Abstract
Annexin A4 (Anx4) is a cytosolic calcium-binding protein with four repeat domains, each containing one calcium-binding site (CBS). The protein interacts with the phospholipid membrane through the CBS-coordinated calcium ion, although the role of each CBS in the calcium-dependent association is unclear. To determine the role of each CBS, 15 CBS-abolished variants were produced in various combinations by substitution of a calcium-liganding residue on each CBS by Ala. Various mutant combinations produced different influences on calcium-dependent membrane-binding behavior and on the sodium-dependent dissociation of membrane-bound Anx4. Our data suggest the interaction of Anx4 with the lipid membrane consists of strong and weak interactions. CBSs I and IV mediate formation of strong interactions, while CBSs II and III are important for weak interactions. We also suggest Anx4 binds the lipid membrane through CBSs I and IV in the cytoplasmic fluids.
Graphic Abstract
We suggest Anx4 associates with the lipid membrane via the calcium ion in calcium binding sites I and IV in the cytoplasmic fluid.

Annexins are calcium-dependent phospholipid-binding proteins.Citation1) They are constructed from fourfold or eightfold imperfect repeat domains.Citation1−4) Annexin A4 (Anx4) possesses four annexin repeat domains, each with one calcium-binding site (CBS) (Fig. ),Citation5,6) and interacts with lipid membranes in a calcium-dependent manner.Citation7,8) Anx4 is localized to the cytoplasm in a variety of cells, including epithelial and secretory cells of the liver, brain, pancreas, intestine, adrenal medulla, and kidney.Citation9−11) In a previous study, PSORT and hydropathy plot analyses demonstrated that rat Anx4 is a cytosolic peripheral membrane protein bound to the cytosolic leaflet of zymogen granules in pancreatic acinar cells.Citation12)
Fig. 1. Schematic representation of Anx4.
Notes: Image produced with PyMOLCitation33) using the PDB data (PDB entry 2ZHJ) for annexin IV.Citation20) Arrows represent the positions of CBS I, II, III, and IV, respectively; N and C indicate the N- and C-termini. Spheres represent the mutated residue on each CBS.
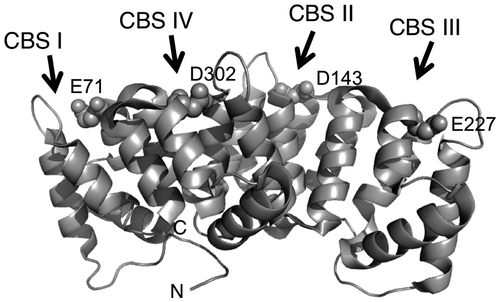
Various annexin crystal structures show that the CBS domains coordinate calcium ions.Citation13) The crystal structure of annexin A5 showed that the coordinated calcium ion on the CBS interacts with the lipid head group, thus indicating that membrane association of annexins is mediated by the CBS-coordinated calcium.Citation14) The Anx4 crystal structure also showed the CBS domains coordinate calcium.Citation15) The differential lipid specificities of the repeat domains of Anx4 were explored by creating various CBS mutations.Citation16) The results of these studies suggest lipid binding occurs through and is dependent upon CBS-coordinated calcium in Anx4. What remains unclear is whether all CBS domains share the same role in lipid association. Anx4 binds via CBS III to surfactant protein A.Citation17,18) Annexin A2 is a selective receptor for a novel surfactant A-binding cytotoxin synthesized by Mycoplasma pneumonia.Citation19) We hypothesize that the orientation of Anx4 on the lipid membrane and its interaction with other proteins are important to understanding its physiological function. Although the physiological function of Anx4 is unclear, we believe Anx4 function is expressed when the protein associates with the lipid membrane.
Lipid association is nearly abolished in the presence of sodium chloride at concentrations exceeding 400 mM.Citation7) The crystal structure of Anx4 shows that the CBSs also coordinate sodium.Citation20) From these evidences, we deduced that sodium ion is important factor to understand the role of each CBS in the association. The sodium-dependent dissociation of Anx4 would help us understand the role of each CBS in calcium-dependent lipid association. In this study, we used 15 CBS mutants to explore the role of each domain in calcium-dependent lipid association. The variants were constructed by substituting a calcium-liganding residue on each CBS with Ala. Differences in the behavior of these Anx4 variants in calcium-dependent association and sodium-dependent dissociation revealed the role of each CBS on the Anx4–lipid association.
Materials and methods
Materials
Recombinant wild-type Anx4 was prepared from pGEX3X, which carries the rat Anx4 gene.Citation12) Fifteen recombinant variants were produced in expression vectors and purified as described below. Enzymes for cloning were purchased from TaKaRa Bio Inc (Shiga, Japan). Factor Xa and purification columns were obtained from GE Healthcare Japan Co (Tokyo, Japan). Egg yolk phosphatidylcholine and bovine brain phosphatidylserine were obtained from Sigma–Aldrich (MO, USA). Other chemicals were purchased from Wako Pure Chemical Industries (Osaka, Japan).
