Abstract
Plants produce a diverse array of secondary metabolites as chemical barriers against herbivores. Many phytophagous insects are highly adapted to these allelochemicals and use such unique substances as the specific host-finding cues, defensive substances of their own, and even as sex pheromones or their precursors by selectively sensing, incorporating, and/or processing these phytochemicals. Insects also serve as pollinators often effectively guided by specific floral fragrances. This review demonstrates the ecological significance of such plant secondary metabolites in the highly diverse interactions between insects and plants.
Graphical Abstract
Insects are highly adapted to plant secondary metabolites and use them as host-finding cues, defensive substances, and even as sex pheromones as in the giant butterfly, Idea leuconoe.

Insects are the most diverse group of animals on the earth, representing more than half of all known living organisms. While most of insects rely on plants’ primary metabolites (e.g. carbohydrates, lipids, and proteins), plants have evolved a high diversity of secondary metabolites (e.g. alkaloids, terpenoids, and phenolics) to cope with heavy herbivory. Insects are continuously challenged with counter mechanisms to detoxify or circumvent plants’ defense systems. On the other hand, insects and plants have coevolved mutualistic relationships through pollination. In such a complex ecological network, insects have developed highly sensitive and specific chemical sensors and biochemical processes to detect allelochemicals—to find host plants and nectar sources, to avoid noxious plants, and to escape from predators, in addition to their intraspecific recognition via pheromonal communications.
This review focuses on the chemistry and ecological significance of plant’s secondary metabolites involved in insect life histories and emphasizing particularly the following five subjects that are often closely linked to each other—partly summarizing my 45 years of research in insect chemical ecology.
I. Plant metabolites as host-finding cues
II. Plant metabolites as chemical barriers
III. Plant allelochemicals for defense
IV. Plant metabolites for sexual communication
V. Floral volatiles in mutualistic associations
I. Plant metabolites as host-finding cues
Most phytophagous insects feed on a limited number of plant species that often belong to a single plant family. The choice of host plants is determined both at the egg-laying and larval-feeding stages.Citation1) Insects’ host recognition involves multiple sensory modalities, including visual, olfactory, gustatory, and tactile cues. My attention has been focused on the phytochemical factors controlling egg-laying and larval-feeding behavior in swallowtail butterflies and sap-sucking behavior in aphids. These insects respond to specific chemical ingredients in the host plants.
1. Oviposition stimulants of swallowtail butterflies
“How can a citrus swallowtail butterfly sharply distinguish her host plants such as citrus and zanthoxylum-pepper trees, from many other neighboring plants?” was a question in my mind since childhood. The selection of an oviposition site by an adult female is crucial to the survival of her offspring, because the larvae are destined to eat only selected plant species. Thus, the mother butterflies must lay their eggs with great precision on the host plants. Females of swallowtails detect specific chemicals in the host plants by vigorously drumming upon the leaf surface with their forelegs. Toothbrush-like chemosensilla were found on the foretarsi of the female butterflies.Citation2) When a piece of filter paper impregnated with a host plant extract was brought in contact with the tarsal sensilla, she immediately deposited one or more eggs on the substrate.
The complex chemical profiles of oviposition stimulant systems have been revealed step by step in several papilionid species mainly by Keiichi Honda (Hiroshima Univ.), Paul Feeny’s group (Cornell Univ.), and our group (Nishida, Kyoto Univ.) providing a fairly systematic model for understanding the chemical basis and evolutionary process of host selection in phytophagous insects.
Rutaceae-feeders
Oviposition stimulants of a citrus swallowtail butterfly, Papilio xuthus, in Citrus unshiu leaves were found to consist of multiple components, which included flavonoids (hesperidin (1), narirutin (2), rutin (3), vicenin-2 (4)), a nucleoside (adenosine (5)), alkaloids ((−)-synephrine (6), 5-hydroxy-Nω-methyltryptamine (7), bufotenine (8)), a cyclitol ((+)-chiro-inositol (9)), and an amino acid derivative ((−)-stachydrine (10)) (Fig. ).Citation3–Citation5) None of the individual components elicited oviposition responses alone. The specific activity was provoked only when these compounds were applied as a mixture, indicating the importance of a synergistic effect of multiple components in host recognition. HondaCitation6) elucidated the oviposition stimulants of another citrus-feeding swallowtail, P. protenor, which also shares the same host, C. unshiu. Among the seven stimulant components, three components (1, 6, and 10) were shared by P. xuthus and P. protenor.Citation7) More than 70 swallowtail species in the tribe Papilionini are known to feed on the citrus family (Rutaceae). The oviposition stimulants of P. macilentus and P. bianor, which share a common rutaceous host, Orixa japonica, were characterized as cnidioside A (11) (P. macilentus, unpublished result) and a mixture of a hydroxylated γ-lactone (12), and a hydroxycinnamoyl ester (13) (P. bianor), respectively, demonstrating the distinct difference in the host-finding cues between these two sympatric species.Citation8,Citation9)
Fig. 1. Plant metabolites as host-finding cues in butterflies and aphids.
Note: Oviposition stimulants of P. xuthus: hesperidin (1), narirutin (2), rutin (3), vicenin-2 (4), adenosine (5), 5-hydroxy-Nω-methyltryptamine (6), bufotenine (7), (−)-synephrine (8), (+)-chiro-inositol (9), (−)-stachydrine (10); P. macilentus: cnidioside A (11); P. bianor: (−)-2C-methyl-D-erythrono-1,4-lactone (12), (−)-4-(E)-caffeoyl-L-threonic acid (13); P. polyxenes: luteolin 7-O-(6″-O-malonyl)-β-D-glucopyranoside (14), tyramine (15), chlorogenic acid (16); A. alcinous: aristolochic acid I (17), sequoyitol (18), 3-hydroxy-4-methoxycinnamoylmalic acid (19); B. philenor: 17 and pinitol (20); L. japonica: isorhamnetin 3-O-glucopyranosyl-(l→6)-galactopyranoside-7-O-glucopyranoside (21). Larval-feeding stimulant of P. xuthus: citrusin I (22), isosinensetin (23), 1,2-dilinolenoyl-3-galactopyranosyl-sn-glycerol (24). Probing stimulants in an aphid, M. crassicauda: quercetin 3-O-α-L-arabinopyranosyl-(1→6)-(2″-O-(E)-p-coumaroyl)-β-D-galactopyranoside (25).
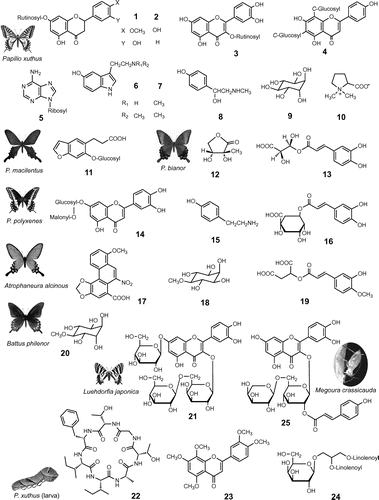
Apiaceae-feeders
Females of an Apiaceae-feeding common yellow swallowtail, P. machaon hippocrates, “mistakenly” lay eggs on plants belonging to Rutaceae family, such as Poncirus trifoliata and Zanthoxylum piperitum, common hosts of P. xuthus and P. protenor. The same reaction can be induced by stimulating a P. machaon female with extracts of these plants, although the response is much weaker than that induced by an extract from a carrot leaf, one of its major hosts, suggesting some common components involved as oviposition stimulants between Apiacea and Rutaceae.Citation2) The oviposition stimulants of another Apiaceae-feeder, P. polyxenes, were identified as a blend of a malonylated flavone glycoside (14), tyramine (15), and chlorogenic acid (16).Citation10,Citation11) The synergistic effects of combinations of flavonoids, hydroxycinnamates, and phenethylamine derivatives appeared to be a common feature of oviposition stimulant system among these two closely related butterfly species, even though the host-plant taxa (Apiaceae and Rutaceae) are remote to each other. Such an underlying phytochemcial similarity might have provided a route to colonization on novel hosts among the papilionid butterflies.Citation12,Citation13)
Aristolochiaceae-feeders
Most of the swallowtail butterflies in the tribe Troidini and Zerynthiini feed exclusively on the Aristolochiaceae. The oviposition stimulants of the troidine species Atrophaneura alcinous were isolated from the aerial parts of a pipevine, Aristolochia debilis, and identified as a mixture of aristolochic acids (AAs) (AA-I, 17) and sequoyitol (18).Citation14) AAs bear a nitro group on the phenanthrene ring, unique alkaloidal metabolites contained exclusively in the Aristolochiaceae, and thus would serve as an excellent cue. However, AAs exhibited very low activity alone. This represents an importance of synergism between 17 and 18. In addition, 3-hydroxy-4-methoxycinnamoylmalic acid (19), closely related to hydroxycinnamoyl esters (13 and 16) utilized as a chemical cue by several Rutaceae-feeders, was involved as an additional factor in A. alcinous (unpublished results). Further, pinitol (20), a hybrid compound between 9 and 18 was characterized as an oviposition stimulant for another troidine, Battus philenor, together with 17.Citation15) One of the oviposition stimulants of a primitive zerynthiine swallowtail, Luehdorfia japonica, was isolated from the leaves of Heterotropa aspera and identified as a new flavonol triglycoside (21).Citation16) The tribe Zerynthiini, to which L. japonica belongs, is considered to represent remnants of the stock from which the rest of the Papilionidae evolved.Citation17) Utilization of flavonoids as the oviposition stimulant components by both the most advanced genus, Papilio, and one of the most primitive genera, Luehdorfia, suggests the underlying conservative nature in host recognition within the family Papilionidae. Flavonoids seem certainly to provide a phytochemical diversity and specificity together with other stimulant ingredients as the host-finding cues for these phytophagous insects.
