Abstract
The anti-proliferative activities of the 6-O-acyl derivatives of d-allose against the human leukemia MOLT-4F cell line were examined. The activity of the 6-O-dodecanoyl derivative (3) was approximately 30 times stronger than that of d-allose. An evaluation of the derivatives of 3 that occurred in a furanose form revealed the pyranose forms of 3 to be important for the anti-proliferative activity.
d-Allose, a naturally occurring rare monosaccharide, is a C-3 epimer of d-glucose that displays such biological activities as an anti-proliferative effect against various human cancer cell lines,Citation1−5) immunosuppressive effect,Citation6) and plant growth inhibitory effect.Citation7) Although d-allose has attracted attention as the functional sugar, the limited availability of this “rare” sugar from natural sources and the lack of a bulk-scale production method have hampered investigations on its mechanism of action and the development of superior analogs. A bio-production method for d-allose has recently been developed, using recombinant enzymes from bacteria,Citation8) which has facilitated studies on the biological activities of d-allose.
d-Allose has been reported to have no effect on normal human cell lines,Citation2,9) but inhibited the proliferation of human ovarian,Citation1) prostate,Citation2) liver,Citation3) leukemia,Citation4) and tongueCitation10) cancer cell lines. d-Allose may therefore be a potential anti-cancer compound with few side effects. However, its effective concentration is relatively high (1–100 mM), and improvements in its activity by developing superior derivatives are needed.
Our group has recently demonstrated that esterification with medium-chain fatty acids at the C-6 position of d-allose increased its inhibitory effect on plant growth, whereas a similar modification to d-glucose did not.Citation7,11) Although why this modification increased its inhibitory activity is not fully understood, we speculate that this structural modification could be applied to enhance the anti-proliferative activity of d-allose.
We evaluated in this present study the anti-proliferative activities of 6-O-acyl-d-allosesCitation12) (1–3; Fig. ) against the human leukemia MOLT-4F cell line. To examine the contribution of the furanose forms of d-allose to this anti-proliferative activity, we also synthesized d-allose derivatives that were predominantly in a furanose form and evaluated their activities.
Fig. 1. Structures of d-glucose, d-allose, and 6-O-acyl-d-alloses (1–3), and synthetic schemes for 5-O-dodecanoyl-d-ribose (4), 6-O-dodecanoyl-5-deoxy-d-allose (6), 6-O-dodecanoyl-5-O-methyl-d-allose (7), methyl 6-O-dodecanoyl-α-d-allofuranoside (8), and methyl 6-O-dodecanoyl-β-d-allofuranoside (9).
Notes: TCDI, 1,1′-thiocarbonyldiimidazole.
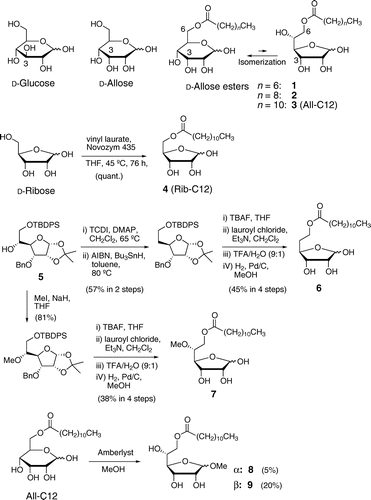
6-O-Octanoyl-(1), decanoyl-(2), and dodecanoyl-d-allose (3: All-C12), and 6-O-dodecanoyl-d-glucose (Glc-C12) were prepared by using immobilized lipase from Candida antarctica (Novozym 435, purchased from Novozymes Japan) as previously described.Citation12) The anti-proliferative activities of these monosaccharide esters were evaluated by a cell growth inhibition assay against the human T-cell leukemia cell line, MOLT-4F, which has shown relatively high sensitivity to d-allose.Citation4) MOLT-4F cells were incubated for 48 h with various concentrations of each compound (10, 30, 100, and 300 μM for 1, 