Abstract
The isolation of biosurfactant-producing yeasts from food materials was accomplished. By a combination of a new drop collapse method and thin-layer chromatography, 48 strains were selected as glycolipid biosurfactant producers from 347 strains, which were randomly isolated from various vegetables and fruits. Of the producers, 69% were obtained from vegetables of the Brassica family. Of the 48 producers, 15 strains gave relatively high yields of mannosylerythritol lipids (MELs), and were identified as Pseudozyma yeasts. These strains produced MELs from olive oil at yields ranging from 8.5 to 24.3 g/L. The best yield coefficient reached 0.49 g/g as to the carbon sources added. Accordingly, MEL producers were isolated at high efficiency from various vegetables and fruits, indicating that biosurfactant producers are widely present in foods. The present results should facilitate their application in the food and related industries.
Graphical Abstract
Mannosylerythritol lipids (MELs) producers were isolated from food materials by an improved drop collapse method and thin-layer chromatography.
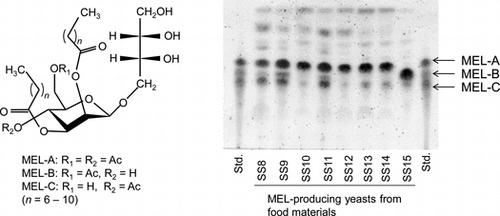
Key words:
Biosurfactants (BSs) are surface-active compounds produced by a variety of microorganisms. They have attracted considerable interest in recent years due to their unique properties (e.g., biodegradability, biocompatibility, mild production conditions, and various functions)Citation1Citation–Citation3) as compared to chemically synthesized surfactants. Despite the many advantages of using BSs in various technology areas, commercial applications of them have been limited. Although some of BSs are used in cosmetics,Citation4Citation–Citation6) there have been few reports on the feasibility of using BSs in the food, pharmaceutical, and related industries. This is probably due to a lack of information on the safety of BSs and their producers. Indeed, many BS producers have been isolated from soil contaminated by oils or hydrocarbons and are taxonomically different from conventional yeasts and bacteria.Citation7) Hence, we focused on the screening of BS producers from food materials, aiming to facilitate applications in the food and related industries.
Among the BSs hitherto reported, glycolipid types are the most promising in view of high productivity and multifunctionality. For example, sophorolipids and mannosylerythritol lipids (MELs, Fig. ) are representative glycolipid BSs, abundantly produced by various yeast strains belonging to the genera Starmerella and Pseudozyma at yields of 100 g/L or more.Citation1CitationCitation–Citation4) These BSs show not only excellent interfacial and self-assembling propertiesCitation8Citation–Citation10) but also versatile biological actions, including antitumor and anti-inflammatory effects.Citation11,12) Many yeast strains are known to be present in various food materials.Citation13,14) Thus, the selection of BS producers from yeasts appears to be preferable.
In screening of BS producers, various drop collapse methods are often used in view of ease of handling and low cost,Citation15,16) but one of the major drawbacks of these methods is a relatively prominent background, which is caused by hydrophobic compounds such as vegetable oils or hydrocarbons employed as carbon sources. Hence, we also paid attention to improvements to the conventional drop collapse method, based on our previous study of BS production from water-soluble carbon sources.Citation17)
In this study, we targeted mainly yeast strains in food materials and undertook the isolation of BS producers by an improved collapse method. Here, we explain for the first time that glycolipid BS producers are widely present in various vegetables and fruits. We also attempted a taxonomic characterization of the isolated yeasts and examined glycolipid production of them.
Materials and methods
Microorganisms
The Pseudozyma antarctica T-34Citation18) and Pseudozyma sp. KM-160Citation19) used in this study were isolated as MEL-A and MEL-B producer respectively, as reported previously. Pseudozyma tsukubaensis JCM 10324T, another MEL-B producer,Citation20) was purchased from the Japan Collection of Microorganisms. Pseudozyma graminicola CBS 10092, a MEL-C producer,Citation21) was from the Centraalbureau voor Schimmelcultures (CBS) of the Institute of the Royal Netherlands Academy of Arts and Sciences. Starmerella bombicola NBRC 10243, a sophorolipid producer,Citation1,4) was from the National Institute of Technology and Evaluation (NITE) of the Biological Resource Center (NBRC). Saccaromyces cerevisiae NBRC 0216, NBRC 1953, and NBRC 0309 were also from NBRC and were used as non-BS producers. They were stored at 4 °C and renewed every 2 weeks.