2. Larval phagostimulants of swallowtail butterflies
The cabbage white butterflies, Pieris rapae, and P. brassicae (Pieridae), recognize host crucifers by glucosinolates, both during oviposition and larval feeding.Citation18–Citation20) Likewise, larvae of an Aristolochiaceae-feeding swallowtail, A. alcinous, were found to be stimulated by the host-specific secondary metabolites 17 together with unknown cofactors.Citation14,Citation21) These facts suggested a congruent sensory mechanism between the tarsal chemoreceptors of adults and the gustatory chemoreceptors of larvae towards host-specific plant allelochemicals. However, the nature of such chemosensory processes at both larval and adult stages is not well understood.
Larval-feeding stimulants for a Rutaceae-feeding swallowtail, P. xuthus, were examined in C. unshiu leaves in comparison with the oviposition stimulants described above, using thin paper strips as a substrate. Stimulation of feeding on the extracts was found to require a mixture of chemicals including sugars (D-glucose, D-fructose, and D-sucrose), stachydrine (10), a cyclic peptide (citrusin I (22)), a polymethoxyflavone (isosinensetin (23)), and lipids (i.e. four 1-monoacylglycerols and a glycoglyceride (24)) (Fig. ).Citation22) The larvae readily consumed the test strips treated with a mixture of all the 11 compounds, while very few larvae consumed the strip when each compound was tested alone, indicating that host recognition by P. xuthus larvae is mediated by a specific combination of both primary and secondary metabolites. This result is in contrast to the 10 oviposition stimulant components of P. xuthus contained in the same host species (C. unshiu) in which only one compound, stachydrine (10), was an ingredient in common. While the larval-feeding stimulant mixture is dominated by nutrients and other compounds of general significance for primary metabolism, the component oviposition stimulants are exclusively secondary substances that have fairly restricted distributions in plants. Since the initial host choice for the larvae is made by the ovipositing female, unique secondary metabolites may be less important cues for larval feeding than are compounds useful for indicating food and microhabitat quality once settled on the host plant. Likewise, our recent study revealed that the larvae of a primitive Aristolochiaceae-feeding swallowtail butterfly, Sericinus montela (tribe Zerynthiini), were stimulated to feed by a mixture of secondary substances specific to the host A. debilis (17 and 18) plus a series of ubiquitous sugars (glucose, fructose, and sucrose) and a lipid (24). The complex blend of these primary compounds was identical to the key larval-feeding stimulants of P. xuthus (unpublished results). This result further substantiates the common nature of both primary and secondary plant metabolites serving as gustatory cues to which oligophagous lepidopteran larvae are tuned.
Initially, the complexities of host recognition and feeding stimulation were demonstrated in some lepidopteran larvae: some volatile essential oil components were found to act as olfactory cues in determining the choice of host plants prior to contact with the leaves by larvae of the Apiaceae-feeding swallowtail species, P. polyxenes.Citation23) In the silkworm moth, Bombyx mori (Bombycidae), larval-feeding behavior is controlled by three distinct factors: attractants (e.g. citral, linalool, 3-hexenol), biting factors (e.g. isoquercitrin, β-sitosterol), and swallowing factor (cellulose) in addition to cofactors (e.g. sucrose, myo-inositol, and inorganic phosphate)Citation24); a recent study has revealed an olfactory receptor protein responsible for a key mulberry leaf volatile, jasmone.Citation25) Elucidation of olfactory and gustatory chemical cues and their chemosensory mechanisms of host recognition both at egg laying and larval feeding will contribute to a greater understanding of the evolution of host selection in Lepidoptera.
3. Probing stimulants in aphids
Aphids (Aphididae) are mostly sap feeders that gregariously suck plant nutrients from the phloem of still-developing buds and leaves. Although many agricultural pest species are polyphagous, infesting a wide range of crops and often alternating seasonally between hosts, many other species are oligophagous within a limited number of host species belonging to a single family. Van EmdenCitation26) declared that “aphids are phytochemists,” i.e. their life histories are greatly influenced by plant chemistry. The host ranges of the Aphididae are far more diverse than those of butterflies and include ferns, gymnosperms, and angiosperms, yet aphids exhibit some similarities in host selectivity to each other among some oligophagous species. Thus, my studies on aphids were conducted in parallel to those on butterflies to clarify the chemical bases of host specificity among several aphid species.
The process of finding food sources in aphids involves the following sequential steps: (1) orientation to a plant, (2) external examination, (3) probing through the plant tissues, (4) tapping the phloem, and (5) ingestion.Citation27) Specific chemical cues seem to be associated within each step during the assessment of suitable hosts.
My research group has elucidated chemical factors that regulate steps (3)–(5) in an oligophagous bean aphid, Megoura crassicauda, which feeds selectively on plants in the genus Vicia (Fabaceae) and is known as a pest of broad bean, Vicia faba. Two specific probing stimulants were isolated from one of its favorite hosts, narrowleaf vetch, V. angustifolia, and were characterized as acylated flavonol diglycosides (25 and its glucosidic isomer).Citation28) These compounds strongly induced formation of a proteinous stylet sheath on a parafilm membrane when applied as a solution in distilled water, suggesting that they act as a factor in navigating the stylet towards the phloem in step (3). In contrast, their corresponding deacyl analogs, present abundantly in the host tissues, were suggested to serve as a negative stimulus to allow the aphid to refrain from sucking during tissue penetration before tapping the phloem, although the distribution of these analogs in the plant tissues remains unknown. Chemical factors in the host plant extract involved in the feeding steps (4) and (5), were represented by sucrose and amino acids, because the aphids positively imbibed an artificial diet composed of primary nutrients mimicking a phloem sap in addition to the probing stimulants and produced a large quantity of honeydews.Citation29) Thus, the feeding behavior of M. crassicauda was shown to be controlled by multiple chemical stimuli composed of primary and secondary plant metabolites mediating the process of the settlement on its hosts.
A dihydrochalcone, phlorizin, is known as a host-specific chemical cue for an apple aphid Aphis pomi.Citation30) We have also identified the probing stimulants of a cowpea aphid, Aphis craccivora (host broad bean, V. faba), rose aphid, Sitobion ibarae (host Japanese rose, Rosa multiflora), and an elder aphid, Acyrthosiphon magnoliae (winter host elder, Sambucus racemota) to be flavonol glycosides present in the corresponding hosts (unpublished results). These results provide additional examples of utilization of flavonoids as kairomonal cues by aphids.
II. Plant metabolites as chemical barriers
Besides insecticidal and other noxious plant allelochemicals, there is a diverse array of secondary metabolites to disrupt processes such as insect host-finding behavior and endocrinological systems. This section illustrates the ecological and physiological roles of some of these phytochemicals as potential chemical modulators of phytophagous insects’ activities.