2, 3, and Glc-C12; 100, 300, 1000, and 3000 μM for d-allose), and the cell viability was measured as previously described.Citation4) Cell growth was calculated as a percentage of the untreated control (see the legend to Fig. for details). All the compounds tested inhibited the growth of MOLT-4F cells in a concentration-dependent manner. Compounds 2 and 3 at 300 μm almost completely killed the cells, while the growth inhibition by Glc-C12 was much lower at the same concentration, this was near the concentration needed for micelle formation (CMC for Glc-C12 in water is 365–298 μM).Citation13) Fig. shows the cell growth rate with 100 μM of each compound. While d-allose had little effect at this concentration, 1, 2, and 3, respectively, suppressed cell growth to 62, 46, and 21%, suggesting the effectiveness of C-6 esterification and the importance of the length of the estereal side chain. The growth inhibitory activity of Glc-C12 (57%) was significantly lower than that of 3, and 100 μM of dodecanoic acid (lauric acid) had little effect (data not shown). Since it has previously been reported that d-glucose had little effect on the proliferation of MOLT-4F cells, even at 20 mM,Citation4) these results indicate that the d-allose moiety was important for this inhibitory activity. GI50 values, the concentration required to inhibit cell growth by 50%, estimated from dose-response curves are listed in Table . The GI50 value for 3, respectively, is approximately 30 and 2.5 times smaller than those for d-allose and Glc-C12.
Fig. 2. Growth inhibitory activities of d-allose, 1–3, 6-O-dodecanoyl-d-glucose (Glc-C12), 4, 6, 7, 8, and 9 at 100 μM against the Human Leukemia MOLT-4F cell line.
Notes: Values are the mean, and error bars represent 95% confidence intervals (n = 3–12). The assay was performed under the conditions described by Hirata et al. Citation4) A DMSO stock of the compounds was diluted with the assay medium to a final DMSO concentration of 0.5%. Cell growth was estimated using Cell-counting Kit 8 (Doujin Chemical, Kumamoto, Japan). Absorbance values for the control well (C) and test well (T) were measured at 450 nm, together with the absorbance at time 0 (C0). A blank value was subtracted from each value. Cell growth inhibition (% growth) by each compound was calculated as 100[(T − C0)/(C − C0)] (T ≥ C0) or 100[(T − C0)/C0] (T < C0). *p < 0.05 (Steel–Dwass test).
![Fig. 2. Growth inhibitory activities of d-allose, 1–3, 6-O-dodecanoyl-d-glucose (Glc-C12), 4, 6, 7, 8, and 9 at 100 μM against the Human Leukemia MOLT-4F cell line.Notes: Values are the mean, and error bars represent 95% confidence intervals (n = 3–12). The assay was performed under the conditions described by Hirata et al. Citation4) A DMSO stock of the compounds was diluted with the assay medium to a final DMSO concentration of 0.5%. Cell growth was estimated using Cell-counting Kit 8 (Doujin Chemical, Kumamoto, Japan). Absorbance values for the control well (C) and test well (T) were measured at 450 nm, together with the absorbance at time 0 (C0). A blank value was subtracted from each value. Cell growth inhibition (% growth) by each compound was calculated as 100[(T − C0)/(C − C0)] (T ≥ C0) or 100[(T − C0)/C0] (T < C0). *p < 0.05 (Steel–Dwass test).](/cms/asset/68ef1ecb-0ee8-4964-b460-1aeeb8e7d14f/tbbb_a_882747_f0002_b.gif)
Table 1. GI50 values for d-allose and its derivatives against the human leukemia MOLT-4F cell line.
