Abstract
The production of chemical compounds from renewable resources is an important issue in building a sustainable society. In this study, Escherichia coli was metabolically engineered by introducing T7lac promoter-controlled aroFfbr, pabA, pabB, and pabC genes into the chromosome to overproduce para-aminobenzoic acid (PABA) from glucose. Elevating the copy number of chromosomal PT7lac-pabA-pabB distinctly increased the PABA titer, indicating that elevation of 4-amino-4-deoxychorismic acid synthesis is a significant factor in PABA production. The introduction of a counterpart derived from Corynebacterium efficiens, pabAB (ce), encoding a fused PabA and PabB protein, resulted in a considerable increase in the PABA titer. The introduction of more than two copies of PT7lac-pabAB (ce-mod), a codon-optimized pabAB (ce), into the chromosome of a strain that simultaneously overexpressed aroFfbr and pabC resulted in 5.1 mM PABA from 55.6 mM glucose (yield 9.2%). The generated strain produced 35 mM (4.8 g L−1) PABA from 167 mM glucose (yield 21.0%) in fed-batch culture.
Graphical Abstract
Escherichia coli was metabolically engineered by introducing several genes into the chromosome to overproduce para-aminobenzoic acid from glucose.
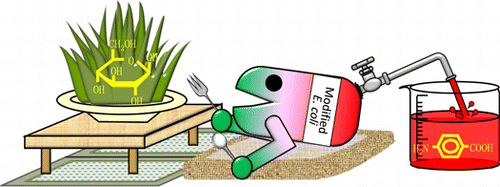
Introduction
Many micro-organisms have the ability to synthesize aromatic compounds from renewable resources such as glucose. Making use of this ability, there have been attempts to produce a variety of aromatic compounds. Many aromatic compounds have been produced biologically from renewable resources at least on the laboratory scale. Various useful aromatic compounds, including shikimate (SHIK),Citation1) p-hydroxybenzoate,Citation2–4) phenol,Citation5) cinnamate,Citation6) coumarate,Citation7,8) p-hydroxystyrene,Citation9–11)styrene,Citation12) tyrosol,Citation13) and tyramine,Citation14) have been produced from glucose by metabolically engineered Pseudomonas putida or Escherichia coli. These achievements show a common strategy that involves metabolic engineering of the SHIK pathway, including pathway modifications or expansions.
p-Aminobenzoic acid (PABA) is used in dyes and feed additives, and as a cross-linking agent for polyurethane resins and a building block for pharmaceuticals, and it has the potential to serve as a building block for aromatic polymers. Currently, PABA is chemically synthesized from fossil fuel resources, but many organisms can synthesize it as an intermediate of folic acid (FA) synthesis. E. coli synthesizes PABA from glucose through the central metabolic pathway, the SHIK pathway, and the FA pathway (Fig. ). First, glucose is converted to phosphoenolpyruvic acid (PEP) via glycolysis and to erythrose-4-phosphate (E4P) via the pentose phosphate pathway, and then 3-deoxy-d-arabino-heptulosonate-7-phosphate (DAHP) is synthesized by aroF-, aroG-, or aroH-encoded DAHP synthase. Through six steps in the SHIK pathway, DAHP is converted to chorismic acid (CHO), the branching point for the synthesis of Phe and Tyr, Trp, ubiquinone (UQ) and related compounds, and FA. PABA is an intermediate of FA synthesis and is synthesized from CHO via a two-step reaction. The first step of PABA synthesis from CHO is catalyzed by aminodeoxychorismate (ADC) synthase subunit II (PABA synthase component II) and ADC synthase subunit I (PABA synthase component I), encoded by pabA and pabB. PabA and PabB form a heterodimer complex, and PabA catalyzes the release of the delta amino group from glutamine as ammonia,Citation15) whereas PabB catalyzes the incorporation of the released ammonia into the para position of CHO as an amino group to form 4-amino-4-deoxychorismic acid (4ADC).Citation16–18) The second step is catalyzed by 4ADC lyase, encoded by pabC, at which PabC catalyzes the formation of PABA by releasing pyruvic acid.Citation19)
Fig. 1. PABA synthesis pathways in E. coli and S. cerevisiae.
Note: E. coli and S. cerevisiae harbor the genes in square brackets and the parentheses, respectively. The product in the gray shadow is the target aromatic compound, PABA. PRE, prephenic acid; OABA, o-aminobenzoic acid.