1. Oviposition and feeding deterrents in butterflies
Although the citrus swallowtail, P. xuthus, feeds on various rutaceous species, both the female butterflies and larvae avoid a rutaceous plant, O. japonica, due to potent deterrent chemicals in the plant. The most abundant flavonoid triglycoside (26) was characterized as one of the major oviposition deterrents from the leaves (Fig. ).Citation31) This compound is a xylosyl derivative of rutin (3), a positive ovipositional stimulant for the butterfly (Fig. ), and may possibly disrupt the oviposition stimulant activity due to its structural resemblance, competing for the same chemoreceptor neurons on the foretarsi. Further, two hydroxybenzoic acid derivatives were characterized as potent deterrents of both oviposition and larval feeding in P. xuthus: a glycosylated hydroxybenzoic acid (27) and disyringoyl glucaric acid (28).Citation32) Simultaneous occurrence of these compounds in O. japonica appears to provide an effective chemical barrier against P. xuthus. This suggests a congruent chemosensory mechanism of allomonal chemicals acting on both female tarsal chemoreceptors and larval maxillary taste receptors, which can be contrasted to the case of oviposition stimulants/larval-feeding stimulants in P. xuthus as discussed above (I-1 and I-2).
Fig. 2. Plant metabolites as chemical barrier against insects.
Note: Oviposition and feeding deterrents of P. xuthus: quercetin 3-O-(2G-β-D-xylopyranosylrutinoside) (26), 5-{[2-O-(β-D-apiofuranosyl)-β-D-glucopyranosyl]oxy}-2-hydroxybenzoic acid (27) and disyringoyl glucaric acid (28). Probing deterrent in aphids, M. crassicauda: (E)-2-methyl-2-butene-1,4-diol 4-O-β-D-glucopyranoside (29). Insect growth regulators: juvenile hormone III (30), juvocimene II (31), 1-(3,4-methylenedioxyphenyl)-(E)-3-decene (juvadecene) (32).
![Fig. 2. Plant metabolites as chemical barrier against insects.Note: Oviposition and feeding deterrents of P. xuthus: quercetin 3-O-(2G-β-D-xylopyranosylrutinoside) (26), 5-{[2-O-(β-D-apiofuranosyl)-β-D-glucopyranosyl]oxy}-2-hydroxybenzoic acid (27) and disyringoyl glucaric acid (28). Probing deterrent in aphids, M. crassicauda: (E)-2-methyl-2-butene-1,4-diol 4-O-β-D-glucopyranoside (29). Insect growth regulators: juvenile hormone III (30), juvocimene II (31), 1-(3,4-methylenedioxyphenyl)-(E)-3-decene (juvadecene) (32).](/cms/asset/bc2ede77-b3d8-4597-a882-b3034d6179b6/tbbb_a_877836_f0002_b.gif)
2. Probing deterrent in aphids
As mentioned in I-3, the bean aphid, M. crassicauda, feeds selectively on plants of the genus Vicia. However, it never infests the tiny vetch, V. hirsuta, even though the plant often forms a mixed community with V. angustifolia, one of the most favorite hosts of the aphid. A specific probing deterrent was isolated from V. hirsuta by monitoring the inhibitory effect, and its structure was elucidated as a hemiterpene glucoside (29).Citation33) Because the stylet insertion process is a crucial step for the aphid’s settlement on a plant, the deterrent glycoside seems to act as an effective chemical barrier for V. hirsuta.
3. Insect growth regulators
Plants even manufacture exact insect hormone molecules, such as 20-hydroecdysone and juvenile hormone (JH) III (30) in massive quantities.Citation34,Citation35) During my collaborative investigations of hormonal substances with Professor William S. Bowers at Cornell University, two novel compounds, juvocimenes I and II (31), with an extraordinarily high JH activity were isolated from an essential oil of sweet basil, Ocimum basilicum (Labiatae).Citation36) Juvocimenes possess a fused structure between (E)-β-ocimene (monoterpene) and (E)-anethole (phenylpropanoid).Citation37) Juvocimene II showed about 3000 times as much activity as that of JH I in the milkweed bug, Oncopeltus fasciatus in a topical application. Similarly, a compound with a significant JH activity was isolated from the plant, Macropiper excelsum (Piperaceae), and was determined as 1-(3,4-methylenedioxyphenyl)-(E)-3-decene (32) (named juvadecene).Citation38)
It is of great interest if plants have developed specific biosynthetic pathways in response to intensive herbivory by modifying existing molecular units to make a novel arsenal as mentioned above: oviposition deterrent flavonoid triglycosides (26) with rutin (3) + xylose; juvocimenes (31) with monoterpene + phenylpropanoid; juvadecene (32) by extension of side chain of a phenylpropanoid commonly present in Piperaceae.
III. Sequestration of plant allelochemicals for defense
A number of phytophagous insects seem to have been selected to sequester plant allelochemicals in their body tissues and/or integuments.Citation39,Citation40) Thus, such insects can obtain a potent defense mechanism without manufacturing noxious chemicals of their own. Sequestered phytochemicals include highly toxic alkaloids, terpenoids, and phenolics, which normally function in plants to repel or poison herbivores. This section presents examples of a variety of sequestrates and their defensive modes of action against potential predators.
1. Recycling of larval host chemicals for defense
Pipevine swallowtails—aristolochic acids
A number of aposematic swallowtail species belonging to both tribe Troidini and Zerynthiini feed on the Aristolochiaceae and sequester a series of toxic AAs from their host plants for defense.Citation40–Citation42) Larvae of A. alcinous selectively incorporate AAs from their host plant, A. debilis, into their body tissues. A large titer was found in eversible defensive glands (osmeteria) as a concentrated solution in glycerol.Citation21) AAs act as a feeding deterrent for bird predators. AAs are present in pupal and adult tissues as well as in eggshells and yolk, which suggests there is an effective protection of every life stage from potential enemies by depending solely upon the host pipevine. Since AAs act as host-finding cues both by the adult female butterflies (I-1) and larvae and (I-2), an assessment of the host-plant quality both at the oviposition and larval-feeding stages could guarantee subsequent protection.Citation14) Ecological adaptation of A. alcinous thus seems to be strongly associated with sensory mechanisms specifically developed for perception of AAs.
Danaine butterflies—pyrrolizidine alkaloids
Most danaine butterflies acquire pyrrorizidine alkaloids (PAs) during their adult stage by foraging for non-host plants rich in PAs. However, a giant danaine butterfly, Idea leuconoe (Nymphalidae), in Okinawa feeds on Parsonsia laevigata (Apocynaceae) and sequesters a series of pyrrolizidine alkaloids including new macrocyclic alkaloids, ideamines B (33), and C as N-oxide forms, directly from its host plant (Fig. ).Citation43,Citation44) The alkaloids are considered to function as defensive substances against predators, and are used as pheromone precursor by males during courtship (see Section IV). Most other danaine species acquire PAs not from the hosts but as adults by foraging from PA-containing plants (see III-2). It has been suggested the host Parsonsia is one of the ancestral food plants of the Danainae, and the insects retained the ability to acquire PAs through pharmacophagy even after they shifted to other plants.Citation45) This primitive butterfly may provide a clue for understanding the evolution of pharmacophagous behavior in the subfamily Danainae.
Fig. 3. Defensive substances sequestered from plants.
Note: I. leuconoe: ideamine B (33); P. apollo and A. glossulariata: sarmentosin (34); A. gaschkevitchii arichannatoxin I (35); A. nipponicus: paederoside (36); A. rosae ruficornis: clerodendrin D (37); D. speciosa and C. arcuata: 23,24-dihydrocucurbitacin D (38).