Ikekawa et al. have reported that 3-O-alkyl-d-glucose and 3-O-alkyl-d-allose exhibited growth inhibitory effects against mouse lymphoma L-5178Y cells.Citation14) However, the inhibitory effects of d-allose ethers were about 5–100-fold weaker than those of the corresponding d-glucose ethers. Therefore, modifying at the C-6 position of d-allose may be promising for the development of stronger anti-proliferative compounds that retain the unique activity of this rare sugar. Additionally, the installation of a C-6 dodecanoyl group on such other monosaccharides as d-mannose, d-gulose, and d-altrose might possibly be effective, because the simple glucose ester Glc-C12 showed significant anti-proliferative activity.
The unique activity of d-allose has been attributed to its structural resemblance to d-glucose which affects cellular metabolism and signal transduction.Citation15) However, unlike d-glucose, All-C12 as well as d-allose have been shown to predominantly exist in not only 6-membered pyranose forms, but also 5-membered furanose forms in a solution (pyranoses/furanoses = ca. 9:1).Citation16) Fig. shows that the orientation of the hydroxyl group at the C-3 position of d-allofuranose was the same as that of biologically important d-ribofuranose, the sugar moiety of RNA. Whether the furanose forms of the d-allose ester would contribute to its anti-proliferative activity was unknown, which led us to synthesize four derivatives of All-C12 (6, 7, 8, and 9; Fig. ) and 5-O-dodecanoyl-d-ribose (4) that were restricted to the furanose forms, and to evaluate their activities.
Regioselective acylation at the C-5 position of d-ribose, existing mainly in pyranose forms,Citation17) was achieved by lipase-catalyzed selective transesterificationCitation18) to quantitatively produce 5-O-dodecanoyl-d-riboseFootnote1 (4, Rib-C12; Fig. ). The synthesis of 5-deoxy-All-C12 (6) and 5-O-methyl-All-C12 (7) was initiated from known protected d-allofuranose 5Citation19) (Fig. ). Radical deoxygenation of the 5-OH group of 5 by Barton’s procedureCitation20,21) [1,1’-thiocarbonyldiimidazole (TCDI); Bu3SnH reduction], followed by deprotection of the TBDPS group by tetra-n-butylammonium fluoride (TBAF), acylation of a primary hydroxyl group at the C-6 position, acidic hydrolysis of the acetonide group, and catalytic hydrogenation of the benzyl group produced 6Footnote2 in six steps with an overall yield of 26%. Methylation of the hydroxyl group at the C-5 position of 5, followed by similar deprotection/acylation/deprotection steps provided 7Footnote3 in five steps with an overall yield of 31%. Treating All-C12 with methanol and H+ ion exchange resin (Amberlyst 15DRY) produced a crude mixture of methyl allofuranosides and methyl allopyranosides (56%, furanosides:pyranosides = ca. 4:1), which was followed by purification using reversed- and normal-phase HPLC, gave methyl 6-O-dodecanoyl-d-allofuranosides 8Footnote4 (α isomer, 5%) and 9Footnote5 (β isomer, 20%). The low yield of pure methyl allofuranosides may have been due to migration of the 6-O-acyl group to the 5-OH group under the HPLC conditions, and pure methyl allopyranosides could not be obtained for the assay. The anti-proliferative activities of these furanose derivatives against MOLT-4F cells were evaluated as already described. The effect of 4 (Rib-C12) at 100 μM was slightly lower than that of 3, and the effects of 6 and 7 at 100 μM were similar to that of Glc-C12 (Fig. ). The respective GI50 values for 4, 6, and 7, which have no free hydroxyl group at C-5, were 76, 120, and 100 μM (Table ). The anti-proliferative activities of methyl allofuranosides 8 and 9 that retain the 5-OH group were similar to those of furanose derivatives 6 and 7 (the respective GI50 values for 8 and 9 were 100 and 110 μM). These results suggest that the restriction to a furanose form, regardless of the modification of the 5-OH group, might have been responsible for their lower activities, and that the pyranose forms, not the furanose form of All-C12 may have been important for its anti-proliferative activity.
d-Allose taken up into cells has previously been shown to be initially phosphorylated by hexokinase which is essential for its biological activity.Citation15) However, the d-allose esters have no primary hydroxyl group to be phosphorylated. An investigation into the mechanism of action of the d-allose esters is needed to further develop superior derivatives.