Site-directed mutagenesis
Anx4 variants were constructed using a site-directed mutagenesis kit (QuikChange, Stratagene, CA, USA). Mutagenic primers (Hokkaido System Science, Sapporo, Japan) are listed in Table and were used according to manufacturer instructions. Each variant carries a single, double, triplet, or quadruple substitution, in which CBS residues Glu71, Asp143, Glu227, and/or Asp302 were replaced with Ala and were denoted as summarized in Table . Each site-directed mutant was verified by restriction digestion and DNA sequencing (ABI PRISM 310, Life Technologies, CA, USA). The mature coding sequence was inserted into the BamHI site of pGEX3, and proper orientation of the clones was verified by DNA sequencing. The expression vectors were used as the framework for production of the variants.
Table 1. Primers for the substitution of the residue on CBSs of annexin IV.
Table 2. Summarized variant species of annexin IV.
Preparation of recombinant proteins
Escherichia coli HB101 was transformed with each expression vector to produce glutathione S-transferase-tagged recombinant proteins that were expressed and purified as described previously.Citation20) Purified proteins were concentrated, and the buffer was exchanged to 20 mM Tris-HCl, pH 7.4 by centrifugal filtration (Amicon Ultra-10, Millipore, MA, USA).
Vesicle preparation
Lipid vesicles were prepared by sonication of an equimolar phospholipid mixture composed of phosphatidylcholine and phosphatidylserine as described by Hu et al.Citation21) with a slight modification. The mixed phospholipids were dried under nitrogen and then suspended in 20 mM Tris-HCl, pH 7.4 with a sonication on ice for 15 min. Small unilamellar vesicles were used within 1 h after preparation.
In vitro assay for membrane association and dissociation
We used an in vitro assay to assess calcium-dependent association of Anx4: 100 μg lipid vesicles and 2 μg recombinant protein were incubated at 25 °C for 1 h in 20 mM Tris-HCl, pH7.4, containing 0–500 μM calcium in a final volume of 20 μL. For wild-type Anx4, the association assay was carried out in the presence or absence of 150 mM NaCl. For the variants, the association assay was carried out in the absence of NaCl. Calcium chloride was added into the lipid–protein mixture. The mixture was centrifuged at 15,000 × g for 20 min to yield the membrane-bound fraction (pellet) and the non-membrane-bound fraction (supernatant). The pellets were washed three times with 20 mM Tris-HCl, pH7.4 in a volume of 200 μl and resuspended in 20 mM Tris-HCl, pH7.4, containing 1 mM EDTA in a final volume of 20 μl. The pellets and supernatants were separated by SDS-PAGECitation22) and stained with 0.25% Coomassie Brilliant Blue R-250. Band intensity was determined using Image J.Citation23) The ratio of membrane-bound proteins was estimated by dividing the band intensity of the pellets by the total of the band intensity of the pellets and supernatants. The ratio was expressed as the percentage.
For the in vitro dissociation assay, membrane-bound proteins were prepared in 20 mM Tris-HCl, pH7.4, containing 2 mM calcium chloride as described above. The membrane-bound fractions were washed three times in 20 mM Tris-HCl, pH7.4 and then suspended and incubated at 25 °C for 1 h in 20 mM Tris-HCl, pH7.4, containing 0–500 mM sodium, potassium, or magnesium chloride. The mixture was centrifuged at 15,000 × g for 20 min to separate the membrane-bound and unbound fractions and then quantified as above. In the dissociation assay for the CBS-AAAA variant, in which all CBSs are abolished, the ratio of membrane-bound variants was calculated by dividing the band intensity of the membrane-bound protein by that of the membrane-bound wild-type protein in the presence of 2 mM calcium chloride. Data represent the average ± standard deviation of three independent experiments.
In the association and dissociation assays, data were fitted using the smooth command or the command for the Michaelis–Menten equation of a graphic soft (KareidaGraph 4.5, Synergy Software, PA).
Results
Calcium-dependent association of the wild-type protein
The association of wild-type Anx4 with lipid vesicles was measured in the presence of calcium chloride with or without sodium chloride (Fig. ). In the absence of sodium chloride, the wild-type protein and vesicles bound in a two-step behavior, calcium-dependent manner that yielded an inflection in the calcium chloride concentration curve from 40 to 100 μM (Fig. , open circles). The transition reached a plateau at approximately 100 μM (Fig. , open circles). This dual-inflection binding behavior is the result of dual binding mechanisms.
Fig. 2. Calcium-dependent membrane association of Anx4.
Notes: Membrane association by wild-type Anx4 was investigated in various concentrations of calcium in the absence (open circles) and presence (closed circles) of 150 mM sodium chloride. Data represent the average ± standard deviation of three independent experiments.