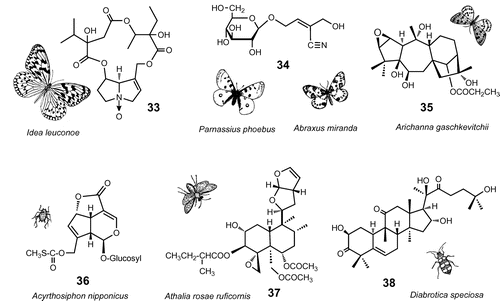
Apollo butterflies—cyanoglucoside
It was initially found that a number of day-flying Abraxas moth species (e.g. A. miranda, A. latifasciata, Abraxas glossulariata, etc. Geometridae) feeding on leaves of Euonymus spp. (Celastraceae) during the larval stage store a large quantity of a bitter-tasting cyanoglucoside, sarmentosin (34), in the adult body tissues from the larval hosts.Citation41,Citation46) Sarmentosin itself is not cyanogenic, but its epoxide spontaneously liberates HCN.Citation47) During discussion with Dr Miriam Rothschild, a possible involvement of sarmentosin with Apollo butterflies (Parnassius spp., Papilionidae) was suggested, because they feed on Sedum, which was reported to contain sarmentosin. As predicted, sarmentosin was detected in a substantial quantity in adults of Parnassius apollo in Europe and in P. phoebus in the Rocky Mountains in the USA.Citation42,Citation48) Thus, the secret of their aposematic life style both as larvae and adults can be explained by sermentosin, although any potential predators and the actual defensive roles of the compound remain to be clarified.
Ericaceae-feeding moth—grayanoid diterpenes
Grayanotoxins (GTs, grayanoids) are highly oxygenated diterpenes with 5- and 7-membered rings, unique in ericaceous plants. These compounds exhibit potent neurotoxicity in mammals by binding to specific sodium ion channels in cell membranes, and thus herbivorous animals avoid the plant.Citation49) However, larvae of the leopard moth, Arichanna gaschkevitchii (Geometridae), selectively eat with impunity the young leaves of an ericaceous plant, Pieris japonica, even though it contains high concentrations of GTs. Several GTs, including two new analogs, arichannatoxins I (35) and II, were identified from the body tissues of adult moths in a concentration as high as 300 μg/moth, which was sufficient to deter predatory house lizards.Citation41,Citation50) The adult moth exhibits an aposematic color pattern with orange-yellow hind wings and it flies during the day like a butterfly. A related species with a similar wing pattern, A. melanaria, oligophagous on several ericaceous plants often lacking GTs, sequesters much lower amounts of GTs when fed on P. japonica (less than 60 μg/moth). This represents a case of Müllerian mimicry in the same genus, sharing the same host, with different loads of the defense substances.Citation40)
Rubiaceae-feeding aphid—Iridoid glucoside
A ruby-red-colored aphid, Acyrthosiphon nipponicus (Aphididae), monophagously feeds on Paederia scandens (Rubiaceae). The aphid is seldom attacked by the ladybird beetle, Harmonia axyridis (Coccinellidae). A potent deterrent against the beetle was isolated from the aphid and identified as paederoside (36), an iridoid glycoside containing a sulfur atom in the molecule, unique in the aphid’s host, P. scandens.Citation51) The aphids secrete a mixture of 36 and lipids from the cornicles (aphids’ defensive glands), and quickly smear the fluid onto a predator’s mouthpart. Once an adult of H. axryridis bites into the aphid, it immediately releases the aphid, regurgitates, and quickly flees from the aphid colony. This exemplifies a typical case of usurpation of plant’s defensive substance by a phytophagous insect effectively to repel its major predator in a tritrophic interaction. The effect of the aposematic red color of the aphid against the ladybirds is not known. Kamo et al.Citation52) recently reported that the black-colored cowpea aphid, A. craccivora, that infests the black locust, Robinia pseudoacacia (Fabaceae), showed toxicity to H. axyridis due to cyanamide (NH2-CN), whereas the same aphid that infests the common vetch, V. angustifolia (free from the toxin), is suitable prey for H. axyridis larvae, further exemplifying this multitrophic adaptation mechanism in aphid–host plant interactions (cf. I-3).
2. Pharmacophagy—sequestration of non-host plant chemicals
“Plants are not only a ‘grocery store’ for feeding, but can also be a ‘pharmacy,’” this observation by BoppréCitation53) being the basis for his metaphorical term, “pharmacophagy.” If an animal acquires plant substances not for primary metabolism but for specific purpose other than nutrition or host recognition, it is defined as pharmacophagous. Adults of many danaine butterflies and some arctiid moths gather PAs pharmacophagously from specific plants rich in the alkaloids instead of acquiring them from their larval hosts (cf. I. leuconoe, III-1).Citation42) Several other pharmacophagous associations have been found in Diptera, Hymenoptera, and Coleoptera, as described below.Citation54)
Turnip sawfly—neo-clerodane diterpenoids
Adults of the turnip sawfly, Athalia rosae ruficornis (Tenthredinidae), visit a plant, Clerodendron trichotomum (Verbenaceae), and feed voraciously on glandular trichomes on the leaf surface. A series of bitter-tasting neo-clerodane diterpenoids (e.g. clerodendrins A, B, D (37) were characterized as the potent phagostimulants for the sawfly (Fig. ).Citation54,Citation55) The insect subsequently sequesters some of the analogs and likely becomes extremely bitter to predators on its body surface. The major sequestrate 37 deterred feeding by sparrows and tree lizards.Citation54,Citation56)
Leaf beetle—cucurbitacin triterpenes
Polyphagous leaf beetles, Diabrotica speciosa, and Cerotoma arcuata (Chrysomelidae) are destructive pests of various crops in Brazil. They are strongly arrested by root components from the cucurbit plant, Ceratosanthes hilariana (a non-host for larvae). Although the major phagostimulants were identified as cucurbitacins B and D, the adults selectively accumulated a relatively more stable minor component, 23,24-dihydrocucurbitacin D (38), gaining bitterness effective against a bird predator.Citation54,Citation57) Similarly, four Asian Cucurbitaceae-feeding specialists in the genus Aulacophora were found to sequester the same compound directly from the larval/adult host cucurbits.Citation58) The strong affinity to cucurbitacins, selective sequestration of the analogs and consequent protection from predators suggested an ecological adaptation mechanism developed in common among these two geographically isolated subtribes in the Luperini—both of the New and Old Worlds.Citation58)
Oriental fruit fly—methyl eugenol
The oriental fruit fly, Bactrocera dorsalis (Tephritidae) is a serious agricultural pest, infesting most succulent fruit species in the tropics and subtropics. Methyl eugenol (ME, 44) is known as an extremely potent attractant for B. dorsalis males (Fig. ), and the compound was successfully used as a mass-trapping agent in eradication programs such as in Okinawa.Citation59) The males attracted to the chemical source, voraciously feed on the intact chemical and sequester its metabolites, 2-allyl-4,5-dimethoxyphenol (45) and (E)-coniferyl alcohol (46), selectively in the rectal glands known to be a sex pheromone reservoir.Citation60) The total amount of the metabolites accumulated in the rectal glands was often as high as 100 μg/male, sufficient to deter feeding by predators, such as sparrows, lizards, and spiders.Citation54,Citation61) Because 44 is distributed widely in plants, males can acquire the compound pharmacophagously by foraging during adulthood.Citation62) Many host fruits of B. dorsalis contain 44 as a minor component. However, the larvae which fed on an artificial diet containing 44 did not carry over the compounds to the adults.Citation63) Pheromonal roles of ME-metabolites are discussed in Section IV and synomonal associations are discussed in Section V.
Fig. 4. Plant chemicals for sexual communication.
Note: Males of the giant danaine butterfly, I. leuconoe, biotransform defensive pyrrolizidine alkaloid, lycopsamine (39), to danaidone (40) and viridifloric β-1actone (41) and emit from the hairpencil organ as sex pheromone. A female oriental fruit moth, G. molesta, is attracted to male sex pheromone composed of methyl epijasmonate (42) and ethyl (E)-cinnamate (43). Males of oriental fruit fly, B. dorsalis, pharmacophagously acquire methyl eugenol (44) from plants and biotransform to sex pheromone 2-allyl-4,5-dimethoxyphenol (45) and (E)-coniferyl alcohol (46), which entice females during courtship. Males of the guava fruit fly, B. correcta, sequester β-caryophyllene (47) in the rectal pheromone glands. Mediterranean fruit fly, C. capitata, may use α-copaene (48) as a cue to navigate both sexes to the rendezvous site.
