Abstract
We performed metabolic engineering on the budding yeast Saccharomyces cerevisiae for enhanced production of succinic acid. Aerobic succinic acid production in S. cerevisiae was achieved by disrupting the SDH1 and SDH2 genes, which encode the catalytic subunits of succinic acid dehydrogenase. Increased succinic acid production was achieved by eliminating the ethanol biosynthesis pathways. Metabolic profiling analysis revealed that succinic acid accumulated intracellularly following disruption of the SDH1 and SDH2 genes, which suggests that enhancing the export of intracellular succinic acid outside of cells increases succinic acid production in S. cerevisiae. The mae1 gene encoding the Schizosaccharomyces pombe malic acid transporter was introduced into S. cerevisiae, and as a result, succinic acid production was successfully improved. Metabolic profiling analysis is useful in producing chemicals for metabolic engineering of microorganisms.
Graphical Abstract
In this study, we examined succinic acid production by Saccharomyces cerevisiae and improved the productivity by metabolic engineering based on metabolic profiling analysis with CE-TOFMS.

The budding yeast Saccharomyces cerevisiae is utilized in the production of ethanol as fuel and alcoholic beverages. Since genome sequencing of it was completedCitation1) and genetic manipulation tools have been developed, S. cerevisiae has the potential as a host species for the production of fuel alcohols and chemical building blocks. Recently, the production of organic acids such as lactic acidCitation2–11) and malic acidCitation12–15) by S. cerevisiae has been examined because S. cerevisiae grows well under low pH conditions. Here, we report a metabolic engineering approach to succinic acid production by S. cerevisiae.
Succinic acid is regarded as one of the most useful chemical building blocks. It can be converted into useful chemicals, such as 1,4-butanediol and adipic acid.Citation16,17) The commercial market for succinic acid is 30,000–50,000 tons per year, expected to expand to 100,000 tons per year by 2015.Citation18) It has been reported that succinic acid production has been achieved using recombinant microorganisms, such as Escherichia coliCitation19–26) and Corynebacterium glutamicum.Citation27–29) Moreover, natural succinic acid-producing microorganisms have been isolated, including Actinobacillus succinogenesCitation30) and Mannheimia succiniciproducens.Citation31)
Usually, succinic acid is produced by microorganisms via the reductive tricarboxylic acid (TCA) cycle under microaerobic or anaerobic conditions, but other fermentation metabolites are also produced, including ethanol, lactic acid, acetic acid, and formic acid. In addition, CO2 assimilation through anaplerotic reactions to produce oxaloacetic acid, and the use of NADH as a reducing power for malate dehydrogenase are necessary steps in the production of succinic acid. Aerobic succinic acid production through the oxidative TCA cycle by microorganisms has also been developed. The theoretical maximum yield under aerobic conditions is lower than under anaerobic conditions: 67 and 133% (C-mol per C-mol glucose consumed) under aerobic and anaerobic conditions, respectively. However, under aerobic conditions, it is expected that the production of these fermentation metabolites as by-products can be reduced. Moreover, as reviewed by Raab et al. under anaerobic conditions, other reducing power besides NADH should be considered for succinic acid production.Citation32) In addition, as for S. cerevisiae, the activity of the reductive TCA cycle is low. The metabolism from oxaloacetic acid to succinic acid via malic acid and fumaric acid is not thermodynamically favorable, and the enzymes for this metabolism are subjected to glucose repression.Citation32) Hence, aerobic succinic acid production is more advantageous than anaerobic production in S. cerevisiae. It has been reported that aerobic succinic acid production was attempted by constructing S. cerevisiae recombinant strains in which genes related to the TCA cycle, such as SDH1, SDH2, SDH3, SDH4, and FUM1, were disrupted.Citation33,34) Recently, aerobic production of succinic acid through the oxidative TCA cycle and a glyoxylate shunt by a recombinant strain of S. cerevisiae, in which the IDH1 and IDP1 genes, encoding NAD+-dependent and NADP+-dependent mitochondrial isocitrate dehydrogenases, respectively, as well as the SDH1 and SDH2 genes were disrupted, was reported.Citation32,35)
For metabolic engineering to improve the productivity of the target product (i.e. improving productivity through genetic modifications, such as gene deletion, gene overexpression, and the introduction of heterologous genes related to the biosynthesis of target product into the host cells), it is better to do a comprehensive analysis of the cellular behavior of the host species.Citation36,37) At present, systems for such a comprehensive analysis by genomics, transcriptomics, proteomics, and metabolomics have advanced rapidly. Hence, metabolic engineering based on these analyses is regarded as important. Among these comprehensive analyses, metabolomics is effective in the attempt to understand cellular metabolic states for use in metabolic engineering.Citation38,39) In metabolomics, various chemical analysis systems have been utilized, including high performance liquid chromatography, gas chromatography, and capillary electrophoresis (CE), combined with mass spectrometry (MS), for the detection, quantification, and identification of the chemical structures of metabolites. Among these metabolomic analysis systems, CE–MS is used in the analysis of charged metabolites, such as sugar phosphates and organic acids.Citation40) Because most intracellular metabolites are charged, CE–MS can be used in metabolome analysis.