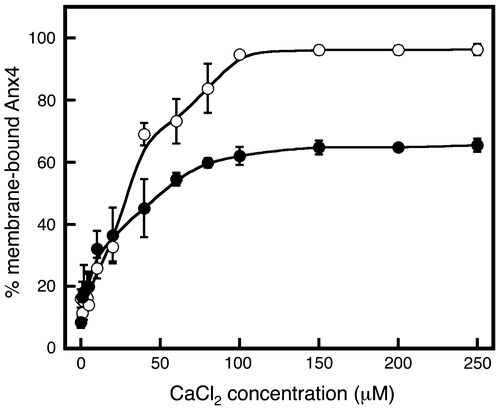
In the presence of sodium chloride, the maximum ratio of bound protein decreased by approximately 40% (Fig. , closed circles). The calcium chloride concentration curve weakly showed an inflection from 20 to 60 μM. Thus, the binding interaction is diminished by adding sodium chloride. We concluded that the calcium-dependent binding behavior in the absence of sodium chloride was derived from a combination of strong and weak interactions.
Metal-dependent dissociation of wild-type protein from membrane vesicles
Protein binding to lipid vesicles occurs through different interactions, each of which yields a different dissociation curve. The dissociation behaviors of the bound proteins were investigated by adding various concentrations of sodium, potassium, and magnesium chlorides (Fig. ). Sodium chloride at concentrations exceeding 400 mM yielded nearly complete release of Anx4 from the membrane vesicles (Fig. , closed circles), consistent with a previous report.Citation7) Sodium chloride at 150 mM reduced Anx4 binding by approximately 40% (Fig. , closed circles). This is consistent with the results of the association experiment at calcium chloride concentration of more than 100 μM in the presence of 150 mM sodium chloride (Fig. , closed circles). Furthermore, it was clear that Anx 4 was gradually released at sodium concentrations up to 200 mM, after which the dissociation of Anx4 drastically increased. These dissociation patterns suggest the bound proteins interact with membrane vesicles via different mechanisms, one susceptible to low concentrations of sodium chloride and the other to higher concentrations exceeding 200 mM.
Fig. 3. Metal-dependent dissociation of Anx4.
Notes: Anx4 dissociation was investigated at various concentrations of sodium chloride (closed circles and solid line), magnesium chloride (open triangles and dashed line), and potassium chloride (open squares and dotted line). Data represent the average ± standard deviation of three independent experiments.
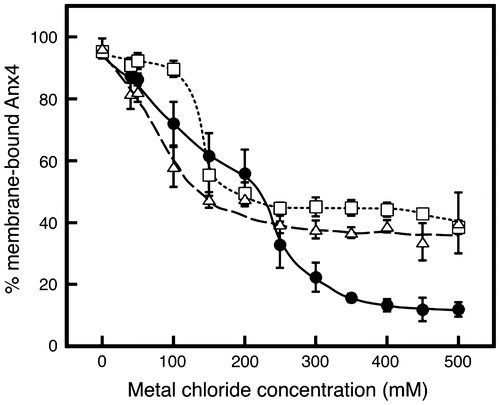
Anx4 is a cytosolic membrane-bound protein. Potassium is generally present at higher concentrations than sodium in cytoplasmic fluids. Dissociation of Anx4 was investigated in the presence of various concentrations of potassium chloride (Fig. , open squares). Interestingly, the bound proteins were largely unaffected by adding potassium at concentrations less than 100 mM, but sharply decreased to a plateau at approximately 40% at 100–150 mM. The transition indicates that Anx4 association with lipid vesicles is mediated by an interaction that is abolished by a lower concentration of potassium and another that remains stable at higher concentrations. Thus, the interaction is composed of a combination of different interactions that show different sensitivity to potassium ion.
Calcium is a divalent cation, but sodium and potassium ions are monovalent. To observe the effect of divalent cations on dissociation, we added magnesium chloride in various concentrations (Fig. , open triangles). The bound proteins gradually decreased to a plateau of about 40% by addition of 150 mM, similar to the effect of potassium. These results support the conclusion that the Anx4 association with lipid vesicles is mediated by strong and weak interactions.
Association and dissociation of the quadruple annexin IV variant
The CBS-AAAA variant, in which the residues Glu71 on CBS I, Asp143 on CBS II, Glu227 on CBS III, and Asp302 on CBS IV are mutated to Ala, gradually bound the lipid vesicles with increasing calcium chloride to a plateau of 40% at 200 μM (Fig. (A)). The bound CBS-AAAA variant was rapidly released by low-concentration sodium chloride (Fig. (B)). The weak interaction may be induced by an incomplete diminishment of calcium coordination on CBSs or by calcium coordination on other regions.
Fig. 4. Calcium-dependent association and sodium-dependent dissociation of variants with a quadruple substitution.