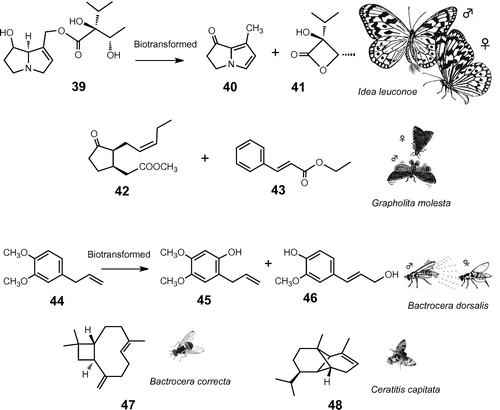
IV. Plant metabolites for sexual communication
Insects produce a diverse assemblage of sex pheromones to attract the opposite sex of the same species. These compounds are strictly species-specific with unique chemical structures and/or combinations of multiple components manufactured by specific biosynthetic pathways. However, in some instances, insects employ specific plant secondary metabolites to attract and excite the partners during courtship.
1. Plant-derived sex pheromones
Giant danaine butterfly—pyrrolizidine alkaloids
Males of the danaine butterfly, I. leuconoe, display ‘hairpencils,’ a pair of brush-like glandular organs, to cause the female to become quiescent during courtship. The hairpencil volatiles are composed of a complex mixture, including danaidone (40) and viridifloric β-1actone (41) (Fig. ).Citation64,Citation65) Virgin females were visually attracted to and olfactorily arrested by an artificial butterfly model scented with a blend of the two PA fragments 40 and 41. These two compounds appeared to be fragments of a pyrrolizidine alkaloid such as lycopsamine 39 originated from the host, P. laevigata, and sequestered in the body tissues as N-oxide forms for defense (see Section III-1). The female seems to verify a male’s ability to provide protection via the quality of pheromone volatiles emitted from hairpencils. This strong allelochemical linkage by this primitive butterfly suggests that a secondary colonization of danaine species to PA-free plants such as Asclepiadaceae might have taken place with retention of the PA-mediated allomonal and pheromonal systems via pharmacophagous acquisition of the precursors from non-host PA plants.Citation42)
Oriental fruit moth—herbal essence
Males of the oriental fruit moth, Grapholita molesta, emit a herbal scent, such as methyl epijasmonate (42) and ethyl (E)-cinnamate (43), from hairpencils to attract conspecific females after himself attracted to the female’s vicinity by the female pheromone (e.g. (Z)-8-dodecenyl acetate).Citation66) Ester 42, known as the key ingredients in high-grade perfumes originally extracted from jasmine flowers, is a plant hormone that regulates various physiological processes. These herbal essences are presumably derived from their larval host fruits, although the origin of these compounds remains to be clarified.Citation67)
Oriental fruit fly—phenylpropanoids
The strong attractiveness of B. dorsalis males to phenylpropanoid 44 (ME) is a unique characteristic to obtain a defensive measure as discussed above (see Section III). Males fed with 44 significantly had their performance during courtship enhanced, and they competed for virgin females significantly better than ME-deprived males.Citation68–Citation70) The pharmacophagously acquired ME-metabolites 45 and 46 were shown to arrest females, inducing their acceptance posture as illustrated in Fig. . Males of several other closely related Bactrocera species (B. carambolae, B. zonata, and B. correcta) fed on ME, selectively converted it into other unique subsets of ME-metabolites, including (Z)-coniferyl alcohol and (Z)-3,4-dimethoxy-cinnamyl alcohol, and stored these in the rectal gland.Citation71,Citation72) A series of sesquiterpene hydrocarbons such as β-caryophyllene (47) was identified from the rectal gland of wild B. correcta males.Citation73) These pharmacophagously acquired rectal sequestrates (both phenylpropanoids and sesquiterpenes) were suggested to function possibly as a unique sex pheromone to attract conspecific females of this highly diverged species radiating from central Southeast Asia. Compound 47 was found to be a more attractive male lure than 44 in B. correcta, and 47 thus serves as a highly selective monitoring agent not attractive to other sympatric pest species in Thailand (unpublished results).
In these instances, the male pheromone systems are suggested to have advanced within the context of sexual selection—the female’s preference for the specific components linked to fitness-related defensive compounds. These plant-originated male pheromones may have evolved possibly through a sensory exploitation process to appeal to the female’s chemoreceptors, and as a consequence females recognize males that are coincidentally highly endowed with a defensive armament.Citation42) These male-initiated pheromone systems can be contrasted to the case of the turnip sawfly, A. rosae ruficornis (see Section III-2), wherein females pharmacophagously acquire clerodendrins (e.g. 37) from C. trichotomum and males select females loaded with the bitter-tasting diterpenoids.Citation74) Sexual selection in these cases appeared to be tightly linked with defensive fitness properties arising from natural selection via plant secondary metabolites.
2. Plant signals for rendezvous cues
Observation of patrolling males of the citrus swallowtail butterflies, P. xuthus and P. protenor, searching for females along the edge of citrus bushes suggests an involvement of some chemical cues from the hosts (personal observations). However, a possible role of the host odor in this precopulatory step has rarely been investigated.Citation75)
Mediterranean fruit fly—α-copaene
A sesquiterpene hydrocarbon, α-copaene (48), initially found in Angelica oil as a potent attractant for males of the Mediterranean fruit flies, Ceratitis capitata (Tephritidae), is also found as a minor leaf volatiles of various plant species, including its major hosts such as orange, guava, and mango.Citation76) Despite the specific attraction of male flies, they never ingest the chemical during exposure to 48, unlike the case of B. dorsalis males in which they incorporate 44 as a pheromone precursor. In a laboratory test, lek-like behavior of C. capitata has been induced by either citrus leaves containing 48 or artificially by using a plastic leaf model thinly coated with 48.Citation77) Mating occurred exclusively on the artificial leaves treated with 48, suggesting the compound potentially serves as a chemical cue to facilitate orientation of flies to the rendezvous site. This represents an “external use” of plant volatiles for courtship other than (or in addition to) their own sex/aggregation pheromones.
In the white-spotted longicorn beetle, Anoplophora malasiaca (Cerambycidae), Yasui et al.Citation78) demonstrated that host plant sesquiterpenes (e.g. 47 in Citrus leaves) serve as a cue in their intraspecific communication. The host-shift of Rhagoletis pomonella (Tephritidae) from its native host downy hawthorn, Crataegus mollis, to introduced domesticated apple, Malus domestica, has been shown to be initiated by specific blends of their native host fruit volatiles where they rendezvous.Citation79) Divergence of incipient populations and speciation in phytophagous insects may be driven by such host-related chemical cues, both in habitat-specific mating choice and in host preference by ovipositing females, although this warrants further in-depth investigations.
V. Floral volatiles in mutualistic associations
Pollination syndromes between higher plants and insects have resulted in the rich flora and fauna of the earth that we see today. Orchids are among the largest and most diverse group of the flowering plants, having radiated via highly specialized associations with specific pollinators. Mutualistic interactions between a group of orchids and pollinator fruit flies via floral synomones were examined as a collaboration projects with Dr Keng-Hong Tan in a chemoecological study of pest fruit flies in the genus of Bactrocera (Tephritidae), particularly for species of economic importance.
Flowers of a fruit fly orchid, Bulbophyllum cheiri (Orchidaceae), attract males of several Bactrocera fruit fly species by floral methyl eugenol (44) in the rain forest of Southeast Asia.Citation80) The flower has a specialized hinged see-saw lip structure which temporarily traps the fruit fly between its lip and column, and transfers pollinia to the back of his thorax. The fly is rewarded by the floral attractant to be used as a precursor for defensive substances and sex pheromonal components (45 and 46) as described in Sections III-2 and IV-1, respectively. In this orchid-fruit fly association, both organisms gain direct reproductive benefits—the orchid flower gets pollinated without having to offer nectar, while the fruit fly boosts its pheromone and defense system with the attractant chemical 44. Thus, the floral volatile is defined as synomone, an interspecific semiochemical that is adaptively advantageous to both the emitting and the receiving organisms. Flowers of another related orchid species, Bulbophyllum vinaceum, produce a complex mixture of phenylpropanoids including 45 and 46 (major), in addition to 44, and attract ME-sensitive fruit flies (Fig. ). The orchid flower seems to endow the male flies not only with precursor attractant (44) but also intact pheromone (45 and 46), although the complete pollinator spectrum and actual role of such a multi-component system of attractant volatiles is unclear.Citation81)
Fig. 5. Floral synomone of fruit fly orchids attracting pollinator Bactrocera fruit flies.