In conclusion, 6-O-acyl-d-alloses were found to have an anti-proliferative effect on human leukemia MOLT-4F cells, and the length of the acyl chain was found to be important for this activity. An evaluation of the derivatives of 6-O-acyl-d-allose restricted to the furanose form indicated that the 6-membered pyranose form of 6-O-acyl-d-allose may have contributed to its anti-proliferative activity. As just mentioned, modifying the C-6 position of d-allose is promising for the development of derivatives with the unique features of d-allose. Structural optimization of the C-6 side chain of d-allose esters is currently underway.
Funding
This work was partly supported by grant-aid for young scientists (B) [grant number 23780117] to R.C.Y. from The Ministry of Education, Culture, Sports, Science and Technology of Japan.
Notes
Abbreviations: All-C12, 6-O-dodecanoyl-d-allose; Glc-C12, 6-O-dodecanoyl-d-glucose; Rib-C12, 5-O-dodecanoyl-d-ribose.
1. 5-O-Dodecanoyl-d-ribose (4): [α]29D + 8.8° (c 0.385, MeOH). 1H-NMR (600 MHz, 295 K, CD3OD, 0.149 M, α-furanose/β-furanose = 0.35:1) for β-furanose, δ ppm: 0.90 (3H, t, J = 7.1 Hz), 1.26–1.34 (16H, m), 1.61 (2H, m), 2.35 (2H, t, J = 7.4 Hz), 3.83 (1H, dd, J = 4.7, 0.9 Hz), 4.01 (1H, td, J = 6.8, 3.4 Hz), 4.06–4.14 (1H, m), 4.09 (1H, t, J = 5.8 Hz), 4.34 (1H, dd, J = 11.5, 3.4 Hz), 5.12 (1H, d, J = 0.7 Hz) ppm; for α-furanose, δ ppm: 0.90 (3H, t, J = 7.1 Hz), 1.29–1.33 (16H, m), 1.61 (2H, m), 2.35 (2H, t, J = 7.4 Hz), 3.92 (1H, t, J = 5.6 Hz), 3.97 (1H, dd, J = 5.6, 4.1 Hz), 4.06–4.14 (2H, m), 4.25 (1H, dd, J = 11.7, 3.3 Hz), 5.24 (1H, d, J = 4.1 Hz). 13C-NMR (125 MHz, 295 K, CD3OD, 0.0112 M) for β-furanose, δ ppm: 14.5, 23.8, 26.0, 30.3, 30.5, 30.5, 30.7, 30.8 (2C), 33.1, 35.0, 66.7, 72.8, 77.0, 81.2, 103.3, 175.4; for α-furanose, δ ppm: 14.5, 23.8, 26.0, 30.2, 30.5, 30.5, 30.7, 30.8 (2C), 33.1, 34.9, 65.3, 72.3, 72.4, 81.5, 98.1, 175.2. HR-FAB-MS m/z 333.2265 (MH+, calcd. for C17H33O6, 333.2277).