Notes: (A) Membrane association of variants with a quadruple substitution, CBS-AAAA was investigated in various concentrations of calcium. (B) Dissociation of CBS-AAAA was investigated in various concentrations of sodium chloride. The ratio was expressed as the percentage of bound CBS-AAAA to bound wild-type Anx4 in the presence of 200 μM calcium chloride. Data represent the average ± standard deviation of three independent experiments. The proportion of membrane-bound variants was calculated by dividing the band intensity of the membrane-bound protein by that of the membrane-bound wild-type protein in the presence of 2 mM calcium chloride.
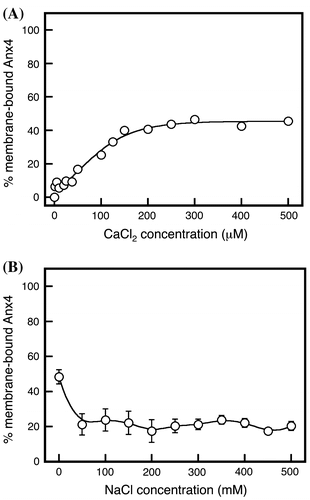
Association and dissociation of triplet variants
Four variants were produced, each with a triplet substitution one native CBS (Table ). CBS-1AAA (closed circles) and CBS-AAA4 variants (open triangles) rapidly bound to lipid vesicles to plateau at approximately 100 μM (Fig. (A)), similar to wild-type protein, but without the inflection (Fig. , closed circles). Bound CBS-A2AA variant bound gradually to a plateau of approximately 70% at 200 μM (Fig. (A), open circles). The CBS-AA3A variant was more gently bound (Fig. (A), closed triangles). These results show that CBS I and IV are the main influences on the association and that membrane association occurs in the order of CBS-AAA4 ≤ CBS-1AAA < CBS-A2AA < CBS-AA3A, suggesting calcium ion is coordinated in the order of CBS IV, CBS I, CBS II, and CBS III.
Fig. 5. Calcium-dependent association and sodium-dependent dissociation of variants with a triplet substitution.
Notes: (A) Membrane association of variants with a triplet substitution in various concentrations of calcium: CBS-1AAA (closed circles and solid line), CBS-A2AA (open circles and short dashed line), CBS-AA3A (closed triangles and long dashed line), and CBS-AAA4 (open triangles and dotted dashed line). (B) Membrane dissociation of CBS-1AAA (closed circles and solid line), CBS-A2AA (open circles and short dashed line), CBS-AA3A (closed triangles and long dashed line), and CBS-AAA4 (open triangles and dotted line) was investigated in various concentrations of sodium. Data represent the average ± standard deviation of three independent experiments.
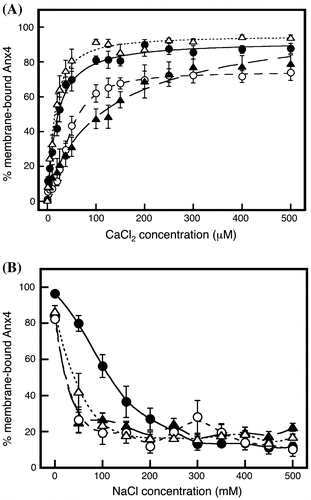
Variant dissociation was analyzed by adding sodium chloride at various concentrations (Fig. (B)). The bound CBS-1AAA variant was gradually released by increasing sodium chloride (Fig. (B), closed circles). In contrast, binding of the other triplet variants sharply decreased, although CBS-AAA4 (Fig. (B), open triangles) dissociation was more gradual than that of CBS-A2AA (Fig. (B), open circles) and CBS-AA3A (Fig. (B), closed triangles). Thus, the physicochemical properties of the interaction through CBS I are much stronger and clearly different from those of the other CBSs, and the CBS IV interaction is a little stronger than the interactions of CBSs II and III.
Association and dissociation of single substitution variants
The CBS-A234 variant exhibited a remarkable calcium-dependent association over a calcium range of 0–100 μM, after which the variant showed a gradual association with maximum binding of approximately 60% (Fig. (A), closed circles). As shown in Fig. , CBS I mediates the stronger interaction. The CBS-12A4 variant also exhibited a binding curve in a two-step mode (Fig. (A), closed triangles), while CBS-1A34 (Fig. (A), open circles) and 123A (Fig. (A), open triangles) bound in a single-step mode. From these results, CBS I was deduced to be important for the formation of the comparatively stronger interaction.
Fig. 6. Calcium-dependent association and sodium-dependent dissociation of variants with a single substitution.
Notes: Calcium-dependent association (A) and sodium-dependent dissociation (B) of variants with a single substitution: CBS-A234 (closed circles and solid line), CBS-1A34 (open circles and short dashed line), CBS-12A4 (closed triangles and dotted line), and CBS-123A (open triangles and long dashed line). Data represent the average ± standard deviation of three independent experiments.