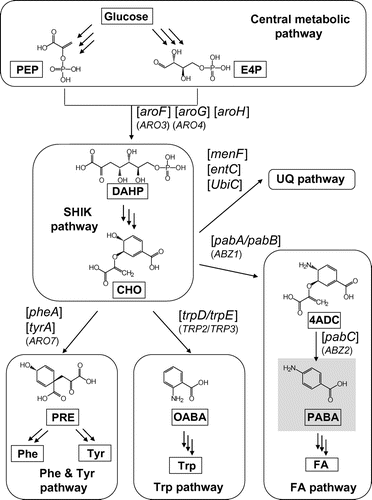
Recently, PABA production from glucose was achieved by means of metabolically engineered Saccharomyces cerevisiae.Citation20) S. cerevisiae synthesizes PABA through a route similar to E. coli. In the study in question, a gene encoding PABA synthase (ABZ1, corresponding to the pabA/pabB complex) was overexpressed with deletion of the genes encoding CHO mutase (ARO7, corresponding to pheA and tyrA) and a portion of the indole-3-glycerolphosphate synthase/anthranilate synthase complex (TRP3, corresponding to trpE) from the chromosome (Fig. ). That is, the gene at the entrance to the FA pathway was overexpressed with deletion of the branched pathways of Phe, Tyr, and Trp synthesis, but the titer and yield of PABA by the constructed strain was not sufficiently high (0.25 mM and <1%). In this study, we aimed at PABA production with higher titer and yield using E. coli. As a strategy for PABA production, we overexpressed not only the genes at the entrance to the FA pathway (pabA and pabB, PABA synthase components II and I), but also the gene at the entrance to the SHIK pathway (aroF, DAHP synthase) and that relevant to the second reaction in the FA pathway (pabC, 4ADC lyase) (Fig. ). Furthermore, we attempted to overexpress a counterpart of pabA and pabB derived from Corynebacterium efficiens for enhancement of PABA production.
Materials and methods
Cloning of genes
Information on the constructed plasmids, vector plasmids, cloned genes, and primers is summarized in Table . Phusion Hot Start DNA Polymerase (Thermo Fisher Scientific, Boston, MA) was used to amplify the genes of interest. The genes encoding the ADC synthase subunit II (pabA), the ADC synthase subunit I (pabB), and the 4ADC lyase (pabC) were amplified from the genomic DNA of E. coli BW25113 with the following primer pairs: pabA, pabA-Nde + pabA-Xho; pabB, pabB-Nde + pabB-Xho; and pabC, pabC-Nde + pabC-Xho. NdeI-XhoI digested amplicons of pabA, pabB, and pabC were introduced into the corresponding site of pET21a-FRTCitation14) to construct pTEI2, pTEI3, and pTEI4, respectively. pabA was also amplified from the genomic DNA with primer pair pabA-Bsp + pabA-Not, digested with BspHI and NotI, and then introduced into the NcoI-NotI site of pETDuet-FRTCitation14) to construct pETDuet-FRT-pabA. Next, a pabB fragment obtained by NdeI-XhoI digestion of pTEI3 was introduced into the corresponding site of pETDuet-FRT-pabA to construct pTEI11 (pETDuet-FRT-pabA-pabB). The gene encoding p-aminobenzoic acid synthase, pabAB (ce), was amplified from the genomic DNA of C. efficiens YS-314 (NBRC100395) with primer pair CePAB-Nde + CePAB-Xho. The NdeI-XhoI-digested amplicon of pabAB (ce) was introduced into the corresponding site of pET21a-FRT to construct pTEI8. Furthermore, pabAB (ce) was synthesized with optimized codon usage for E. coli by the OptimumGeneTM algorithm (GenScript USA, Piscataway, NJ), and was termed pabAB (ce-mod). This gene was inserted into the NdeI-XhoI site of pET21a-FRT to construct pTEI23. The gene encoding DAHP synthase (aroFfbr), fbr for feedback resistance, was cloned into pARO106 in our previous study.Citation14) It was amplified from pARO106 with primer pair EcAroF-Nde + EcAroF-Xho. The NdeI-XhoI-digested amplicon of aroFfbr was introduced into the corresponding site of pET21a-FRT to construct pTEI1.
Table 1. Plasmids constructed in this study.
The GenBank protein IDs for the cloned genes are follows: AroF, AAC75650.1; PabA, AAA58157.1; PabB, AAC74882.1; PabC, AAC74180.1; PabAB (ce), BAC17869.1.