Molecular phylogenetic analysis
Nuclear DNA extraction from the yeasts producing BS was carried out by the method of Makimura et al.Citation22) The sequences of internal transcribed spacer 1 (ITS1), 5.8S rRNA gene, and internal transcribed spacer 2 (ITS2) of each yeast strain were obtained from the DNA Data Base of Japan (DDBJ) (http://www.ddbj.nig.ac.jp) and were aligned with Clustal W software.Citation23) A phylogenetic tree was constructed with MEGA5 software (http://www.megasoftware.net/index.php).
Screening of biosurfactant-producing yeasts by the drop collapse method
The screening sources used are listed on Table . Small pieces of food materials (vegetable leaves or fruit bodies) were inserted into a test tube containing 5 mL of a screening medium (100 g/L of glucose, 1% fermented barley extract (FBE), 50 mg/L of chloramphenicol, 50 mg/L of streptomycin, pH 5.0). FBE was kindly supplied by Sanwa Shurui Co., Ltd. (Usa, Japan),Citation24), and the concentration was checked by brix meter. The tubes were then incubated on a reciprocal shaker (150 strokes/min) at 28 °C for 2 d. The culture broths were spread on YM agar plates (10 g/L of glucose, 5 g/L of peptone, 3 g/L of yeast extract, 3 g/L of malt extract, and 2 g/L of agar). The plates were cultivated at room temperature for several days until colonies appeared. The colonies were picked out and cultured again with screening medium (5 mL) in test tubes at 28 °C for 7 d.
Table 1. Screening of biosurfactant-producing yeasts from food materials.
After centrifugation of the resulting culture broth, an aliquot of the supernatant (10 μL) was subjected to the drop collapse method, as follows: The aliquot was dropped on a strip of parafilm (3 cm × 6 cm, American National Can, Chicago, IL) as a hydrophobic surface, and then, the diameter of the droplet was evaluated. Fresh culture medium was used as control. To check the products, the resulting culture broth was also subjected to thin-layer chromatography (TLC), as described below.
Isolation of BSs
The BSs produced were extracted from the culture broth with equal amounts of ethyl acetate. The extracts were analyzed by TLC on silica plates (Silica Gel 60F, Wako, Tokyo) by a solvent system consisting of chloroform/methanol/7N ammonia solution (65:15:2 by vol.). The compounds on the plates were located by charring at 110 °C for 5 min after spraying of anthrone/sulfuric reagent, as previously reported.Citation18) The purified MEL fraction, prepared as reported previously, was used as the standard.Citation19)
Purification of glycolipid BSs
The upper organic layer was separated and evaporated. The concentrated glycolipids were dissolved in chloroform and then purified by silica gel (Wako-gel C-200) column chromatography with a gradient elution of a chloroform/acetone (10:0 to 0:10, v/v) mixture as solvent system.Citation19) The purified glycolipids were used in subsequent experiments.
Structural analysis
Structure analysis of the purified glycolipids was carried out by nuclear magnetic resonance (NMR) analysis [1H NMR and 13C NMR] with a Varian INOVA 400 (400 MHz) methanol-d4. In the NMR study, purified MEL-A and MEL-B prepared, as reported previously, were used as references.Citation19,20)
The molecular weight of the purified glycolipid was measured by matrix-assisted laser desorption/ionization time-of-flight mass spectrometry (MALDI-TOF/MS) (Voyager-DE PRO, Applied Biosystems, Foster City, CA) with an α-cyano-4-hdroxycinnamic acid matrix. The fatty acid profiles of the purified glycolipids were examined by gas chromatography/mass spectrometry (GC/MS), as previously reported.Citation19) Methyl ester derivatives of fatty acids were prepared by mixing the above-mentioned purified glycolipids (10 mg) with 5% HCl/methanol regent (1 mL) (Wako, Tokyo) at 80 °C over 3 h. After the reaction was quenched with water (1 mL), the methyl ester derivatives were extracted with n-hexane and then analyzed by GC/MS (Hewlett Packard 6890 and 5973 N, Agilent Technologies, Tokyo) with a capillary column (TC-WAX, GL-Science, Tokyo) with a temperature gradient programmed from 90 °C (held for 3 min) to 240 °C at 5 °C/min. All regents and solvents are commercially available and were used as received.
Production of glycolipid BSs by isolated yeasts
Seed cultures were prepared by inoculating cells grown on slants into test tubes containing 5 mL of a YM medium at room temperature on a reciprocal shaker (150 strokes/min) over 2 d. Seed cultures (0.1 mL) were transferred to 300-mL Erlenmeyer flasks containing 30 mL of a production medium (50 g/L of olive oil, 3 g/L of NaNO3, 0.25 g/L of KH2PO4, 0.25 g/L of MgSO4 7H2O, 1 g/L of yeast extract, pH 6.0), and then incubated at 28 °C on a rotary shaker (250 rpm) for 7 d, unless otherwise indicated.