Note: B. vinaceum emits methyl eugenol (ME, 44); B. apertum produces raspberry ketone (RK, 49); B. patens emits zingerone (ZN, 50) which has a hybrid structure between 44 and 49 and attracts both ME-sensitive (e.g. oriental fruit fly, B. dorsalis) and RK-sensitive species (e.g. melon fly, B. cucurbitae).
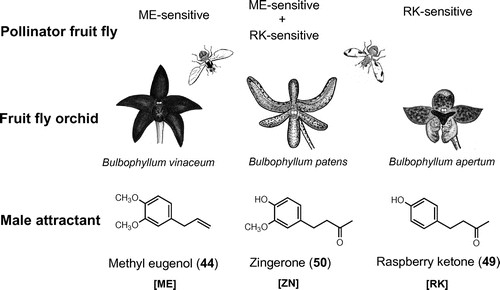
Another fruit fly orchid, Bulbophyllum apertum releases raspberry ketone (RK, 49) in its fragrance to attract several RK-sensitive Bactrocera species such as the melon fly, B. cucubitae.Citation82) The pollination takes place when a fly is compulsively feeding on the see-saw lip and momentarily trapped between the lip and column. The attractant chemical can act either as floral synomone (pollinarium-transported, as in B. albistrigata) or kairomone (accidental removal of pollinarium leading to total pollen wastage, as in B. cucurbitae) depending on the size of the visiting fruit fly species.
Flowers of Bulbophyllum patens attract male of a variety of Bactrocera fruit fly species, which show affinity to either 44 or 49. The attractant component was identified as zingerone (50), a pungent essence of ginger.Citation83) Zingerone conforms to a chemical structure resembling both 44 and 49, and thus shows a potency to attract both ME-sensitive and RK-sensitive fruit fly species (e.g. B. dorsalis, B. carambolae, B. umbrosa, B. cucurbitae, and B. caudata), thereby securing effective pollinators in the complex and dense vegetation in the rain forests. It was demonstrated that B. cucurbitae males that have fed on 50 sequester the intact compound in the rectal pheromone glandsCitation83) and become more successful in mating than unfed males.Citation84) Interestingly, males of an Australian fruit fly, B. jarvisi (a pest of mango fruit) whose specific attractant was initially unknown, was found to be selectively attracted to 50 in the flowers of Bulbophyllum baileyi that the male flies frequently visit.Citation85) This exemplifies a possible use of floral synomones as new monitoring agents for several specific pest species in the orchards.Citation86)
VI. Conclusion
Insects effectively recognize host plants that produce specific primary and secondary metabolites via their finely tuned chemoreceptors. In a similar process, insects reject non-hosts or harmful plants. Host adaptation and speciation in insects are tightly connected to such chemosensory mechanisms—interactions between specific ligands and chemoreceptors. One of the gustatory receptors involved in host recognition for the citrus swallowtail butterfly, P. xuthus, specific to synephrine (8) (Fig. ) has recently been characterized from female tarsal chemosensilla.Citation87) A female-specific chemoreceptive protein was shown to specifically bind to a specific oviposition stimulant, aristolochic acid I (17) for the pipevine swallowtail butterfly, A. alcinous.Citation88) Numerous molecular techniques related to biosynthesis (in plants), biotransformation, or detoxification (in insects) have been employed to provide evidence for possible (co)evolutionary processes between insects and plants.Citation89) The identification of genes related to these processes certainly opens the door to understanding the evolution of host specificity in herbivorous insects. The use of phytochemical cues by insects, both in host finding and in sexual communication, may facilitate a host shift and drive the process of colonization to a new adaptive zone with an explicit genetic isolation from the old host and community. The phytochemical mediation of sexual communication, including rendezvous cues (IV-2) in conjunction with host recognition (I-1, I-2), may be of great importance particularly at the initial stage of speciation, although such a process has not been well understood.Citation75,Citation79)
On another front, phytochemical cues and their derivatives also provide us with innovative tools for pest management. However, in the case of fruit fly attractants, such as the synomones mediating orchid-fruit fly interactions, the intensive use of such allelochemicals in agricultural fields may threaten their communications and their populations, in the natural habitat. We need to understand more about chemical ecological networks in natural ecosystems as well as agricultural ecosystems, to seek a better way to live together.
Acknowledgments
I wish to express my hearty thanks to Professors Emeritus Zenzaburo Kumazawa and late Zenpei Yamashita (Mie University), Professors Emeritus Hiroshi Fukami and late Shoziro Ishii (Kyoto University) for guiding me to this fascinating field of organic chemistry, entomology, and chemical ecology. I also thank Professors William S. Bowers, Wendell L. Roelofs, Paul P. Feeny (Cornell University), Professor Keng-Hong Tan (Universiti Sains Malaysia), and late Dr Miriam Rothschild (UK), for their encouragements and collaboration over a long time. I am grateful to Dr Thomas C Baker for useful comments on the manuscript. Sincere thanks are due to many students and colleagues who shared valuable time for studying insect chemical ecology.
Notes
This review was written in response to the author’s receipt of the JSBBA Award for Senior Scientists in 2013.
References
- Schoonhoven LM, Loon JJA, Dicke M. Insect-plant biology. New York (NY): Oxford University Press; 2005.
- Nishida R. Botyu-Kagaku. Oviposition stimulants of some papilionid butterflies contained in their host plants. 1977;42:133–140.
- Nishida R, Ohsugi T, Kokubo S, Fukami H. Oviposition stimulants of a citrus-feeding swallowtail butterfly, Papilio xuthus L. Experientia. 1987;43:342–344.
- Ohsugi T, Nishida R, Fukami H. Oviposition stimulant of Papilio xuthus, a citrus-feeding swallowtail butterfly. Agric. Biol. Chem. 1985;49:1897–1900.
- Ohsugi T, Nishida R, Fukami H. Multi-component system of oviposition stimulants for a Rutaceae-feeding swallowtail butterfly, Papilio xuthus. Appl. Entomol. Zool. 1991;26:29–40.
- Honda K. Flavanone glycosides as oviposition stimulants in a papilionid butterfly, Papilio protenor. J. Chem. Ecol. 1986;12:1999–2010.
- Honda K. Identification of host-plant chemicals stimulating oviposition by swallowtail butterfly, Papilio protenor. J. Chem. Ecol. 1990;16:325–337.
- Ono H, Nishida R, Kuwahara Y. Oviposition stimulant for a Rutaceae-feeding swallowtail butterfly, Papilio bianor: hydroxycinnamic acid derivative from Orixa japonica. Appl. Entomol. Zool. 2000;35:119–123.
- Ono H, Nishida R, Kuwahara Y. A dihydroxy-γ-lactone as an oviposition stimulant for a Rutaceae-feeding swallowtail butterfly, Papilio bianor. Biosci. Biotechnol. Biochem. 2000;64:1970–1973.
- Feeny P, Sachdev K, Rosenberry L, Carter M. Luteolin 7-O-(6"-malonyl)-β-D-glucoside and trans-chlorogenic acid: oviposition stimulants for the black swallowtail butterfly. Phytochemistry. 1988;27:3439–3448.
- Carter M, Sachdev-Gupta K, Feeny P. Tyramine from the leaves of wild parsnip: a stimulant and synergist for oviposition by the black swallowtail butterfly. Physiol. Entomol. 1998;23:303–312.
- Feeny P. The evolution of chemical ecology: contributions from the study of herbivorous insects. In: Rosenthal GA, Berenbaum MR, editors. Herbivores: their interactions with secondary plant metabolites. Vol. II, Ecological and evolutionary processes. San Diego (CA): Academic Press; 1992. p. 1–44.
- Nishida R. Oviposition stimulants of swallowtail butterflies. In: Scriber JM, Tsubaki Y, and Lederhouse RC, editors. Swallowtail butterflies: their ecology and evolutionary biology. Gainesville (FL): Scientific Publishers; 1995. p. 17–26.
- Nishida R, Fukami H. Oviposition stimulant of an Aristolochiaceae-feeding swallowtail butterfly, Atrophaneura alcinous. J. Chem. Ecol. 1989;15:2565–2575.