Generation of strains
E. coli BW25113(DE3), genotype lacIq rrnBT14 ΔlacZWJ16 hsdR514 ΔaraBADAH33 ΔrhaBADLD78 dcm(DE3), was used as host strain to generate PABA producers. The genes of interest were introduced into chromosomal DNA of the host strain by a method including Red-mediated recombination, FLP/FRT recombination, and P1 transduction.Citation14) Deletion of the chromosomal tyrR gene for the generation of strain AR-G0Km was achieved by Red-mediated recombination with pKD46 and pKD13.Citation21,22) The strains, their generating procedures, and their genotypes are listed in Table .
Table 2. E. coli strains generated from BW25113(DE3).
Production of PABA
The strains were pre-cultivated overnight in 5 mL of LB-G medium (10 g/L peptone, 5 g/L yeast extract, 10 g/L NaCl, and 8 g/L glucose, pH 7.0) at 27 °C. Fifty microliter of the pre-culture were inoculated into 5 mL of M9M medium (10g/L glucose, 6g/L Na2HPO4, 3 g/L KH2PO4, 0.5 g/L NaCl, 2 g/L NH4Cl, 246.5 mg/L MgSO4·7H2O, 14.7 mg/L CaCl2·2H2O, 13.9 mg/L FeSO4·7H2O, 0.29 mg/L ZnSO4·7H2O, 0.24 mg/L CoCl2·6H2O, and 10 mg/L thiamine hydrochloride) and cultivated at 37 °C with reciprocal shaking at 250 rpm until an OD660 of 0.3 was attained. Next, overexpression of the desired genes was induced by the addition of 1 mM isopropyl-β-D-thiogalactopyranoside (IPTG), and cultivation was continued for 44 h (or 68 h for time course experiments) at 27 °C with reciprocal shaking at 200 rpm.
For fed-batch culture, 2M9M-Y-0.5Glc was used as culture medium. The medium contained (5 g/L glucose, 10 g/L dried yeast extract, 12 g/L Na2HPO4, 6 g/L KH2PO4, 1 g/L NaCl, 4 g/L NH4Cl, 246.5 mg/L MgSO4·7H2O, 14.7 mg/L CaCl2·2H2O, 13.9 mg/L FeSO4·7H2O, 0.29 mg/L ZnSO4·7H2O, 0.24 mg/L CoCl2·6H2O, and 10 mg/L thiamine hydrochloride). Two hundred μL of overnight pre-culture in LB-G medium was inoculated into 20 mL of 2M9M-Y-0.5Glc in a 500 mL-shaking flask. The strain was cultivated at 37 °C with reciprocal shaking at 160 rpm until an OD660 of 1.0 was attained, and IPTG and CaCO3 were added to the culture at concentrations of 1 mM and 5%, respectively. Then cultivation was continued at 27 °C. Glucose and NH4Cl were added to the culture at concentrations of 5 and 2 g/L, respectively, every 8 h.
Analytical methods
A high-performance liquid chromatography system fitted with a photodiode array (SPD-M10AVP, Shimadzu, Kyoto, Japan) and an octadecyl silica column (COSMOSIL 5C18-MS-II, 3.0 × 150 mm, Nacalai Tesque, Kyoto, Japan) was used to measure the PABA, Phe, and Tyr amounts in the supernatants of the cultures.Citation13) The compounds were eluted at a flow rate of 0.4 mL/min of the mobile phase containing methanol and 0.1% trifluoroacetic acid. The methanol concentration was increased from 20 to 80% over 5 min, and then maintained for 10 min. Under these conditions, PABA, Tyr, and Phe were eluted at 3.4, 3.8, and 5.2 min, respectively, with acceptable separation of the compounds. The wavelength for the detection of PABA and Tyr was 222 nm, and that for the detection of Phe was 206 nm. The compounds were quantified with standard curves of the respective commercial chemicals (Tokyo Chemical Industry, Tokyo).
Optical density for cell growth was measured at 660 nm (UV-160A, Shimadzu). Cell dry weight (CDW) was calculated from the OD660 values by the formula CDW (g/L) = OD660 × 0.5.Citation13) The glucose concentration was determined colorimetrically by Glucose CII Test Wako (Wako Pure Chemical Industries, Osaka, Japan). Sodium dodecyl sulfate-polyacrylamide gel electrophoresis (SDS-PAGE) analysis was performed following a previous study.Citation14)
Results
Overexpression of aroF, pabA, pabB, and pabC in E. coli for PABA production
To generate PABA-producing strains, aroF, pabA, pabB, and pabC controlled by the T7lac promoter (PT7lac) were introduced into the E. coli chromosome and overexpressed (Table ). Overexpression of aroFfbr, which functions at the entrance to the SHIK pathway, resulted in Phe and Tyr accumulation, but not PABA accumulation (PABA-1). A similar result was obtained when a gene at the entrance to the FA pathway, pabA (PABA-2) or pabB (PABA-27), or the second gene in the FA pathway, pabC (PABA-5), was overexpressed simultaneously with aroFfbr. Since the proteins encoded by pabA and pabB form a dimer complex, pabA and pabB were overexpressed simultaneously along with aroFfbr. The resulting strain, PABA-3, accumulated small amounts of PABA in the culture. Further overexpression of pabC (PABA-4) enhanced the PABA titer by at least four-fold as compared to that in PABA-3. Deletion of PT7lac-aroFfbr from the chromosome of PABA-4 (PABA-30) led to a decrease in the PABA titer to 0.2 mM. These results indicate that overexpression of four genes, aroFfbr, pabA, pabB, and pabC, is an effective strategy for the generation of a PABA-producing strain.