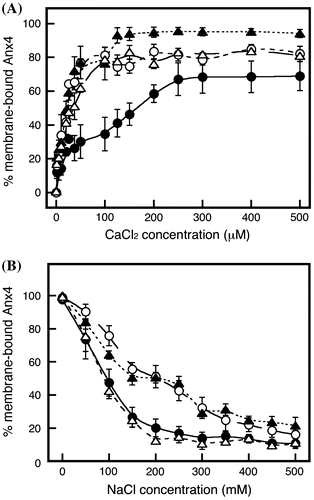
CBS-A234 (closed circles) and CBS-123A (open triangles) were released in a linear fashion with increasing concentrations of sodium chloride up to 200 mM. In contrast, CBS-12A4 (closed triangles) and CBS-1A34 (open circles) were not easily released at concentration of 150–300 mM. The different patterns suggest the possibility that CBS I and CBS IV together play an important role in formation of the strong interaction, while CBSs II and III mediate the weak interaction.
Analysis of doubly substituted CBS variants
We produced six doubly substituted annexin IV variants (Table ). CBS-12AA (Fig. (A), open circles) and CBS-A2A4 (Fig. (B), closed squares) achieved a binding ratio of approximately 60%. The ratios of bound CBS-AA34 (Fig. (A), closed circles) and CBS-1A3A (Fig. (B), open squares) proteins plateaued at approximately 70% in similar patterns. The ratio of bound CBS-1AA4 drastically increased to 100% with increasing calcium chloride (Fig. (C), open triangles), whereas the ratio of bound CBS-A23A increased gradually to approximately 70% (closed triangles). Combining the patterns of CBS-1AA4 and CBS-A23A reveals a two-step binding mechanism similar to that of wild-type proteins as shown in Fig. .
Fig. 7. Calcium-dependent association and sodium-dependent dissociation of variants with a double substitution.
Notes: Calcium-dependent association (A, B, and C) and sodium-dependent dissociation (D, E, and F) were investigated. In panels A and D, CBS-12AA and CBS-AA34 are represented by open circles and a dashed line and by closed circles and a solid line, respectively. In panels B and E, CBS-1A3A and CBS-A2A4 are represented by open squares and a dashed line and by closed squares and a solid line, respectively. In panels C and F, CBS-1AA4 and CBS-A23A are represented by open triangles and a dashed line and by closed triangles and a solid line, respectively. Data represent the average ± standard deviation of three independent experiments.
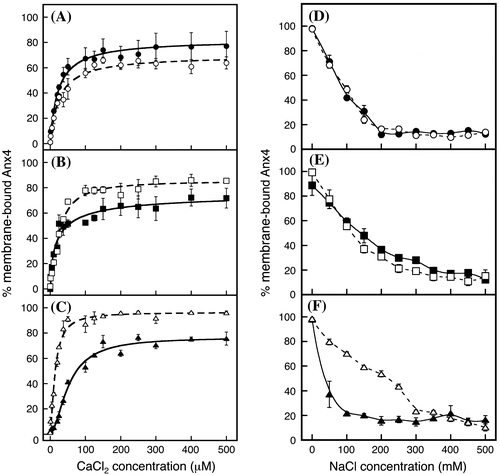
Bound CBS-12AA (open circles) and CBS-AA34 (closed circles) variants were gently released by an increase of sodium chloride (Fig. (D)). The CBS-1A3A (open squares) and CBS-A2A4 (closed squares) variants were released more gently than the CBS-1A3A and CBS-A2A4 variants (Fig. (E)). Bound CBS-1AA4 did not easily dissociate at sodium concentrations of 150–300 mM (Fig. (F), open triangles). In contrast, the stability of CBS-A23A binding sharply decreased (Fig. (F), closed triangles). Thus, the coordinated calcium in CBS I and IV is important for the strong interaction.
Discussions
Annexins are calcium-dependent phospholipid membrane-binding proteins and are constructed from characteristic repeat domains.Citation1) Anx4 coordinates calcium ion on four CBSs,Citation6,15,24,25) which mediate lipid membrane association.Citation16) Crystal structures show that annexin V binds to the polar head of phosphatidylserine through a coordinated calcium ion;Citation14) thus, Anx4 likely also binds the membrane through CBS-coordinated calcium ions, although the kinetics of the association are unknown. In this study, we identified a two-step, calcium-dependent association mechanism for wild-type Anx4 (Fig. , open circles). The ratio of bound proteins decreased in the presence of sodium chloride (Fig. , closed circle), which, at increasing concentrations, yielded the dissociation curve in a two-step mode (Fig. , closed circles).
The calcium-dependent association and sodium-dependent dissociation curves of various CBS mutants (Figs. ) suggest the two-step curve is the result of strong and weak interactions (Fig. (A) and (B)). As shown in Fig. , the stronger interaction is mediated by the coordinated calcium ions in CBSs I and IV (Fig. (A)); the weaker interaction is formed through the coordinated ions in CBSs II and III (Fig. (B)). The role of each CBS is consistent with that described by Sohma et al.Citation16) and by Nelson and CreutzCitation26). The stronger interactions are broken by sodium concentrations > 200 mM, but not by potassium or magnesium (Fig. ). In contrast, the weaker interaction is broken in the presence of 50–200 mM sodium and magnesium chlorides, and 100–150 mM potassium chloride (Fig. ). Sodium-mediated dissociation of the wild-type protein showed incomplete dissociation at >400 mM sodium chloride (Fig. , closed circles).