2. 5-Deoxy-6-O-dodecanoyl-d-allose (6): [α]29D + 12.7º (c 0.186, MeOH). 1H-NMR (600 MHz, 296 K, CD3OD, 0.0266 M, α-furanose/β-furanose = 0.34:1) for β-furanose, δ ppm: 0.89 (3H, t, J = 7.4 Hz), 1.29–1.33 (16H, m), 1.59 (2H, m), 1.82–1.88 (1H, m), 2.03 (1H, m), 2.31 (2H, t, J = 7.4 Hz), 3.82 (1H, dd, J = 4.8, 0.8 Hz), 3.89 (1H, m), 3.96–4.01 (1H, m), 4.19–4.26 (2H, m), 5.09 (1H, s); for α-furanose, δ ppm: 0.89 (3H, t, J = 7.4 Hz), 1.29–1.33 (16H, m), 1.59 (2H, m), 1.74–1.78 (1H, m), 1,95–1.98 (1H, m), 2.31 (2H, t, J = 7.4 Hz), 3.78 (1H, t, J = 5.8 Hz), 3.96–4.01 (2H, m), 4.19–4.26 (2H, m), 5.22 (1H, d, J = 4.1 Hz). 13C-NMR (125 MHz, 296 K, CD3OD, 0.0266 M) for β-furanose, δ ppm: 14.5, 23.8, 26.1, 30.3, 30.4, 30.5, 30.6, 30.8 (2C), 33.1, 35.1, 35.2, 62.7, 76.0, 77.3, 79.9, 103.2, 175.7; for α-furanose, δ ppm: 14.5, 23.8, 26.1, 30.3, 30.4, 30.5, 30.6, 30.8 (2C), 33.1, 33.8, 35.1, 62.5, 72.2, 75.7, 79.9, 97.6, 175.6. HR-FAB-MS m/z 347.2437 (MH+, calcd. for C18H35O6, 347.2434).
3. 6-O-Dodecanoyl-5-O-methyl-d-allose (7): [α]27D + 5.6° (c 0.163, MeOH). 1H-NMR (600 MHz, 296 K, CD3OD, 0.0397 M, α-furanose/β-furanose = 0.4:1) for β-furanose, δ ppm: 0.89 (3H, t, J = 7.0 Hz), 1.29–1.32 (16H, m), 1.60–1.64 (2H, m), 2.34 (2H, t, J = 7.4 Hz), 3.47 (3H, s), 3.51–3.53 (1H, td, J = 5.7, 3.1 Hz), 3.83 (1H, dd, J = 4.8, 1.6 Hz), 3.91 (1H, t, J = 5.8 Hz), 4.13 (1H, dd, J = 12.0, 6.0 Hz), 4.28 (1H, dd, J = 6.3, 4.8 Hz), 4.40 (1H, dd, J = 12.0, 3.2 Hz), 5.11 (1H, d, J = 1.6 Hz); for α-furanose, δ ppm: 0.89 (3H, t, J = 7.0 Hz), 1.29–1.32 (16H, m), 1.60–1.64 (2H, m), 2.34 (2H, t, J = 7.4 Hz), 3.44 (3H, s) 3.46–3.47 (1H, m), 3.95 (1H, dd, J = 5.8, 4.2 Hz), 4.05 (1H, t, J = 4.2 Hz), 4.08 (1H, dd, J = 5.8, 4.2 Hz), 4.11 (1H, dd, J = 11.9, 5.9 Hz), 4.29 (1H, dd, J = 11.9, 4.0 Hz), 5.20 (1H, d, J = 4.2 Hz). 13C-NMR (125 MHz, 296 K, CD3OD, 0.0397 M) for β-furanose, δ ppm: 14.5, 23.8, 26.1, 30.2, 30.4, 30.5, 30.6, 30.8, 33.1, 35.1, 49.3, 59.0, 64.2, 73.0, 77.2, 82.0, 82.6, 103.1, 175.4; for α-furanose, δ ppm: 14.5, 23.8, 26.0, 30.2, 30.4, 30.5, 30.6, 30.8, 33.1, 35.0, 49.6, 59.3, 64.0, 71.6, 72.7, 80.9, 84.3, 97.9, 175.3. HR-FAB-MS m/z 399.2357 (MNa+, calcd. for C19H36O7Na, 399.2359).