Table 3. Effects of gene overexpression on PABA production by E. coli.
Elevating the copy number of chromosomal PT7lac-pabA-pabB
Although PABA-4 produced and accumulated PABA, larger amounts of Phe and Tyr accumulated in the culture (Table ). This indicates that the flux into the Phe and Tyr pathway was superior to that into the FA pathway at the branching point of CHO. For the reason, we considered that in the generated strains, 4ADC synthesis by PabA and PabB was not high enough and the enzyme activities were lower than that of CHO mutase (encoded by pheA and tyrA), which converts CHO to prephenic acid in the Phe and Tyr pathway (Fig. ). Hence, the copy number of chromosomal PT7lac-pabA and PT7lac-pabB was increased to enhance intracellular 4ADC synthesis. For this purpose, one to three copies of PT7lac-pabA-pabB were introduced into various loci of the chromosome of the aroFfbr-pabC overexpressing strain, PABA-5. The resulting strains, PABA-7, PABA-8, and PABA-9, harbored one, two, and three copies of chromosomal PT7lac-pabA-pabB, respectively, in addition to PT7lac-aroFfbr and PT7lac-pabC.
PABA production in PABA-7 (one copy), PABA-8 (two copies), and PABA-9 (tree copies) was examined (Table ). Although the genotype of PABA-7 (tyrR::PT7lac-aroFfbr acs::PT7lac-pabA-pabB pflBA::PT7lac-pabC) differed only slightly from that of PABA-4 (tyrR::PT7lac-aroFfbr acs::PT7lac-pabA mtlA::PT7lac-pabB pflBA::PT7lac-pabC), the PABA titer in PABA-7 was lower than that in PABA-4, but the PABA titer increased with elevated copy numbers of chromosomal PT7lac-pabA-pabB in PABA-8 (two copies) and PABA-9 (three copies). Expression of the pabA and pabB genes of PABA-7 (one copy) and PABA-9 (three copies) was analyzed by SDS-PAGE (Fig. (A)). The amount of intracellular PabA protein apparently increased when the copy number of the chromosomal PT7lac-pabA-pabB rose. On the other hand, the PabB protein was not observed distinctly due to low expression, suggesting that the expression of pabB is a limiting factor in PABA production. Since large amounts of Phe and Tyr byproducts still accumulated in PABA production, we considered that higher 4ADC synthetic activity is necessary.
Table 4. PABA Production by E. coli Harboring Various Copy Numbers of PT7lac-pabA-pabB, of PT7lac-pabAB (ce), and of PT7lac-pabAB (ce-mod).
Fig. 2. SDS-PAGE analysis of pabA-pabB- (A) and pabAB (ce)-overexpressing strains (B).
Note: These strains were cultivated in M9M medium at 37 °C until OD660 reached 1.0, and cultivation was continued at 27 °C for 21 h after IPTG addition. Marks and numbers: M, molecular marker; C, BW25113(DE3); 1, PABA-7 harboring PT7lac-pabA-pabB; 2, PABA-9 harboring three copies of PT7lac-pabA-pabB; 3, PABA-23 harboring PT7lac-pabAB (ce); 4, PABA-25 harboring three copies of PT7lac-pabAB (ce). PABA-7, PABA-9, PABA-23, and PABA-25 harbor PT7lac-aroFfbr and PT7lac-pabC in their chromosomes. Solid arrows indicate the respective proteins. Although PabB was not observed distinctly, the value of the molecular mass (51 kDa) calculated from amino acid sequence is indicated by a broken arrow.