In this study, we examined succinic acid production by the S. cerevisiae SDH1- and SDH2-disrupted strains in shake-flask culture. Because the levels of ethanol produced by the constructed strain were high, the effect of eliminating the ethanol biosynthesis pathways on the production of succinic acid was also investigated. Moreover, in order to apply metabolomics to metabolic engineering to improve the productivity of succinic acid by succinic acid-producing S. cerevisiae, we examined the metabolic profiles of the recombinant strains by metabolomics. For metabolic profiling, the intracellular metabolite levels of the recombinant strains in the central carbon metabolism pathways, such as glycolysis, the TCA cycle, and the pentose phosphate pathway, were analyzed by CE-time-of-flight (TOF) MS. Based on the results of our metabolic profiling, we tried to improve the succinic acid yield from the recombinant S. cerevisiae strain through metabolic engineering, and improved it by expression of the gene encoding the Schizosaccharomyces pombe malic acid transporter.
Materials and methods
Strains and media
All the microbial strains used in this study are listed in Table . For the succinic acid production experiments, S. cerevisiae cells were grown in synthetic defined (SD) medium consisting of 6.7 g L−1 of yeast nitrogen base without amino acids (Difco Laboratories, Detroit, MI), 20 g L−1 (111 mM) of glucose, 20 mg L−1 of uracil, 30 mg L−1 of lysine hydrochloride, and 100 mg L−1 of leucine. SD agar plates were prepared by adding 20 g L−1 of agar to the SD medium. To transform S. cerevisiae to disrupt the SDH1 and SDH2 genes, as described below, cells were cultivated on a 2 × YPAD medium consisting of 20 g L−1 of Bacto yeast extract (Difco), 40 g L−1 of Bacto peptone (Difco), 40 g L−1 of glucose, and 100 mg L−1 of adenine hemisulfate.
Table 1. Microbial strains used in this study.
For the cultivation of E. coli, Lennox (L) medium consisting of 10 g L−1 of Bactotryptone (Difco), 5 g L−1 of Bacto yeast extract, 5 g L−1 of NaCl, and 1 g L−1 of glucose (pH 7) were used. When necessary, 50 mg L−1 of ampicillin was added to the L medium. L agar plates were prepared by adding 15 g L−1 of agar to that medium.
Disruption of the SDH1 and SDH2 genes in S. cerevisiae
The SDH1 and SDH2 genes were disrupted by the method reported by Güeldener et al.Citation41) The DNA fragments for the selection marker cassettes with homologous sequences upstream and downstream of a given target gene were amplified by polymerase chain reaction (PCR) with KOD-Plus- DNA polymerase (Toyobo, Osaka, Japan) and the following sets of primers: 5′-AGAAAAAAATCCAATTTCATAGTACGAAGAAGAACGAGAATAAAGAGCTGAAGCTTCGTACGC-3′ and 5′-AGAAGAGTATGATATTCTTTTCCGTAAAATACAATGAGGTTCAAACATAGGCCACTAGTGGATCTG-3′ for SDH1, and 5′-TGAGCTATACTTTCTTGAAATACTGGAGTATACATATTTATAGGGAGCTGAAGCTTCGTACGC-3′ and 5′-TGAGAGGCTTCTGAGTTCTTCGGGGCTAGCTGTTTTTCTGATAGTCATAGGCCACTAGTGGATCTG-3′ for SDH2. Plasmids pUG72 carrying the loxP-KlURA3-loxP cassette and pUG73 carrying the loxP-KlLEU2-loxP cassette (Euroscarf, Frankfurt, Germany) were used as templates in amplifying the PCR fragments to disrupt the SDH1 and the SDH2 gene, respectively. The amplified fragments were introduced into the BY4739 and S149 strains by the lithium acetate method as reported by Gietz and Woods.Citation42) Transformants were obtained by cultivation on SD agar plates removed uracil or leucine. Disruption of the target genes was confirmed by PCR with primers that annealed to the upstream and downstream regions of a given target gene: 5′-GTTATGGAGTACGGCTTTG-3′ and 5′-GACAGCACCCTTGTACAGCA-3′ to confirm SDH1 disruption and 5′-CGTATTATTGTAGCTCCGCACG-3′ and 5′-TGGACATATATACAGACCGG-3′ to confirm SDH2 disruption. The difference between the length of the fragment amplified from the wild-type genome DNA and that of the one amplified from the disruptant genome DNA was analyzed by agarose gel electrophoresis (data not shown). The BY4739 and S149 strains, carrying the SDH1::KlURA3 and SDH2::KlLEU2 disruptions, were named BY4739sdh12 and S149sdh12, respectively.