4. Methyl 6-O-dodecanoyl-α-d-allofuranoside (8): [α]19D + 61.9° (c 0.084, MeOH). 1H-NMR (600 MHz, 295 K, CD3OD, 0.0011 M), δ ppm: 0.89 (3H, t, J = 7.0 Hz), 1.30 (16H, m), 1.62 (2H, m), 2.36 (2H, t, J = 7.4 Hz), 3.42 (3H, s), 3.79–3.81 (1H, m), 3.92 (1H, dd, J = 5.2, 2.9 Hz), 3.96 (1H, dd, J = 6.7, 4.3 Hz), 4.06 (1H, dd, J = 6.7, 2.9 Hz), 4.09 (1H, dd, J = 11.5, 6.7 Hz), 4.17 (1H, dd, J = 11.5, 4.3 Hz), 4.82 (1H, d, J = 4.3 Hz). 13C-NMR (150 MHz, 296 K, CD3OD, 0.0023 M), δ ppm: 14.48, 23.78, 26.04, 30.25, 30.47, 30.52, 30.79 (2C), 33.12, 34.98, 49.60, 55.58, 66.48, 70.57, 70.81, 73.18, 86.55, 104.37, 167.66. HR-FAB-MS m/z: 377.2541 (MH+, calcd. for C19H37O7, 377.2539).
5. Methyl 6-O-dodecanoyl-β-d-allofuranoside (9): [α]16D − 14.3° (c 0.137, MeOH). 1H-NMR (600 MHz, 295 K, CD3OD, 0.0016 M), δ ppm: 0.90 (3H, t, J = 6.9 Hz), 1.29 (16H, m), 1.62 (2H, t, J = 7.3 Hz), 3.36 (2H, t, J = 6.5 Hz), 3.34 (3H, s), 3.77 (1H, td, J = 6.7, 3.1 Hz), 3.86 (1H, t, J = 6.5 Hz), 3.88 (1H, dd, J = 4.8, 0.9 Hz) 4.06 (1H, dd, J = 11.6, 6.9 Hz), 4.27 (1H, dd, J = 6.1, 5.0 Hz), 4.28 (1H, dd, J = 11.6, 3.1 Hz) 4.73 (1H, d, J = 0.9 Hz). 13C-NMR (150 MHz, 295 K, CD3OD, 0.0016 M), δ ppm: 14.48, 23.79, 26.06, 30.27, 30.47, 30.52, 30.66, 30.79 (2C), 33.12, 35.04, 55.67, 67.07, 72.28, 73.52, 76.47, 84.44, 110.12, 175.62. HR-FAB-MS m/z: 377.2541 (MH+, calcd. for C19H37O7, 377.2539).
References
- Sui L, Dong Y, Watanabe Y, Yamaguchi F, Hatano N, Izumori K, Tokuda M. Growth inhibitory effect of d-allose on human ovarian carcinoma cells in vitro. Anticancer Res. 2005;25:2639–2644.
- Naha N, Lee HY, Jo MJ, Chung BC, Kim SH, Kim MO. Rare sugar d-allose induces programmed cell death in hormone refractory prostate cancer cells. Apoptosis. 2008;13:1121–1134.10.1007/s10495-008-0232-7
- Sui L, Dong Y, Watanabe Y, Yamaguchi F, Hatano N, Tsukamoto I, Izumori K, Tokuda M. The inhibitory effect and possible mechanisms of d-allose on cancer cell proliferation. Int. J. Oncol. 2005;27:907–912.
- Hirata Y, Saito M, Tsukamoto I, Yamaguchi F, Sui L, Kamitori K, Dong Y, Uehara E, Konishi R, Janjua N, Tokuda M. Analysis of the inhibitory mechanism of d-allose on MOLT-4F leukemia cell proliferation. J. Biosci. Bioeng. 2009;107:562–568.
- Yamaguchi F, Takata M, Kamitori N, Nonaka M, Dong Y, Sui L, Tokuda M. Rare sugar d-allose induces specific up-regulation of TXNIP and subsequent G1 cell cycle arrest in hepatocellular carcinoma cells by stabilization of p27kip1. Int. J. Oncol. 2008;32:377–385.