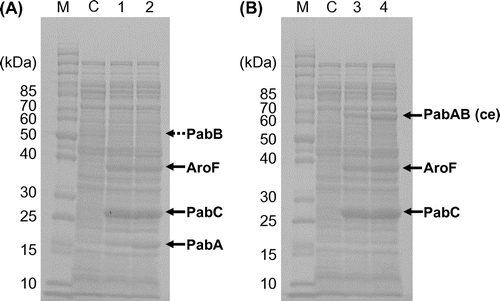
Effects of heterologous pabA and pabB genes on PABA production in E. coli
Aiming to increase the PABA titer further by elevating 4ADC synthetic activity, we tried to use heterologous pabA and pabB genes instead of E. coli ones. A previous study found that some counterparts differed from the E. coli pabA and pabB genes at the structural level.Citation23) That is, pabA and pabB are fused to construct a bifunctional enzyme, in which the glutamine amidotransferase domain (the PabA component) and the 4ADC synthase domain (the PabB component) are located in the N-terminal and C-terminal regions, respectively. This structure appears in a broad range of actinomycetes, plants, and fungi, but rarely in other classes of bacteria, such as Proteobacteria and Firmicutes. Since E. coli PabA and PabB do not form a stable complex,Citation15,16) we considered that fused counterpart has an advantage in PABA synthesis. Hence, this gene, pabAB (ce), was cloned from C. efficiens. Furthermore, a codon-optimized gene of pabAB (ce), pabAB (ce-mod), for overexpression in E. coli was synthesized and cloned.
PT7lac-controlled pabAB (ce) and pabAB (ce-mod) were introduced into the chromosome of the aroFfbr and pabC-overexpressing strain (PABA-5). The generated strains, PABA-12 harboring PT7lac-pabAB (ce) and PABA-23 harboring PT7lac-pabAB (ce-mod), resulted in 3.6 and 3.9 mM PABA titers (Table ), respectively, corresponding to nine-fold higher than that in the strain overexpressing the E. coli counterparts (PABA-4, Table ).
Because increasing the PT7lac-pabA-pabB copy number elevated the PABA titer (Table ), the copy number of chromosomal PT7lac-pabAB (ce-mod) was increased up to three. Strain PABA-25, harboring three copies of chromosomal PT7lac-pabAB (ce-mod), exhibited a 5.1-mM PABA titer, 30% higher than the PABA titer of PABA-23, harboring one copy of chromosomal PT7lac-pabAB (ce-mod). SDS-PAGE analysis revealed that, similarly to the case of PabA (Fig. (A)), the amount of intracellular PabAB (ce) apparently increased with elevating copy numbers of chromosomal PT7lac-pabAB (ce-mod) (Fig. (B)).
PABA productivity by the generated strains
Cell growth, glucose consumption, and product titers during the cultivation of the pabA-pabB overexpressing strain (PABA-9) and the pabAB (ce-mod) overexpressing strain (PABA-25) were measured periodically (Fig. ). Time course experiments revealed the occurrence of by-products (Phe and Tyr) during PABA production. In the cultivation of PABA-9, Phe and Tyr were rapidly produced throughout the entire cultivation period until glucose was exhausted, whereas only small amounts of PABA were produced gradually. Conversely, in the cultivation of PABA-25, PABA was produced rapidly until glucose was exhausted and Phe and Tyr accumulation commenced after 24 h.
Fig. 3. Time course analysis of PABA production by strains PABA-9 and PABA-25.
Note: These strains were cultivated in M9M medium at 37 °C before IPTG addition, and at 27 °C afterwards. Arrows indicate timing of the addition of IPTG for the induction of gene overexpression. Data are averages of triplicate cultures, and the maximum variation was less than 10%. Symbols: hollow circles, OD660; hollow triangles, glucose concentration; solid circles, PABA concentration; solid triangles, Phe concentration; solid squares, Tyr concentration.
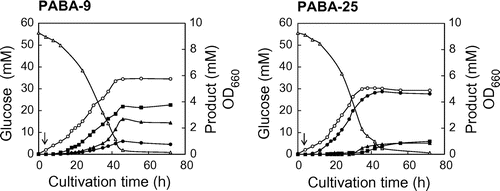
The maximum volumetric and specific productivities of PABA by strains PABA-9 and PABA-25 were determined from data shown in Fig. . The maximum volumetric and specific productivity of PABA by PABA-25 were 5.3 ± 0.8 μmol/L/min and 2.7 ± 0.2 μmol/g CDW/min, respectively, higher than those obtained by means of strain PABA-9 (0.8 ± 0.0 μmol/L/min and 0.3 ± 0.0 μmol/g CDW/min).