Succinic acid production by S. cerevisiae
Precultures were prepared by inoculating a single colony grown on an SD agar plate into 5 mL of SD medium in a test tube and culturing it at 30 °C at 150 strokes per min for 1 d. For the main culture, the preculture was transferred to 100 mL of SD medium in a 500-mL Sakaguchi flask to achieve an OD600 of 0.1, followed by culturing at 30 °C with reciprocal shaking at 150 strokes per min.
Measurement of cell growth and the concentrations of glucose, ethanol, and organic acids
Cell growth was monitored by measuring the optical density of the culture at 600 nm (OD600) with spectrophotometer UVmini-1240 (Shimadzu Corporation, Kyoto, Japan). The glucose concentration in the culture supernatant was measured by enzymatic biosensor BF-5i (Oji Scientific Instruments, Hyogo, Japan). The ethanol and organic acid concentrations in the culture supernatant were measured by gas chromatography and high performance liquid chromatography, respectively, by the methods reported by Usui et al.Citation43)
Metabolic profiling by CE-TOFMS
Metabolic profiling of the succinic acid-producing strains of S. cerevisiae was done by CE-TOFMS.
During the succinic acid production experiments, when OD600 reached approximately 1, an appropriate volume of culture, calculated from the 10/OD600 (mL) (corresponding to approximately 2.2 mg of dry cells), was taken from the culture. All procedures in the pretreatment of the samples were carried out by the method described by Yoshikawa et al.Citation44)
Anionic metabolites were separated in CE with a fused silica capillary (50 μm i.d. × 80 cm length) and an anion buffer solution (Human Metabolome Technologies, Yamagata, Japan). For TOFMS, electron spray ionization–mass spectrometry was done in negative ion mode. The analytical conditions and parameters have been described elsewhere.Citation44) CE-TOFMS analysis was done by an Agilent 7100 CE system equipped with an Agilent 6224 TOF-MS system, an Agilent 1200 isocratic HPLC pump, a G1603A Agilent CE/MS adapter kit, and a G1607A Agilent CE/MS sprayer kit (Agilent Technologies, Santa Clara, CA). For system control and data acquisition, Chemstation software for CE-MS (Agilent Technologies) and the MassHunter software (Agilent) were used. Peak area data were obtained by MassHunter software for qualitative analysis (Agilent). Finally, the molar content of the metabolite per g of dry cells was calculated from the metabolite concentration in methanol solution and the dry cell weight. To evaluate the difference in the levels of each metabolite, as between the two strains, a two-sided t-test was performed at a given a level of significance of 0.05.
Construction of the S. cerevisiae recombinant strain carrying the S. pombe mae1 gene
Malic acid transporter gene mae1from S. pombe was amplified by PCR from S. pombe genome DNA clone FYG16_m23,Citation45) with primers 5′-GTCGACCCCCTTTATCTCTCGATTCGACATG-3′ and 5′-GCGGCCGCAATTAAGGAAAAGCATACAAGCTTA-3′. The amplified fragment was cloned onto the pGEM-T easy plasmid (Promega, Fitchburg, WI), followed by confirmation of the sequence of the cloned DNA by the dideoxy method. The cloned DNA fragment was excised by digestion with SalI and NotI and then recloned into the XhoI-NotI sites of S. cerevisiae expression vector pNV11.Citation46) The resulting plasmid, named pNV11-mae1, was introduced into S. cerevisiae by the lithium acetate method as described above.
Results and discussion
Succinic acid production in SDH1- and SDH2-disrupted S. cerevisiae recombinant strains
As reported previously, disruption of the SDH1 and SDH2 genes enhances aerobic succinic acid production in S. cerevisiae.Citation35) Following that report, we constructed the BY4739sdh12 strain, in which the SDH1 and SDH2 genes were disrupted in wild-type strain BY4739, and examined the production of succinic acid and by-products such as ethanol, glycerol, and fumaric acid in the constructed strain (Fig. and Table ). As shown in Fig. (D)–(F), the by-products were consumed and cell concentration increased after glucose depletion in BY4739 (48–120 h). However, the consumption levels of the by-products after glucose depletion were significantly lowered by disruption of the SDH1 and SDH2 genes consistently with results reported previously.Citation34,47) Therefore, the yields of the target product (succinic acid) and by-products (ethanol, glycerol, and fumaric acid) at the times when almost all input glucose was consumed or glucose consumption stopped were examined to evaluate productivity.
Fig. 1. Succinic acid production in the SDH1- and SDH2-disrupted S. cerevisiae strains.
Note: (A) Cell growth, (B) glucose concentration, (C) succinic acid concentration, (D) ethanol concentration, (E) glycerol concentration, and (F) fumaric acid concentration in the BY4739 (hollow circles) and BY4739sdh12 (solid circles) strains are shown. Average ± standard deviation for three independent culture experiments is shown.