Quantification of glycolipid BSs in the culture broth
Quantification of glycolipid BS in the culture broth was performed by high-performance liquid chromatography (HPLC) on a HPLC system (SSPC; Tosoh, Tokyo) equipped with a silica gel column (Inertsil SIL-100A 5 μm, 4.6 mm × 250 mm; GL Science, Tokyo) with a low-temperature evaporative light scattering detector (ELSD-LT; Shimadzu, Kyoto, Japan) using a gradient solvent program consisting of various proportions of chloroform and methanol (from 100:0 to 0:100) at a flow rate of 1 mL/min. Purified MEL-A, MEL-B, and MEL-C, prepared as reported previously, were used as standard.Citation18Citation–Citation20) All measurements reported here represent means for at least three independent experiments.
Results and discussion
Sensitivity of the drop collapse method
Screening of BS-producing yeasts was performed by slightly modifying a previous method using a Parafilm strip.Citation17) To evaluate the sensitivity of the present drop collapse method, first we measured the diameter of a droplet (10 μL) formed from an aqueous solution including various concentrations of purified MEL-A produced by Pseudozyma antarctica T-34 (Fig. ).
Fig. 2. Drop collapse measurement.
Notes: (A) A photograph of droplets containing various concentrations of MEL-A produced by P. antarctica. (B) Diameter of droplets containing various concentrations of MEL-A. The broken line indicates the CMC (2.7 × 10−6 M) of MEL-A.
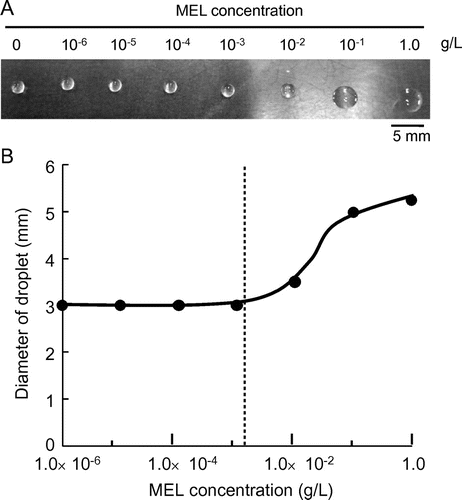
The diameter of the droplet clearly increased with increases in the MEL concentration and reached a plateau at higher concentrations, over 1 g/L. Those droplets including more than 1.0 × 10−2 g/L (1.5 × 10−5 M) of MEL-A spread more widely than the control. It is plausible that the surface tensions of these droplets were significantly decreased by the addition of MEL-A, considering that the critical micelle concentration (CMC) of MEL-A is 1.8 × 10−3 g/L (2.7 × 10−6 M).Citation7) At higher concentrations, over 0.1 g/L, the diameter of the droplet was approximately 1.5-fold larger than that of the control (Fig. ). The observed sensitivity of the drop collapse method was sufficiently high, comparable to that of HPLC with a light scattering detector, for which the detection limit is approximately between 0.1 and 1.0 g/L (data not shown).
Detection of biosurfactant production by the drop collapse method
In conventional drop collapse methods, residual hydrophobic carbon sources, such as vegetable oils and hydrocarbons, exert a drastic background effect on droplet formation. High-level BS producers, such as P. antarcticaCitation17) and Starmerella bombicolaCitation1,4), produce glycolipids not only from vegetable oils but also from water-soluble carbohydrates. In this study, we employed a screening medium including glucose instead of vegetable oils. FBE is a useful organic nutrient that can serve as a nitrogen and mineral source.Citation25) Hence, we also used FBE to simplify the medium composition and to minimize the background effect on droplet formation.
We then checked droplet formation for culture broths prepared with several BS producers. The production of BS was evaluated in terms of drop collapse activity, calculated by the following equation:
where A indicates drop collapse activity (−), Db indicates the diameter of the culture broth after cultivation, and Dm indicates the diameter of the inoculated screening medium before inoculation.
Fig. shows the activities of culture broths prepared with different BS-producing and nonproducing yeasts. All BS producers tested showed high activities, between 1.2 and 1.8, whereas all Saccharomyces strains showed less activity, below 1.1. The present method was thus confirmed properly to reflect the surface tension decrease in the culture broth, induced by extracellular production of BS. Hence, the threshold of the activity for selecting BS producers was set at 1.2 in the following experiments.
Fig. 3. Drop collapse activities of culture broths prepared with various yeast strains.