As is well known, titers of aromatic amino acids are increased by fed-batch culture.Citation24) Hence, as a preliminary, the PABA titer was examined as fed-batch culture of strain PABA-25 was carried out in a shaking flask (Fig. ). The initial and additional glucose concentrations were set low (0.5%) to prevent acid formation by carbon overflow. A total of 167 mM (3%) glucose was added to the medium, and about 35 mM (4.8 g/L) PABA was produced. PABA yield per glucose molecule was about 21%.
Fig. 4. Time course analysis of PABA production by strains PABA-25 in fed-batch culture.
Note: The strain was cultivated in 2M9M-Y-0.5Glc medium at 37 °C before IPTG addition, and at 27 °C afterwards. Every 8 h, glucose and NH4Cl were supplied to the culture at concentrations of 27.8 mM (5 g/L) and 37.4 mM (2 g/L), respectively. Arrow indicates timing of the addition of IPTG for the induction of gene overexpression. Symbols: hollow triangles, glucose concentration; solid circles, PABA concentration; solid triangles, Phe concentration; solid squares, Tyr concentration.
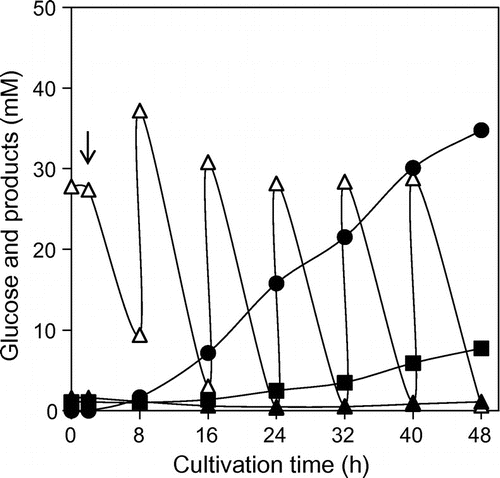
Discussion
In this study, to generate PABA-producing E. coli, pabA and pabB were overexpressed under PT7lac in combination with PT7lac-aroFfbr and PT7lac-pabC. The strain generated, overexpressing both pabA and pabB, distinctly accumulated PABA in culture, and additional overexpression of aroFfbr and pabC enhanced PABA production. It is known that PabB-catalyzed interconversion of CHO and ADC is readily reversible.Citation18) Hence, we speculated that overexpression of pabC-encoded ADC lyase, which catalyzes the irreversible conversion of ADC to PABA, promoted PABA synthesis in the pabA and pabB-overexpressing strain in the present study. On the other hand, because aroFfbr overexpression induces carbon flow into the SHIK pathway,Citation25,26) elevated CHO pools might enhance PABA production, but aroFfbr overexpression caused overflow into the SHIK pathway, and considerable amounts of Phe and Tyr accumulated (PABA-4, Table ). To enhance PABA production further by means of alleviating Phe and Tyr accumulation, the chromosomal copy number of PT7lac-pabA-pabB was increased. The PABA titer increased with increasing copy numbers, suggesting that elevating 4ADC synthesis by pabA and pabB overexpression is the most important point in PABA production by aroF overexpressing strain.
In this study, we found that introduction of the fused type of pabA-pabB derived from C. efficiens was a useful approach for the generation of a PABA-producing strain. The PABA titer in the strain overexpressing pabAB (ce-mod) (PABA-23) was nine-fold higher than that in the one overexpressing pabA and pabB (PABA-4). Furthermore, the accumulation of Phe and Tyr byproducts was appreciably decreased with increasing PABA titers. As for the reason, it was considered that pabAB (ce-mod) was overexpressed in active form in E. coli and that its expression level was higher than pabB of E. coli, although it originated from actinomycetes. In addition to the expression level, we assumed that PabAB (ce) has a structural advantage. Although E. coli PabA does not exhibit glutaminase activity without forming a complex with PabB, it does not form a stable complex with PabB.Citation15,16) On the other hand, E. coli PabB synthesizes 4ADC with ammonia, but the Km value of PabB for ammonia (138 mM) is greater than that of the PabA-PabB complex for glutamine (1.6 mM).Citation17) Hence we speculated that a fused form of PABA synthase, such as PabAB (ce), has an advantage in exhibiting stable activity over a two-component PABA synthase like E. coli one. Since the fused type of pabA-pabB, like pabAB (ce), appears to be widely distributed in actinomycetesCitation27) in addition to eukaryotes such as yeastCitation28) and plants,Citation29) a survey of more suitable enzymes, with respect to enzymatic activity, optimal temperature, and expression level in E. coli, might contribute to the generation of a strain capable of producing PABA at higher titer and yield.