- Papaj D, Feeny P, Sachdev-Gupta K, Rosenberry L. D-(+)-Pinitol: an oviposition stimulant for the pipevine swallowtail butterfly, Battus philenor. J. Chem. Ecol. 1992;18:799–815.
- Nishida R. Oviposition stimulant of a zerynthiine swallowtail butterfly, Luehdorfia japonica. Phytochemistry. 1994;36:873–877.
- Ehrlich PR, Raven PH. Butterflies and plants: a study in coevolution. Evolution. 1964;18:586–608.
- Verschaffelt E. The cause determining the selection of food in some herbivorous insects. Proc. K. Ned. Akad. Wet. 1910;13:536–542.
- David WAL, Gardiner BOC. Oviposition and the hatching of the eggs of Pieris brassicae (L.) in a laboratory culture. Bull. Entomol. Res. 1962;53:91–109.
- Traynier RMM, Truscott RJW. Potent natural egg-laying stimulant for cabbage butterfly Pieris rapae. J. Chem. Ecol. 1991;17:1371–1380.
- Nishida R, Fukami H. Ecological adaptation of an Aristolochiaceae-feeding swallowtail butterfly, Atrophaneura alcinous, to aristolochic acids. J. Chem. Ecol. 1989;15:2549–2563.
- Murata T, Mori N, Nishida R. Larval feeding stimulants for a Rutaceae-feeding swallowtail butterfly, Papilio xuthus L. in Citrus unshiu leaves. J. Chem. Ecol. 2011;37:1099–1109.
- Dethier VG. Chemical factors determining the choice of food plants by Papilio larvae. Am. Nat. 1941;75:61–73.
- Hamamura Y, Hayashiya K, Naito K, Matsuura K, Nishida J. Food selection by silkworm larvae. Nature. 1962;194:754–755.
- Tanaka K, Uda Y, Ono Y, Nakagawa T, Suwa M, Yamaoka R, Touhara K. Highly selective tuning of a silkworm olfactory receptor to a key mulberry leaf volatile. Curr. Biol. 2009;19:1–10.
- Van Emden HF. Aphids as phytochemists. In: Harborne JB, editor. Phytochemical ecology: annual proceedings of the Phytochemical Society, No. 8. London: Academic Press; 1972. p. 34–36.
- Montllor CB. The influence of plant chemistry on aphid feeding behavior. In: Bernays EA, editor. Insect–plant interactions III. Boca Ranton (FL): CRC Press; 1991. p. 125–173.
- Takemura M, Nishida R, Mori N, Kuwahara Y. Acylated flavonol glycosides as probing stimulants of a bean aphid, Megoura crassicauda, from Vicia angustifolia. Phytochemistry. 2002;61:135–140.
- Takemura M, Kuwahara Y, Nishida R. Chemical basis of feeding behavior of a bean aphid, Megoura crassicauda, controlled by primary and secondary substances in the host Vicia angustifolia. Entomol. Exp. Appl. 2006;121:51–57.
- Klingauf F. Die Wirkung des Glucosids Phlorizin auf das Wirtswahlverhalten von Rhopalosiphum imsertum (Walk.) und Aphis pomi De Geer (Homoptera: Aphididae). Z. Angew. Entomol. 1971;68:41–55.
- Nishida R, Ohsugi T, Fukami H, Nakajima S. Oviposition deterrent of a Rutaceae-feeding swallowtail butterfly, Papilio xuthus, from a non-host rutaceous plant, Orixa japonica. Agric. Biol. Chem. 1990;54:1265–1270.
- Ono H, Kuwahara Y, Nishida R. Hydroxybenzoic acid derivatives in a non-host rutaceous plant, Orixa japonica, deter both oviposition and larval feeding in a Rutaceae-feeding swallowtail butterfly, Papilio xuthus L. J. Chem. Ecol. 2004;30:287–301.
- Ohta N, Mori N, Kuwahara Y, Nishida R. A hemiterpene glucoside as a probing deterrent of the bean aphid, Megoura crassicauda, from a non-host vetch, Vicia hirsuta. Phytochemistry. 2006;67:584–588.
- Dinan L. Phytoecdysteroids: biological aspects. Phytochemistry. 2001;57:325–339.
- Toong YC, Schooley DEA, Baker FC. Isolation of insect juvenile hormone III from a plant. Nature. 1988;333:170–171.
- Bowers WS, Nishida R. Juvocimenes: potent juvenile hormone mimics from sweet basil. Science. 1980;209:1030–1032.
- Nishida R, Bowers WS, Evans PH. Synthesis of highly active juvenile hormone analogs, juvocimene I and II, from oil of sweet basil, Ocimum basilicum L. J. Chem. Ecol. 1984;10:1435–1451.
- Nishida R, Bowers WS, Evans PH. Juvadecene: discovery of a juvenile hormone mimic in the plant, Macropiper excelsum. Arch. Insect Biochem. Physiol. 1983;1:17–24.
- Duffey SS. Sequestration of plant natural products by insects. Annu. Rev. Entomol. 1980;25:447–477.
- Rothschild M. Secondary plant substances and warning colouration in insects. Symp. R. Entomol. Soc. London. 1972;6:59–83.
- Nishida R. Sequestration of plant secondary compounds by butterflies and moths. Chemoecology. 1995;5/6:127–138.
- Nishida R. Sequestration of defensive substances from plants by Lepidoptera. Annu. Rev. Entomol. 2002;47:57–92.
- Nishida R, Kim CS, Fukami H, Irie R. Ideamine N-oxides: pyrrolizidine alkaloids sequestered by a danaine butterfly, Idea leuconoe. Agric. Biol. Chem. 1991;55:1787–1792.
- Kim CS, Nishida R, Fukami H, Abe F, Yamauchi T. 14-Deoxyparsonsianidine N-oxide: A pyrrolizidine alkaloid sequestered by the giant danaine butterfly, Idea leuconoe. Biosci. Biotechnol. Biochem. 1994;58:980–981.
- Edgar JA. Parsonsieae: ancestral larval foodplants of the Danainae and Ithomiinae. In: Vane-Wright RI, Ackery PR, editors. The biology of butterflies. London: Academic Press; 1984. p. 91–93.
- Nishida R, Rothschild M, Mummery R. A cyanoglucoside, sarmentosin, from the Magpie moth, Abraxas grossulariata, Geometridae: Lepidoptera. Phytochemistry. 1994;36:37–38.
- Nahrstedt A, Walther A, Wray V. Sarmentosin epoxide, a new cyanogenic compounds from Sedum cepaea. Phytochemistry. 1982;21:107–110.
- Nishida R, Rothschild M. A cyanoglucoside stored by a Sedum-feeding Apollo butterfly, Parnassius phoebus. Experientia. 1995;51:267–269.
- Seyama I, Narahashi T. Modulation of sodium channels of squid nerve membrane by grayanotoxin I. J. Pharmacol. Exp. Ther. 1981;219:614–624.
- Nishida R, Fukami H, Iriye R, Kumazawa Z. Accumulation of highly toxic ericaceous diterpenoids by the geometrid moth, Arichanna gaschkevitchii. Agric. Biol. Chem. 1990;54:2347–2352.
- Nishida R, Fukami H. Host plant iridoid-based chemical defense of an aphid, Acyrthosiphon nipponicus against ladybird beetles. J. Chem. Ecol. 1989;15:1837–1845.
- Kamo T, Tokuoka Y, Miyazaki M. Quantification of canavanine, 2-aminoethanol, and cyanamide in Aphis craccivora and its host plants, Robinia pseudoacacia and Vicia angustifolia: effects of these compounds on larval survivorship of Harmonia axyridis. J. Chem. Ecol. 2012;38:1552–1560.
- Boppré M. Redefining “pharmacophagy”. J. Chem. Ecol. 1984;10:1151–1154.
- Nishida R, Fukami H. Sequestration of distasteful compounds by some pharmacophagous insects. J. Chem. Ecol. 1990;16:151–164.
- Nishida R, Fukami H, Miyata T, Takeda M. Clerodendrins: feeding stimulants for the adult turnip sawfly, Athalia rosae ruficornis, from Clerodendron trichotomum (Verbenaceae). Agric. Biol. Chem. 1989;53:1641–1645.