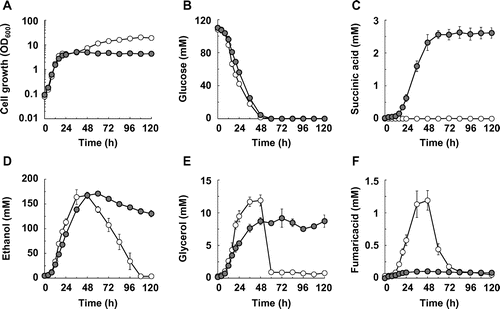
Table 2. Production yields in succinic acid-producing S. cerevisiae recombinant strains.
As shown in Fig. (A), the cell growth of the BY4739 and BY4739sdh12 strains reached stationary phase by 24 h, and most of the glucose input was consumed by 48 h. The specific glucose consumption rate during exponential growth (0–16 h) in BY4739sdh12 was lower than that of BY4739 (Supplemental Table 1; see doi:10.1080/09168451.2014.877816). It is known that Sdh1p and Sdh2p are components of complex II in the respiratory chain. Although Robinson et al. reported that complex II is not required for oxidative phosphorylation,Citation48) transcriptome analysis revealed that disruption of the SDH3 gene encoding a subunit of succinic acid dehydrogenase, which results in impairment of complex II in the respiratory chain, causes downregulation of genes related to central carbon metabolism.Citation49) Thus, the disruption of the SDH1 and SDH2 genes might affect the oxidative phosphorylation reactions and as a result, the specific glucose consumption rate was decreased by disruption of the SDH1 and SDH2 genes at the exponential phase. However, the specific glucose consumption rate at the stationary phase (24–48 h) in the BY4739sdh12 strain was higher than that in the BY4739 strain (Supplemental Table 2). The reason for this discrepancy remains unclear.
We calculated the yield of succinic acid and of its by-products at 48 h, when most of the input glucose had been consumed. As expected, increased succinic acid production was caused by disrupting the SDH1 and SDH2 genes (Fig. (C)), and about 1.5% of the carbons derived from glucose were converted to succinic acid (Table ). The yield of fumaric acid, which is produced from succinic acid by the reaction of succinic acid dehydrogenase in the TCA cycle, was decreased by disruption of the SDH1 and SDH2 genes (Table ), but approximately 50% of the carbons derived from glucose were converted to ethanol as a by-product in both the BY4739 and BY4739sdh12 strain (Table ).
Increased succinic acid production in SDH1- and SDH2-disrupted S. cerevisiae by disruption of the ADH1–5 genes
It is known that ethanol is a by-product of the production of useful chemicals by S. cerevisiae. We have reported that disruption of genes encoding NADH-dependent alcohol dehydrogenases, which convert acetaldehyde to ethanol, is important for elimination of the ethanol biosynthesis pathways.Citation50,51) Hence, we examined the effect of disrupting the ADH1–5 genes on the production of succinic acid by S. cerevisiae in which the SDH1 and SDH2 genes were disrupted (Fig. and Table ). The SDH1 and SDH2 genes were disrupted in ADH1–5-disrupted strain S149Citation50) and the resulting strain was named S149sdh12.
Fig. 2. Succinic acid production in ADH1–5-disrupted strain S149 and its SDH1- and SDH2-disrupted strain S149sdh12.
Note: (A) Cell growth, (B) glucose concentration, (C) succinic acid concentration, (D) ethanol concentration, (E) glycerol concentration, and (F) fumaric acid concentration in the S149 (hollow triangles) and S149sdh12 (solid triangles) strains are shown. Average ± standard deviation for three independent culture experiments is shown.
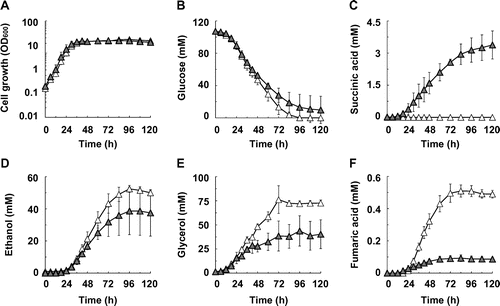
As shown in Fig. (A), the cell growth of the S149 and S149sdh12 strains reached stationary phase by 36 h. The specific glucose consumption rates during exponential growth (0–30 h) and the stationary phases (36–72 h) were not significantly different as between the S149 and S149sdh12 strains (Supplemental Tables 1 and 2). Most input glucose was consumed by 84 h in the S149 strain, while glucose consumption stopped at 84 h in the S149sdh12 strain. Hence, the productivity of metabolites was examined at 72 h (Table ). As expected, the ethanol yield was decreased by disruption of the ADH1–5 genes (Table ). The succinic acid production yield in the S149sdh12 strain was higher than in the BY4739sdh12 strain (Table ). Similarly to the BY4739 and BY4739sdh12 strains, fumaric acid production yield was lower in the S149sdh12 strain than in the S149 strain (Table ). These results indicate that disruption of the ADH1–5 genes is effective for improving succinic acid production from SDH1- and SDH2-disrupted S. cerevisiae. The succinic acid yield was approximately 20-fold higher when the SDH1 and SDH2 deletions were combined with the ADH1–5 deletions, but glycerol production was also increased by disruption of the ADH1–5 genes. In S. cerevisiae, ethanol biosynthesis reactions contribute mainly to the oxidization of NADH produced through glycolysis to maintain the intracellular balance of NADH and NAD+.Citation52) Moreover, when the ethanol biosynthesis pathways are disrupted, glycerol biosynthesis is induced to oxidize NADH produced through glycolysis.Citation53,54) Hence, in our experiments, glycerol production was increased in ADH1–5-disrupted strains S149 and S149sdh12.