In this study, generated strain PABA-25 harboring PT7lac-aroFfbr, PT7lac-pabA, PT7lac-pabC, and three copies of PT7lac-pabAB (ce-mod) in the chromosome produced PABA from 55.6 mM (1%) glucose at a titer of 5.1 mM and a yield of 9.2%. The titer and the yield values were 20-hold and 10-hold higher than those in a previous study of S. cerevisiae.Citation20) Furthermore, preliminary fed-batch culture resulted in increased PABA titer and yield, of up to 35 mM (4.8 g/L) and 21%, which correspond to 140-fold and 20-fold higher. In addition, the specific productivity of PABA by strain PABA-25 was 2.7 ± 0.2 (μmol/g CDW/min) in M9M medium. This value is similar to those for Tyr and other aromatic compounds previously reported.Citation4,11,13) Hence we consider that our strategy, overexpression of the genes at the entrances to the SHIK (aroF) and the FA (pabA-pabB) pathways and at the second reaction in the FA pathway (pabC), is appropriate in generating a PABA-producing strain.
References
- Krämer M, Bongaerts J, Bovenberg R, Kremer S, Müller U, Orf S, Wubbolts M, Raeven L. Metabolic engineering for microbial production of shikimic acid. Metab. Eng. 2003;5:277–283.10.1016/j.ymben.2003.09.001
- Barker JL, Frost JW. Microbial synthesis of p-hydroxybenzoic acid from glucose. Biotechnol. Bioeng. 2001;76:376–390.10.1002/bit.10160
- Meijnen JP, Verhoef S, Briedjlal AA, Winde JH, Ruijssenaars HJ. Improved p-hydroxybenzoate production by engineered Pseudomonas putida S12 by using a mixed-substrate feeding strategy. Appl. Microbiol. Biotechnol. 2011;90:885–893.10.1007/s00253-011-3089-6
- Verhoef S, Ruijssenaars HJ, de Bont JA, Wery J. Bioproduction of p-hydroxybenzoate from renewable feed stock by solvent-tolerant Pseudomonas putida S12. J. Biotechnol. 2007;132:49–56.10.1016/j.jbiotec.2007.08.031
- Wierckx NJ, Ballerstedt H, de Bont JA, Wery J. Engineering of solvent-tolerant Pseudomonas putida S12 for bioproduction of phenol from glucose. Appl. Environ. Microbiol. 2005;71:8221–8227.10.1128/AEM.71.12.8221-8227.2005
- Nijkamp K, Luijk N, Bont JA, Wery J. The solvent-tolerant Pseudomonas putida S12 as host for the production of CA from glucose. Appl. Microbiol. Biotechnol. 2005;69:170–177.10.1007/s00253-005-1973-7
- Nijkamp K, Westerhof RG, Ballerstedt H, Bont JA, Wery J. Optimization of the solvent-tolerant Pseudomonas putida S12 as host for the production of p-coumarate from glucose. Appl. Microbiol. Biotechnol. 2007;74:617–624.10.1007/s00253-006-0703-0
- Vannelli T, Wei Qi W, Sweigard J, Gatenby AA, Sariaslani FS. Production of p-hydroxycinnamic acid from glucose in Saccharomyces cerevisiae and Escherichia coli by expression of heterologous genes from plants and fungi. Metab. Eng. 2007;9:142–151.10.1016/j.ymben.2006.11.001
- Wei Qi W, Vannelli T, Breinig S, Ben-Bassat A, Gatenby AA, Haynie SL, Sariaslani FS. Functional expression of prokaryotic and eukaryotic genes in Escherichia coli for conversion of glucose to p-hydroxystyrene. Metab. Eng. 2007;9:268–276.10.1016/j.ymben.2007.01.002
- Sariaslani FS. Development of combined biological and chemical process for production of industrial aromatics from renewable resources. Annu. Rev. Microbiol. 2007;61:51–69.10.1146/annurev.micro.61.080706.093248
- Verhoef S, Wierckx N, Westerhof RG, de Winde JH, Ruijssenaars HJ. Bioproduction of p-hydroxystyrene from glucose by the solvent-tolerant bacterium Pseudomonas putida S12 in a two-phase water-decanol fermentation. Appl. Environ. Microbiol. 2009;75:931–936.10.1128/AEM.02186-08
- McKenna R, Nielsen DR. Styrene biosynthesis from glucose by engineered E. coli. Metab. Eng. 2011;13:544–554.10.1016/j.ymben.2011.06.005
- Koma D, Yamanaka H, Moriyoshi K, Ohmoto T, Sakai K. Production of aromatic compounds by metabolically engineered Escherichia coli with an expanded shikimate pathway. Appl. Environ. Microbiol. 2012;78:6203–6216.10.1128/AEM.01148-12
- Koma D, Yamanaka H, Moriyoshi K, Ohmoto T, Sakai K. A convenient method for multiple insertions of desired genes into target loci on the Escherichia coli chromosome. Appl. Microbiol. Biotechnol. 2012;93:815–829.10.1007/s00253-011-3735-z
- Roux B, Walsh CT. p-aminobenzoate synthesis in Escherichia coli: kinetic and mechanistic characterization of the amidotransferase PabA. Biochemistry. 1992;31:6904–6910.10.1021/bi00145a006
- Rayl EA, Green JM, Nichols BP. Escherichia coli aminodeoxychorismate synthase: analysis of pabB mutations affecting catalysis and subunit association. Biochim. Biophys. Acta. 1996;1295:81–88.10.1016/0167-4838(96)00029-5
- Viswanathan VK, Green JM, Nichols BP. Kinetic characterization of 4-amino 4-deoxychorismate synthase from Escherichia coli. J. Bacteriol. 1995;177:5918–5923.