- Hossain MA, Wakabayashi H, Goda F, Kobayashi S, Maeba T, Maeta H. Effect of the immunosuppressants FK506 and d-allose on allogenic orthotopic liver transplantation in rats. Transplant. Proc. 2000;32:2021–2023.10.1016/S0041-1345(00)01540-2
- Afach G, Kawanami Y, Kato-Noguchi H, Izumori K. Practical production of 6-O-octanoyl-d-allose and its biological activity on plant growth. Biosci. Biotechnol. Biochem. 2006;70:2010–2012.10.1271/bbb.60150
- Granström TB, Takata G, Tokuda M, Izumori K. Izumoring: a novel and complete strategy for bioproduction of rare sugars. J. Biosci. Bioeng. 2004;97:89–94.
- Yamaguchi F, Kamitori K, Sanada K, Horii M, Dong Y, Sui L, Tokuda M. Rare sugar d-allose enhances anti-tumor effect of 5-fluorouracil on the human hepatocellular carcinoma cell line HuH-7. J. Biosci. Bioeng. 2008;106:248–252.10.1263/jbb.106.248
- Hoshikawa H, Mori T, Mori N. In vitro and in vivo effects of d-allose: up-regulation of thioredoxin-interacting protein in head and neck cancer cells. Ann. Otol. Rhinol. Laryngol. 2010;119:567–571.
- Kobayashi M, Ueda M, Furumoto T, Kawanami Y. Retarding activity of 6-O-acyl-d-alloses against plant growth. Biosci. Biotechnol. Biochem. 2010;74:216–217.10.1271/bbb.90713
- Afach G, Kawanami Y, Izumori K. Synthesis of d-allose fatty acid esters via lipase-catalyzed regioselective transesterification. Biosci. Biotechnol. Biochem. 2005;69:833–835.10.1271/bbb.69.833
- Ferrer M, Comelles F, Plou FJ, Cruces MA, Fuentes G, Parra JL, Ballesteros A. Enzymatic acylation of di- and trisaccharides with fatty acids: choosing the appropriate enzyme, support and solvent. Langmuir. 2002;18:667–673.10.1021/la010727g
- Ikekawa T, Irinoda K, Saze K, Katori T, Matsuda H, Ohkawa M, Kosik M. Studies on synthesis of 3-O-alkyl-d-glucose and 3-O-alkyl-d-allose derivatives and their biological activities. Chem. Pharm. Bull. 1987;35:2894–2899.10.1248/cpb.35.2894
- Stoltzman CA, Kaadige MR, Peterson CW, Ayer DE. MondoA senses non-glucose sugars: regulation of thioredoxin-interacting protein (TXNIP) and the hexose transport curb. J. Biol. Chem. 2011;286:38027–38034.10.1074/jbc.M111.275503
- Collins PM, Ferrier RJ. Monosaccharides. New York (NY): Wiley; 1995.
- Angyal SJ. The Composition and conformation of sugars in solution. Angew. Chem. Int. Ed. 1969;8:157–166.
- Pulido R, Ortiz FL, Gotor V. Enzymatic regioselective acylation of hexoses and pentoses using oxime esters. J. Chem. Soc., Perkin. Trans. 1. 1992:2891–2897.
- Haines AH. Synthesis of 6,6′-ether linked disaccharides from d-allose, d-galactose, d-glucose and d-mannose; evidence on the structure of coyolosa. Org. Biomol. Chem. 2004;2:2352–2358.10.1039/b407468h
- Barton DHR, McCombie SW. A new method for the deoxygenation of secondary alcohols. J. Chem. Soc., Perkin. Trans. 1. 1975:1574–1585.10.1039/p19750001574
- Hartwig W. Modern methods for the radical deoxygenation of alcohols. Tetrahedron. 1983;39:2609–2645.10.1016/S0040-4020(01)91972-6