- Nishida R, Kawai K, Amano T, Kuwahara Y. Pharmacophagous feeding stimulant activity of neo-clerodane diterpenoids for the turnip sawfly, Athalia rosae ruficornis. Biochem. Syst. Ecol. 2004;32:15–25.
- Nishida R, Fukami H, Tanaka Y, Magalhães BP, Yokoyama M, Blumenschein A. Isolation of feeding stimulants of Brazilian leaf beetles (Diabrotica speciosa and Cerotoma arcuata) from the root of Ceratosanthes hilariana. Agric. Biol. Chem. 1986;50:2831–2836.
- Nishida R, Yokoyama M, Fukami H. Sequestration of cucurbitacin analogs by New and Old World chrysomelid leaf beetles in the tribe Luperini. Chemoecology. 1992;3:19–24.
- Koyama J, Teruya T, Tanaka K. Eradication of the Oriental fruit fly (Diptera: Tephritidae) from the Okinawa Islands by a male annihilation method. J. Econ. Entomol. 1984;77:468–472.
- Nishida R, Tan KH, Serit M, Lajis NH, Sukari AM, Takahashi S, Fukami H. Accumulation of phenylpropanoids in the rectal glands of males of the Oriental fruit fly, Dacus dorsalis. Experientia. 1988;44:534–536.
- Wee SL, Tan KH. Allomonal and hepatotoxic effects following methyl eugenol consumption in Bactrocera papayae against Gekko monarchus. J. Chem. Ecol. 2001;27:953–964.
- Tan KH, Nishida R. Methyl eugenol: its occurrence, distribution, and role in nature, especially in relation to insect behavior and pollination. J. Insect Sci. 2012;12:1–60.
- Shelly TE, Nishida R. Larval and adult feeding on methyl eugenol and the mating success of male oriental fruit flies, Bactrocera dorsalis (Hendel) (Diptera: Tephritidae). Entomol. Exp. Appl. 2004;112:155–158.
- Nishida R, Schulz S, Kim CS, Fukami H, Kuwahara Y, Honda K, Hayashi N. Male sex pheromone of the giant danaine butterfly, Idea leuconoe. J. Chem. Ecol. 1996;22:949–972.
- Schulz S, Nishida R. The pheromone system of the male danaine butterfly, Idea leuconoe. Bioorg. Med. Chem. 1996;4:341–349.
- Baker TC, Nishida R, Roelofs WL. Close range attraction of female Oriental fruit moths to herbal scent of male hairpencils. Science. 1981;214:1359–1361.
- Nishida R, Fukami H, Baker TC, Roelofs WL, Acree TE. Oriental fruit moth pheromone: attraction of females by an herbal essence. In: Acree TE, Soderlund DM, editors. Semiochemistry: flavors and pheromones. Berlin: Walter de Gruyter; 1985. p. 47–63.
- Shelly TE, Dewire AM. Chemically mediated mating success in male oriental fruit flies, Bactrocera dorsalis (Diptera: Tephritidae). Ann. Entomol. Soc. Am. 1994;87:375–382.
- Tan KH, Nishida R. Sex pheromone and mating competition after methyl eugenol consumption in Bactrocera dorsalis complex. In: McPheron BA, Steck GJ, editors. Fruit fly pests – a world assessment of their biology and management. Port St. Lucie (FL): St. Lucie Press; 1996. p. 147–153.
- Tan KH, Nishida R. Ecological significance of male attractant in the defence and mating strategies of the fruit fly, Bactrocera papayae. Exp. Appl. 1998;89:155–158.
- Wee SL, Tan KH, Nishida R. Pharmacophagy of methyl eugenol by males enhances sexual selection of Bactrocera carambolae (Diptera: Tephritidae). J. Chem. Ecol. 2007;33:1272–1282.
- Tan KH, Tokushima I, Ono H, Nishida R. Comparison of phenylpropanoid volatiles in male rectal pheromone gland after methyl eugenol consumption, and molecular phylogenetic relationship of four global pest fruit fly species – Bactrocera invadens, B. dorsalis, B. correcta and B. zonata. Chemoecology. 2011;21:25–33.
- Tokushima I, Orankanok W, Tan KH, Ono H, Nishida R. Accumulation of phenylpropanoid and sesquiterpenoid volatiles in male rectal pheromonal glands of the guava fruit fly, Bactrocera correcta. J. Chem. Ecol. 2010;36:1327–1334.
- Amano T, Nishida R, Kuwahara Y, Fukami H. Pharmacophagous acquisition of clerodendrins by the turnip sawfly, (Athalia rosae ruficornis) and their role in the mating behavior. Chemoecology. 1999;9:145–150.
- Visser JH. Host odor perception in phytophagous insects. Annu. Rev. Entomol. 1986;31:121–144.
- Fornasiero U, Guitto A, Caporale G, Baccichetti F, Musajo L. Identificazion della sostanza attrattiva per i maschi della Ceratitis capitata, contenunuta nell’olio essenziale dei semi di Angelica archangelica. Gazz. Chim. Ital. 1969;99:700–710.
- Nishida R, Shelly TE, Whittier TS, Kaneshiro KY. α-Copaene, a potential rendezvous cue for the Mediterranean fruit fly, Ceratitis capitata. J. Chem. Ecol. 2000;26:87–100.
- Yasui H, Yasuda T, Fukaya M, Akino T, Wakamura S, Hirai Y, Kawasaki K, Ono H, Narahara M, Kousa K, Fukuda T. Host plant chemicals serve intraspecific communication in the white-spotted longicorn beetle, Anoplophora malasiaca (Thomson) (Coleoptera: Cerambycidae). Appl. Entomol. Zool. 2007;42:255–268.
- Linn C Jr, Feder JL, Nojima S, Dambroski HR, Berlocher SH, Roelofs WL. Fruit odor discrimination and sympatric host race formation in Rhagoletis. Proc. Natl. Acad. Sci. USA. 2011;100:11490–11493.
- Tan KH, Nishida R, Toong YC. Floral synomone of a wild orchid, Bulbophyllum cheiri, lures Bactrocera fruit flies for pollination. J. Chem. Ecol. 2002;28:1161–1172.
- Tan KH, Tan LT, Nishida R. Floral phenylpropanoid cocktail and architecture of Bulbophyllum vinaceum orchid in attracting fruit flies for pollination. J. Chem. Ecol. 2006;32:2429–2441.
- Tan KH, Nishida R. Synomone or kairomone? - Bulbophyllum apertum flower releases raspberry ketone to attract Bactrocera fruit flies. J. Chem. Ecol. 2005;31:509–519.
- Tan KH, Nishida R. Mutual reproductive benefits between a wild orchid, Bulbophyllum patens, and Bactrocera fruit flies via a floral synomone. J. Chem. Ecol. 2000;26:533–546.
- Khoo CCH, Tan KH. Attraction of both sexes of melon fly, Bactrocera cucurbitae to conspecific males – a comparison after pharmacophagy of cue-lure and a new attractant – zingerone. Entomol. Exp. Appl. 2000;97:312–320.
- Tan KH, Nishida R. Zingerone in floral synomone of Bulbophyllum baileyi (Orchidaceae) attracts Bactrocera fruit fly during pollination. Biochem. Syst. Ecol. 2007;35:334–341.
- Fay HAC. A highly effective and selective male lure for Bactrocera jarvisi (Tryon) (Diptera:Tephritidae). Aust. J. Entomol. 2012;51:189–197.
- Ozaki K, Ryuda M, Yamada A, Utoguchi A, Ishimoto H. A gustatory receptor involved in host plant recognition for oviposition of a swallowtail butterfly. Nat. Commun. 2011;2:542.
- Tsuchihara K, Wazawa T, Ishii Y, Yanagida T, Nishida R, Zheng XG, Ishiguro M, Yoshihara K, Hisatomi O, Tokunaga F. Characterization of chemoreceptive protein binding to an oviposition stimulant using a fluorescent micro-binding assay in a butterfly. FEBS Lett. 2008;583:345–349.
- Berenbaum MR, Zangerl AR. Facing the future of plant-insect interaction research: Le retour à la “Raison d’Être”. Plant Physiol. 2008;146:804–811.