- Ye QZ, Liu J, Walsh CT. p-Aminobenzoate synthesis in Escherichia coli: purification and characterization of PabB as aminodeoxychorismate synthase and enzyme X as aminodeoxychorismate lyase. Proc. Nat. Acad. Sci. USA. 1990;87:9391–9395.10.1073/pnas.87.23.9391
- Green JM, Nichols BP. p-Aminobenzoate biosynthesis in Escherichia coli. Purification of aminodeoxychorismate lyase and cloning of pabC. J. Biol. Chem. 1991;266:12971–12975.
- Krömer JO, Nunez-Bernal D, Averesch NJ, Hampe J, Varela J, Varela C. Production of aromatics in Saccharomyces cerevisiae―a feasibility study. J. Biotechnol. 2013;163:184–193.10.1016/j.jbiotec.2012.04.014
- Baba T, Ara T, Hasegawa M, Takai Y, Okumura Y, Baba M, Datsenko KA, Tomita M, Wanner BL, Mori H. Construction of Escherichia coli K-12 in-frame, single-gene knockout mutants: the Keio collection. Mol. Syst. Biol. 2006. doi: 10.1038/msb4100050.
- Datsenko KA, Wanner BL. One-step inactivation of chromosomal genes in Escherichia coli K-12 using PCR products. Proc. Nat. Acad. Sci. USA. 2000;97:6640–6645.10.1073/pnas.120163297
- James TY, Boulianne RP, Bottoli AP, Granado JD, Aebi M, Kües U. The pab1 gene of Coprinus cinereus encodes a bifunctional protein for para-aminobenzoic acid (PABA) synthesis: implications for the evolution of fused PABA synthases. J. Basic Microbiol. 2002;42:91–103.10.1002/1521-4028(200205)42:2<91::AID-JOBM91>3.0.CO;2-8
- Lütke-Eversloh T, Stephanopoulos G. l-Tyrosine production by deregulated strains of Escherichia coli. Appl. Microbiol. Biotechnol. 2007;75:103–110.10.1007/s00253-006-0792-9
- Ikeda M. Towards bacterial strains overproducing l-tryptophan and other aromatics by metabolic engineering. Appl. Microbiol. Biotechnol. 2006;69:615–626.10.1007/s00253-005-0252-y
- Sprenger GA. From scratch value: engineering Escherichia coli wild type cells to the production of l-phenylalanine and other fine chemicals derived from chorismate. Appl. Microbiol. Biotechnol. 2007;75:739–749.10.1007/s00253-007-0931-y
- Chang Z, Sun Y, He J, Vining LC. p-Aminobenzoic acid and chloramphenicol biosynthesis in Streptomyces venezuelae: gene sets for a key enzyme, 4-amino-4-deoxychorismate synthase. Microbiology. 2001;147:2113–2126.
- Edman JC, Goldstein AL, Erbe JG. Para-aminobenzoate synthase gene of Saccharomyces cerevisiae encodes a bifunctional enzyme. Yeast. 1993;9:669–675.10.1002/(ISSN)1097-0061
- Camara D, Richefeu-Contesto C, Gambonnet B, Dumas R, Rébeillé F. The synthesis of pABA: Coupling between the glutamine amidotransferase and aminodeoxychorismate synthase domains of the bifunctional aminodeoxychorismate synthase from Arabidopsis thaliana. Arch. Biochem. Biophys. 2011;505:83–90.10.1016/j.abb.2010.09.010