Metabolic profiling of succinic acid-producing S. cerevisiae by the use of CE-TOFMS
Metabolomics is a powerful tool for the investigation of cellular metabolic profiles. In this study, we did metabolic profiling of the succinic acid-producing S. cerevisiae strain by metabolomics to improve succinic acid production further by metabolic engineering. We compared the metabolite profiles of the S149sdh12 strain with those from parent strain S149 during the exponential growth phase. We focused on the metabolites from central carbon metabolism, such as glycolysis, the pentose phosphate pathway, and the TCA cycle.
As shown in Fig. , the intracellular levels of the measured metabolites belonging to the glycolysis and pentose phosphate pathway in the S149sdh12 strain were similar to those in the S149 strain. As for the metabolites in the TCA cycle, the intracellular levels of succinic acid were significantly higher in the S149sdh12 strain than in the S149 strain, while the levels of fumaric acid and malic acid in the S149sdh12 strain were significantly lower in the S149sdh12 strain than in the S149 strain. These differences in metabolite levels were statistically significant (p < 0.05 by a t-test). Similar phenomena were observed when we compared the metabolic profiles of the BY4739 and BY4739sdh12 strains (data not shown). These results suggest that the S149sdh12 strain accumulates succinic acid intracellularly, and that the enhanced export of succinic acid to the outside of the cells is one of the strategies for enhancing succinic acid production by the S149sdh12 strain through metabolic engineering.
Fig. 3. Metabolic profiles of the S149 and S149sdh12 strains as analyzed by CE-TOFMS.
Note: The intracellular metabolite level (Y-axis, μmol g of dry cell−1) in the S149 (left, white bar) and S149sdh12 (right, gray bar) strains is shown. Average ± standard deviation for three independent culture experiments is shown. Asterisks indicate that the intracellular content was significantly different between the S149 and S149sdh12 strains. Glc, glucose; G6P, glucose-6-phosphate; F6P, fructose-6-phosphate; FBP, fructose-1,6-bisphosphate; DHAP, dihydroxyacetone phosphate; GAP, glyceraldehyde-3-phosphate; 1,3-BPG, 1,3-bisphosphoglycerate; 3PG, 3-phosphoglycerate; 2PG, 2-phosphoglycerate; PEP, phosphoenolpyruvate; Pyr, pyruvate; AcCoA, acetyl-CoA; AcAld, acetoaldehyde, EtOH, ethanol; PGL, phospho-glucono-1,5-lactone; 6PG, 6-phosphoglycerate; Ru5P, ribulose-5-phosphate; Xu5P, xylulose-5-phosphate; R5P, ribose-5-phosphate; S7P, sedoheptulose-7-phosphate; E4P, erythrose-4-phosphate; Cit, citrate; cis-Aco, cis-aconitate; IsoCit, isocitrate; 2OG, 2-oxoglutarate; SucCoA, succinyl-CoA; Suc, succinate; Fum, fumarate; Mal, malate; OAA, oxaloacetate.
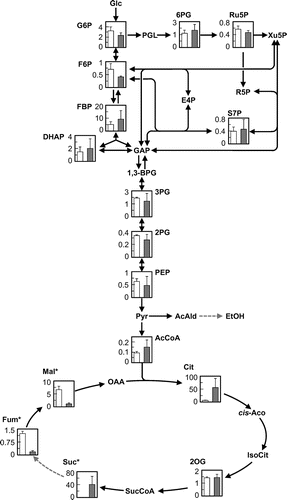
Metabolic engineering for the enhancement of succinic acid production by S. cerevisiae based on metabolic profiling data
Based on the metabolic profiling data, the S149sdh12 strain accumulated succinic acid intracellularly. Hence, enhancing the export of succinic acid to the outside of the cell should improve succinic acid production in the S149sdh12 strain, but the genes encoding the succinic acid transporters were not be found in the S. cerevisiae genome. It has been reported that introduction of the malic acid transporter gene mae1from S. pombe into S. cerevisiae increased extracellular succinic acid levels.Citation55) Following this report, we introduced the mae1 gene from S. pombe into the S149sdh12 strain and examined succinic acid production in the newly constructed strain (Fig. and Table ).
Fig. 4. Improvement of succinic acid production in the S149sdh12 strain by expression of the S. pombe mae1 gene.