Fig. 8. Schematic model of the association and dissociation of Anx4.
Notes: Schematic models for loss of the stronger interaction (A), loss of the weaker interaction (B), and the manner of the association in cytoplasmic fluid (C). In each model, Anx4 is drawn as shown in Fig. . Symbols I, II, III, and IV represent CBS I, II, III, and IV, respectively. Light gray bars show the lipid membrane. Small black and gray circles represent calcium and sodium ions, respectively.
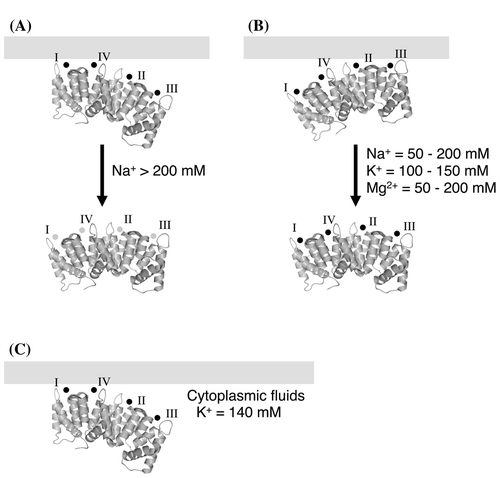
As shown in the association experiments with triplet variants (Fig. (A)), loss of a single native CBS generated different initial increases in calcium-dependent association in the order of CBS-AAA4, CBS-1AAA, CBS-A2AA, and CBS-AA3A with increasing concentrations of sodium chloride (Fig. (A)). Thus, calcium ion is coordinated in the order: CBS IV, CBS I, CBS II, and CBS III. Coordination on CBSs I and IV occurred at almost similar concentrations (Fig. (A)), both lower than CBSs II and III. When metal ions are coordinated by the CBSs, the loops around CBSs II and III move, although the loop around CBS I moves very little.Citation20) This structural motion might be related to the order of the coordination of the calcium ion. In contrast, the bound proteins were released in the order of CBS-A2AA ~ CBS-AA3A < CBS-AAA4 << CBS-1AAA with increasing concentrations of sodium chloride (Fig. (B)). Thus, the interaction decreases with increasing sodium concentrations, although the mechanism is unclear. We previously showed that Anx4 coordinates sodium in the CBSs.Citation20) When the interaction between protein and membrane is broken by substitution of the calcium ion for a sodium ion, the calcium coordination is also broken. The dissolution of the calcium coordination would be thermodynamically unfavorable. In other words, the substitution may be difficult to be carried out. The concentration of sodium required to break the association (approximately 200 mM, Fig. , closed circles) is, however, more than 2,000 times the concentration of calcium ion required for its formation (approximately 100 μM, Fig. , open circles). When the bound proteins are released from the lipid vesicles, the calcium concentration in the buffer is very low. A calcium-released protein coordinates sodium ion in the CBS for structural stabilization.Citation27) The exchange of calcium for sodium ion may provide structural stabilization to the calcium-free form. As shown in Fig. , the stronger interaction begins to break down at approximately 200 mM sodium chloride (closed circles). The weaker interaction is gradually diminished by adding < 200 mM sodium ion. These dissociation patterns suggest the calcium ions in CBSs I and IV are replaced with sodium at concentrations > 200 mM (Fig. (A)). The substitution on CBSs I and IV may occur more frequently at higher concentrations of sodium than of calcium. In contrast, the interaction through CBSs II and III may be broken by loss of the coordinate bond between the calcium ion and the lipid head group at sodium concentrations less than 200 mM.
The CBS-1AA4 variant bound to membrane at a lower concentration of calcium chloride than the wild-type protein (Figs. and (C)). If each CBS competitively coordinates calcium ion with each other, CBSs I and IV of CBS-1AA4 variant would coordinate calcium ion at a lower concentration than those of wild-type protein. It may be induced by the different competition of the calcium coordination between wild-type Anx4 and the CBS-1AA4 variant. The phenomenon implies that CBSs I and IV are an important site for the membrane association.
The CBS-AAAA variant was also bound to lipid membrane with very weak interaction (Fig. ). The weak interaction may be induced by an incomplete diminishment of calcium coordination on CBSs or by calcium coordination on other regions. Although in previous study, Sohma et al.Citation16) have investigated on the calcium-dependent association using various CBS mutants, CBS-AAA is not used in the study. It is unclear that the coordination of calcium on each CBS is completely diminished by the mutation of these residues. In addition, it is reported that annexin A1 coordinates calcium ion on the other region.Citation28) Although the coordination is not reported in Anx4, it shows a possibility that the interaction of CBS-AAAA variant is induced by such as the calcium coordination on other regions.
