolase inhibitors from natural products. Food Chem. Toxicol. 2014;64:225–230.10.1016/j.fct.2013.11.042
- Moser D, Achenbach J, Klingler FM, Estel la B, Hahn S, Proschak E. Evaluation of structure-derived pharmacophore of soluble epoxide hydrolase inhibitors by virtual screening. Bioorg. Med. Chem. Lett. 2012;22:6762–6765.10.1016/j.bmcl.2012.08.066