Note: (A) Cell growth, (B) glucose concentration, (C) succinic acid concentration in the S149sdh12/pNV11 (hollow squares) and S149sdh12/pNV11-mae1 (solid squares) strains are shown. Intracellular succinic acid levels in the S149sdh12/pNV11 (white bar) and S149sdh12/pNV11-mae1 (gray bar) strains are shown (D). Average ± standard deviation for three independent culture experiments is shown.
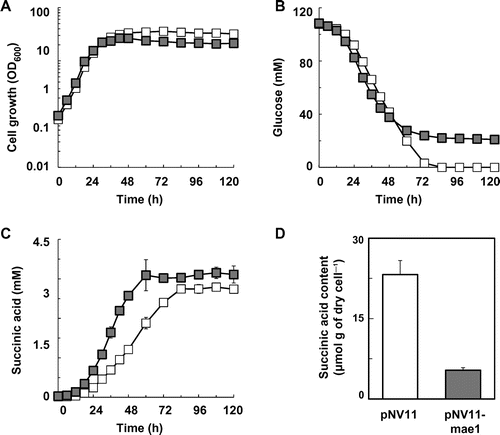
Table 3. Production yields in succinic acid-producing S. cerevisiae strains expressing the S. pombe mae1 gene.
The mae1 gene was cloned onto S. cerevisiae expression vector pNV11, and the resulting plasmid, pNV11-mae1, was introduced into the S149sdh12 strain. In the recombinant strain, the cloned mae1 gene was expressed from the TDH3 promoter on pNV11. Similarly to the results for the S149 and S149sdh12 strains, most input glucose was consumed by 84 h in the S149sdh12/pNV11 strain, while glucose consumption stopped at 84 h in S149sdh12/pNV11-mae1 (Fig. (B)). Hence, we examined the productivity of metabolites at 72 h (Table ). As shown in Fig. (C) and Table , the introduction and expression of the mae1 gene in the S149sdh12 strain enhanced succinic acid production levels and the yield. In addition, the specific succinic acid production rate at the exponential growth phase was also increased by the introduction of the mae1 gene (Supplemental Table 1). Reduction of the intracellular level of succinic acid by the introduction of the mae1 gene was confirmed by CE-TOFMS analysis (Fig. (D)).
Although the levels of ethanol and glycerol in S149sdh12/pNV11 at 72 h were higher than those in S149sdh12/pNV11-mae1 (Supplemental Fig. 1), the ethanol and glycerol production yields did not change due to the introduction of the mae1 gene (Table ). Fumaric acid production levels and yield of it at 72 h were also increased by the introduction of S. pombe mae1 gene, as shown in Table and Supplemental Fig. 1. The reason might be that the mae1 gene product exports fumaric acid as well as malic acid and succinic acid in S. cerevisiae.Citation12)
Our results indicate that enhancing the export of succinic acid improves succinic acid production yields by S. cerevisiae. By combining deletion of the SDH1, SDH2, and ADH1–5 genes and the introduction of the S. pombe mae1 gene, the yield of succinic acid was increased.
In this study, we performed metabolic engineering on S. cerevisiae to increase succinic acid production based on metabolic profiling analysis. Our metabolic profiling revealed that intracellular succinic acid levels in SDH1- and SDH2-disrupted S. cerevisiae strains S149sdh12 and BY4739sdh12 were higher than those in the S149 and BY4739 parent strains. Hence, we examined the effect of expression of the S. pombe mae1 gene encoding the malic acid transporter on the export of succinic acid in these strains. In the event, the production of succinic acid was improved. Metabolic profiling can be a powerful tool to produce useful chemicals in metabolic engineering. This is the first report on the application of metabolic profiling analysis to improve succinic acid production by recombinant S. cerevisiae strains.
Raab et al. reported that a succinic acid yield by S. cerevisiae was achieved at 7.3% (C-mol per C-mol glucose consumed) by disrupting the SDH1, SDH2, IDH1, and IDP1 genes.Citation35) In the present study, the succinic acid production at a yield of 2.4% (C-mol per C-mol glucose consumed) was achieved by disrupting the SDH1, SDH2, and ADH1–5 genes and overexpressing the S. pombe mae1 gene (Table ). Although we improved succinic acid production based on the results of metabolic profiling, the production yield in our experiments was about 1/3-fold lower than that reported by Raab et al.Citation35) However, addition of glutamate is necessary for cell growth in the strain disrupted SDH1, SDH2, IDH1, and our strain should prove superior when the carbon yield is improved. In addition, our result was far from the yield of succinic acid produced by various microorganisms.Citation56) For example, succinic acid yields in the metabolically engineered E. coli, C. glutamicum, A. succinogenes, and M. succiniciproducens were achieved at more than 50% (C-mol per C-mol glucose consumed). Therefore, further improvement of succinic acid production by S. cerevisiae is required. For example, utilization of a recombinant strain eliminating both ethanol and glycerol biosynthesis pathways, which we reported previously,Citation51) as host strain for succinic acid production might prove effective because the yields of ethanol and glycerol were nevertheless high (Table ), due to the presence of the SFA1 gene encoding NADH-dependent alcohol dehydrogenase and the ADH6 and ADH7 genes encoding NADPH-dependent alcohol dehydrogenases in the genome of the S149sdh12 strain. However, as we reported previously, since glucose consumption rate of this recombinant strain is very low,Citation51) the establishment of stable culture system is required. Therefore, improvement of the performance of this recombinant strain is required first. Then we will attempt succinic acid production by S. cerevisiae strain as host. Further study of the possibility of metabolic engineering of S. cerevisiae will be done to improve of succinic acid production.