Anx4 is a cytosolic protein found in a variety of tissues including epithelial and secretory cells of the liver, brain, placenta, pancreas, intestine, adrenal medulla, and kidney.Citation9–12) We previously demonstrated that Anx4 is a peripheral membrane protein bound to the cytosolic leaflet of zymogen granules in exocrine pancreas.Citation12,29) Cytoplasmic concentrations of sodium, potassium, and calcium ions are approximately 10, 140, and 0.1 mM.Citation30) In the presence of 0.1 mM calcium ion, Anx4 was associated with the lipid membrane (Fig. ). Low sodium ion concentrations had little influence on protein-membrane association (Fig. , closed circles). The cytoplasmic concentration of potassium ion is sufficient to release Anx4 bound by weaker interactions to the lipid membrane (Fig. , open squares). Thus, we propose Anx4 associates with the lipid membrane through the coordinated calcium ions on CBSs I and IV (Fig. (C)). Our proposal is biologically interesting and important for understanding the association of Anx4 with the lipid membrane in cytoplasmic fluids. In contrast, CBSs II and III would have little interaction with the lipid membrane in the cytoplasmic fluids. Anx4 binds via CBS III to surfactant protein A.Citation17,18) The free CBSs II and III might play an important role in the expression of the physiological function of Anx4. Calcium-bound Anx4 binds ATP, but this activity does not influence lipid binding.Citation31) In contrast, calcium influx in living cells yields a partition of membrane-bound Anx4 into mobile and immobile forms.Citation32) These forms may be the result of different binding mechanisms. In any case, calcium-induced membrane binding plays an important role in the expression of Anx4 function; however, the physiological function of Anx4 is unclear.
Our study provides the first evidence that Anx4 association with the lipid membrane is mediated by relatively strong and weak interactions. We suggest Anx4 associates with the lipid membrane via the calcium ion in CBSs I and IV in the cytoplasmic fluid.
Notes
Abbreviations: Anx4, annexin A4; CBS, Ca2+-binding site.
References
- Raynal P, Pollard HB. Annexins: the problem of assessing the biological role for a gene family of multifunctional calcium- and phospholipid-binding proteins. Biochim. Biophys. Acta. 1994;1197:63–93.10.1016/0304-4157(94)90019-1
- Creuzt CE. The annexins and exocytosis. Science. 1992;258:924–931.
- Kaetzel MA, Dedman JR. Annexins: novel Ca2+-dependent regulators of membrane fusion. News Physiol. Sci. 1995;10:171–176.
- Gerke V, Moss SE. Annexins and membrane dynamics. Biochim. Biophys. Acta. 1997;1357:129–154.10.1016/S0167-4889(97)00038-4
- Huber R, Römisch J, Pâques EP. The crystal and molecular structure of human annexin V, an anticoagulant protein that binds to calcium and membranes. EMBO J. 1990;9:3867–3874.
- Liemann S, Huber R. Three-dimensional structure of annexins. Mol. Life Sci. 1997;53:516–521.10.1007/s000180050065
- Bandorowicz-Pikula J, Sikorski A, Bilkowska K, Sobota A. Interaction of annexins IV and VI with phosphatidylserine in the presence of Ca2+: monolayer and proteolytic study. Mol. Membr. Biol. 1996;13:241–250.
- Lizarbe MA, Barrasa JI, Olmo N, Gavilanes F, Turnay J. Annexin-phospholipid interactions. Functional implications. Int. J. Mol. Sci. 2013;14:2652–2683.10.3390/ijms14022652
- Massey D, Traverso V, Rigal A, Maroux S. Cellular and subcellular localization of annexin IV in rabbit intestinal epithelium, pancreas and liver. Biol. Cell. 1991;73:151–156.10.1016/0248-4900(91)90097-7
- Kojima K, Utsumi H, Ogawa H, Matsumoto I. Highly polarized expression of carbohydrate-binding protein p33/41 (annexin IV) on the apical plasma membrane of epithelial cells in renal proximal tubules. FEBS Lett. 1994;342:313–318.10.1016/0014-5793(94)80523-7
- Kaetzel MA, Chan HC, Dubinsky WP, Dedman JR, Nelson DJ. A role for annexin IV in epithelial cell function. Inhibition of calcium-activated chloride conductance. J. Biol. Chem. 1994;269:5297–5302.
- Fukuoka S-I, Kern H, Kazuki-Sugino R, Ikeda Y. Cloning and characterization of ZAP36, an annexin-like zymogen granule membrane associated protein, in exocrine pancreas. Biochim. Biophys. Acta. 2002;1575:148–152.10.1016/S0167-4781(02)00299-3
- Gerke V, Moss SE. Annexins: from structure to function. Physiol. Rev. 2002;82:331–371.