Supplemental material
The supplemental material for this paper is available at http://dx.doi.org/10.1080/09168451.2014.877816
Supplemental Fig. 1 and Supplemental Tables 1 and 2
Download PDF (94.4 KB)Acknowledgments
The genome DNA clone for S. pombe FYG16_m23 and S. cerevisiae expression vector pNV11 was provided by the National Bio-Resource Project (NBRP) of the Ministry of Education, Culture, Sports, Science and Technology of Japan. We thank Ms Yuki Mori and Mr Tatsuya Itoga for CE-TOFMS analysis.
Funding
This work was supported financially by a Grant-in-Aid for Scientific Research (A) (No. 24246134, to S.H.) from the Japan Society for the Promotion of Science.
References
- Goffeau A, Barrell BG, Bussey H, Davis RW, Dujon B, Feldmann H, Galibert F, Hoheisel JD, Jacq C, Johnston M, Louis EJ, Mewes HW, Murakami Y, Philippsen P, Tettelin H, Oliver SG. Science. 1996;274:563–567.
- Hirasawa T, Ookubo A, Yoshikawa K, Nagahisa K, Furusawa C, Sawai H, Shimizu H. Appl. Microbiol. Biotechnol. 2009;84:1149–1159.
- Ishida N, Saitoh S, Ohnishi T, Tokuhiro K, Nagamori E, Kitamoto K, Takahashi H. Appl. Biochem. Biotechnol. 2006;131:795–807.
- Ishida N, Saitoh S, Onishi T, Tokuhiro K, Nagamori E, Kitamoto K, Takahashi H. Biosci. Biotechnol. Biochem. 2006;70:1148–1153.
- Ookubo A, Hirasawa T, Yoshikawa K, Nagahisa K, Furusawa C, Shimizu H. Biosci. Biotechnol. Biochem. 2008;72:3063–3066.
- Ishida N, Suzuki T, Tokuhiro K, Nagamori E, Onishi T, Saitoh S, Kitamoto K, Takahashi H. J. Biosci. Bioeng. 2006;101:172–177.
- Adachi E, Torigoe M, Sugiyama M, Nikawa J-I, Shimizu K. J. Ferment. Bioeng. 1998;86:284–289.
- Pacheco A, Talaia G, Sá-Pessoa J, Bessa D, Gonçalves MJ, Moreira R, Paiva S, Casal M, Queirós O. FEMS Yeast Res. 2012;12:375–381.
- Porro D, Brambilla L, Ranzi BM, Martegani E, Alberghina L. Biotechnol. Progr. 1995;11:294–298.
- van Maris AJ, Winkler AA, Porro D, van Dijken JP, Pronk JT. Appl. Environ. Microbiol. 2004;70:2898–2905.
- Tokuhiro K, Ishida N, Nagamori E, Saitoh S, Onishi T, Kondo A, Takahashi H. Appl. Microbiol. Biotechnol. 2009;82:883–890.
- Zelle RM, de Hulster E, van Winden WA, de Waard P, Dijkema C, Winkler AA, Geertman JM, van Dijken JP, Pronk JT, van Maris AJ. Appl. Environ. Microbiol. 2008;74:2766–2777.
- Wang X, Gong CS, T. Tsao GT. Appl. Biochem. Biotechnol. 1998;70–72:845–852.
- Oliveira EA, Costa AA, Figueiredo ZM, Carvalho Júnior LB. Appl. Biochem. Biotechnol. 1994;47:65–72.
- Figueiredo ZM, Carvalho Júnior LB. Appl. Biochem. Biotechnol. 1991;30:217–224.
- Cukalovic A, Stevens CV. Biofuels, Bioprod. Biorefin. 2008;2:505–529.
- Delhomme C, Weuster-Botz D, Kühn FE. Green Chem. 2009;11:13–26.
- Cheng K-K, Zhao X-B, Zeng J, Zhang J-A. Biofuels, Bioprod. Biorefin. 2012;6:302–318.
- Blankschien MD, Clomburg JM, Gonzalez R. Metab. Eng. 2010;12:409–419.
- Lee SJ, Lee DY, Kim TY, Kim BH, Lee J, Lee SY. Appl. Environ. Microbiol. 2005;71:7880–7887.