- Swairjo MA, Concha NO, Kaetzel MA, Dedman JR, Seaton BA. Ca2+-bridging mechanism and phosphplipid head group recognition in the membrane-binding protein annexin V. Nat. Struct. Biol. 1995;2:968–974.10.1038/nsb1195-968
- Kaetzel MA, Mo YD, Mealy TR, Campos B, Bergsma-Schutter W, Brisson A, Dedman JR, Seaton BA. Phosphorylation mutants elucidate the mechanism of annexin IV-mediated membrane aggregation. Biochemistry. 2001;40:4192–4199.10.1021/bi002507s
- Sohma H, Creutz CE, Gasa S, Ohkawa H, Akino T, Kuroki Y. Biochim. Differential lipid specificities of the repeated domains of annexin IV. Biochim. Biophys. Acta. 2001;1546:205–215.10.1016/S0167-4838(01)00140-6
- Sohma H, Matsushima N, Watanabe T, Hattori A, Kuroki Y, Akino T. Ca2+-dependent binding of annexin IV to surfactant protein A and lamellar bodies in alveolar type II cells. Biochem. J. 1995;312:175–181.
- Sohma H, Creutz CE, Saitoh M, Sano H, Kuroki Y, Voelker DR, Akino T. Characterization of the Ca2+-dependent biding of annexin IV to surfactant protein A. Biochem. J. 1999;341:203–209.10.1042/0264-6021:3410203
- Somarajan SR, Al-Asadi F, Ramasamy K, Pandranki L, Baseman JB, Kannan TR. Annexin A2 mediates Mycoplasma pesumoniae community-acquired respiratory distress syndrome toxin binding to eukaryotic cells. MBio. 2014;19:e01497–14.
- Butsushita K, Fukuoka S-I, Ida K, Arii Y. Crystal structures of sodium-bound annexin A4. Biosci. Biotechnol. Biochem. 2009;73:2274–2280.10.1271/bbb.90366
- Hu N-J, Bradshaw J, Lauter H, Buckingham J, Solito E, Hofmann A. Membrane-induced folding and structure of membrane-bound annexin A1 N-terminal peptides: implications for annexin-induced membrane aggregation. Biophys. J. 2008;94:1773–1781.10.1529/biophysj.107.119685
- Arii Y, Hirose M. Probing the serpin structural-transition mechanism in ovalbumin mutant R339T by proteolytic-cleavage kinetics of the reactive-centre loop. Biochem. J. 2002;363:403–409.10.1042/0264-6021:3630403
- Schneider CA, Rasband WS, Eliceiri KW. NIH Image to ImageJ: 25 years of image analysis. Nat. Methods. 2012;9:671–675.10.1038/nmeth.2089
- Voges D, Berendes R, Burger A, Demange P, Baumeister W, Huber R. Three-dimensional structure of membrane-bound annexin V. A correlative electron microscopy-X-ray crystallography study. J. Mol. Biol. 1994;238:199–213.10.1006/jmbi.1994.1281
- Sutton RB, Sprang SR. Three dimensional structure of annexin IV. In: Seaton BA, editor. Annexins: molecular structure to cellular function. Austin (TX): R.G. Lades Company; 1996. p. 31–42.
- Nelson MR, Creutz CE. Combinatorial mutagenesis of the four domains of annexin IV: effects on chromaffin granule binding and aggregating activities. Biochemistry. 1995;34:3121–3132.10.1021/bi00009a044
- Koch M, Diez J, Fritz G. Crystal structure of Ca2+-free S100A2 at 1.6-Å resolution. J. Mol. Biol. 2008;378:933–942.10.1016/j.jmb.2008.03.019
- Rosengarth A, Gerke V, Leucke H. A calcium-driven conformational switch of the N-terminal and core domains of annexin A1. J. Mol. Biol. 2003;326:1317–1325.10.1016/S0022-2836(03)00027-5
- Fukuoka S-I. Analysis of ZAPs, zymogen granule membrane associated proteins, in the regulated exocytosis of the pancreas. Biosci. Biotechnol. Biochem. 1994;58:1282–1285.10.1271/bbb.58.1282
- Tortora GJ, Derrickson B. Principles of anatomy and physiology: NJ: John Wiley & Sons; 2011. Chapter 27, Fluid, electrolyte, and acid-base homeostasis; p. 1110–1128.
- Bandorowicz-Pikula J, Wrzosek A, Makowski P, Pikula S. The relationship between the binding of ATP and calcium to annexin IV. Effect of nucleotide on the calcium-dependent interaction of annexin with phosphatidylserine. Mol. Membr. Biol. 1997;14:179–186.10.3109/09687689709048180
- Crosby KC, Postma M, Hink MA, Zeelenberg CH, Adjobo-Hermans MJ, Gadella TW. Quantitative analysis of self-association and mobility of annexin A4 at the plasma membrane. Biophys. J. 2013;104:1875–1885.10.1016/j.bpj.2013.02.057
- DeLano WL. The case for open-source software in drug discovery. Drug Discov. Today. 2005;10:213–217.10.1016/S1359-6446(04)03363-X