- Lin H, Bennett GN, San KY. Biotechnol. Bioeng. 2005;89:148–156.
- Ma JF, Jiang M, Chen KQ, Xu B, Liu SW, Wei P, Ying HJ, Chang HN, Ouyang PK. Bioprocess. Biosyst. Eng. 2011;34:411–418.
- Singh A, Cher Soh K, Hatzimanikatis V, Gill RT. Metab. Eng. 2011;13:76–81.
- Wang J, Zhu J, Bennett GN, San KY. Metab. Eng. 2011;13:328–335.
- Zhang X, Jantama K, Moore JC, Jarboe LR, Shanmugam KT, Ingram LO. Proc. Nat. Acad. Sci. 2009;106:20180–20185.
- Zhang X, Shanmugam KT, Ingram LO. Appl. Environ. Microbiol. 2010;76:2397–2401.
- Litsanov B, Brocker M, Bott M. Microb. Biotechnol. 2013;6:189–195.
- Litsanov B, Kabus A, Brocker M, Bott M. Microb. Biotechnol. 2012;5:116–128.
- Okino S, Noburyu R, Suda M, Jojima T, Inui M, Yukawa H. Appl. Microbiol. Biotechnol. 2008;81:459–464.
- Guettler MV, Rumler D, Jain MK. Int. J. Syst. Bacteriol. 1999;49:207–216.
- Lee PC, Lee SY, Hong SH, Chang HN. Appl. Microbiol. Biotechnol. 2002;58:663–668.
- Raab AM, Lang C. Bioeng. Bugs. 2011;2:120–123.
- Arikawa Y, Kuroyanagi T, Shimosaka M, Muratsubaki H, Enomoto K, Kodaira R, Okazaki M. J. Biosci. Bioeng. 1999;87:28–36.
- Kubo Y, Takagi H, Nakamori S. J. Biosci. Bioeng. 2000;90:619–624.
- Raab AM, Gebhardt G, Bolotina N, Weuster-Botz D, Lang C. Metab. Eng. 2010;12:518–525.
- Jang YS, Park JM, Choi S, Choi YJ, Seung do Y, Cho JH, Lee SY. Biotechnol. Adv. 2012;30:989–1000.
- Lee JW, Kim TY, Jang YS, Choi S, Lee SY. Trends Biotechnol. 2011;29:370–378.
- Ellis DI, Goodacre R. Curr. Opin. Biotechnol. 2012;23:22–28.
- Mapelli V, Olsson L, Nielsen J. Trends Biotechnol. 2008;26:490–497.
- Monton MR, Soga T. J. Chromatogr. A. 2007;1168:237–246.
- Gueldener U, Heinisch J, Koehler GJ, Voss D, Hegemann JH. Nucleic Acids Res. 2002;30:23e.
- Gietz RD, Woods RA. Methods Enzymol. 2002;350:87–96.
- Usui Y, Hirasawa T, Furusawa C, Shirai T, Yamamoto N, Mori H, Shimizu H. Microb. Cell Fact. 2012;11:87.
- Yoshikawa K, Hirasawa T, Ogawa K, Hidaka Y, Nakajima T, Furusawa C, Shimizu H. Biotechnol. J. 2013;8:571–580.
- Nakamura T, Nakamura-Kubo M, Hirata A, Shimoda C. Mol. Biol. Cell. 2001;12:3955–3972.
- Ninomiya-Tsuji J, Nomoto S, Yasuda H, Reed SI, Matsumoto K. Proc. Nat. Acad. Sci. 1991;88:9006–9010.
- Lemire BD, Oyedotun KS. Biochim. Biophys. Acta, Bioenerg. 2002;1553:102–116.
- Robinson KM, von Kieckebusch-Guck A, Lemire BD. J. Biol. Chem. 1991;266:21347–21350.
- Cimini D, Patil KR, Schiraldi C, Nielsen J. BMC Syst. Biol. 2009;3:17.
- Ida Y, Furusawa C, Hirasawa T, Shimizu H. J. Biosci. Bioeng. 2012;113:192–195.
- Ida Y, Hirasawa T, Furusawa C, Shimizu H. Appl. Microbiol. Biotechnol. 2013;97:4811–4819.
- Jouhten P, Rintala E, Huuskonen A, Tamminen A, Toivari M, Wiebe M, Ruohonen L, Penttilä M, Maaheimo H. BMC Syst. Biol. 2008;2:60.
- Cordier H, Mendes F, Vasconcelos I, François JM. Metab. Eng. 2007;9:364–378.
- Drewke C, Thielen J, Ciriacy M. J. Bacteriol. 1990;172:3909–3917.
- Camarasa C, Bidard F, Bony M, Barre P, Dequin S. Appl. Environ. Microbiol. 2001;67:4144–4151.
- Lee JW, Kim HU, Choi S, Yi J, Lee SY. Curr. Opin. Biotechnol. 2011;22:758–767.