Notes: Cultivation was performed with a screening medium (100 g/L of glucose, 1% FBE, 100 μL/mL of chloramphenicol, 100 μL/mL of streptomycin, pH 5.0) in a test tube at 28 °C for 2 d. Drop collapse activity was calculated from droplet diameters before and after cultivation. Error bars indicate standard deviation (n = 3).
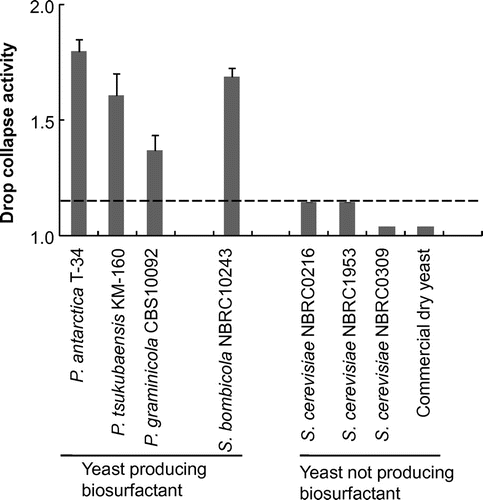
Isolation of biosurfactant producers by the drop collapse method
Yeasts are widely present in the environment, and many of them colonize the surfaces of leaves and flowers.Citation26) Here, we used various vegetables and fruits as BS production. Strains of yeast (n = 347) were isolated randomly from these food materials by enrichment culture with a screening medium. Table summarizes the results of our screening of the yeasts.
On drop collapse assay, 167 strains of the 347 showed high drop collapse activities, indicating that they produce BS from glucose. According to TLC analysis of the culture broths, 48 strains of the 167 were found to produce glycolipid BSs, showing positive reactions, to the anthrone reagent (data not shown).
Of the 48 strains producing glycolipid BS, 33 were isolated from the leaves of qing geng cai and po therb mustard (Brassica rapa), green vegetables belonging to the Brassica family, which includes cabbage, cauliflower, and sprouts. Glycolipid BS producers have been also isolated from fruit bodies, lemon, and apple.
Approximately, 29% of the drop collapse active strains were identified as glycolipid BS producers, indicating that the present screening method is highly selective and practical. More surprisingly, more than 13% of the yeasts randomly isolated from the vegetables and fruits showed production of glycolipid BSs. Trindade et al. have reported that Pseudozyma antarctica, a prolific MEL producer is isolated with high frequency from tropical fruits such as acerola (Malpighia emarginata) and pitanga (Eugenia uniflora).Citation27) These results clearly indicate that glycolipid BS producers are widely on the surfaces of and inside various vegetables and fruits and that they can be taken into the human body with these food materials in daily life.
Taxonomic characterization of isolated yeasts producing glycolipid BSs
We tentatively examined the 48 strains for their productivity of glycolipid BSs with the production medium in a test tube at 28 °C over 7 d. Of the 48 strains, 15 strains (SS1 to SS15) showed relatively high production of glycolipid BSs (Fig. ). In particular, all these glycolipids were estimated to be MELs, based on their Rf values.
Fig. 4. Detection of glycolipid BSs by isolated yeast strains.
Notes: Cultivation was performed with a production medium (50 g/L of olive oil, 3 g/L of NaNO3, 0.25 g/L of KH2PO4, 0.25 g/L of MgSO4 7H2O, 1 g/L of yeast extract, pH 6.0). The products were extracted with ethyl acetate, and the extracts were spotted on a TLC plate and detected by means of anthrone/sulfuric acid reagent.
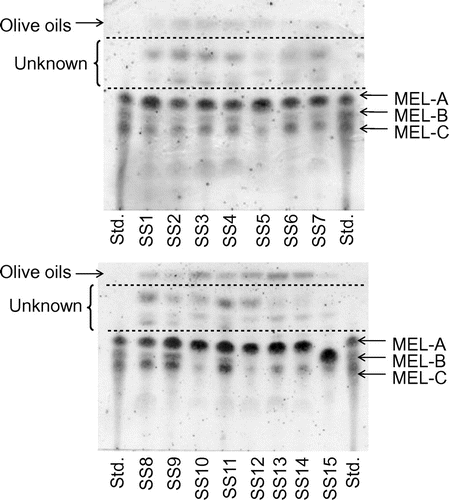
The selected glycolipid BS producers (SS1 to SS15) were partially characterized by molecular phylogenetic analysis with rDNA sequences. Fig. shows a molecular phylogenetic tree for the 15 selected strains and type strains of the genera Pseudozyma and Ustialgo.
Fig. 5. Molecular phylogenetic tree of isolated yeast strains and the genera Pseudozyma and Ustilago.
Notes: DDBJ/GenBank/EMBL accession numbers are indicated in parentheses. Knuc, Kimura’s parameter.
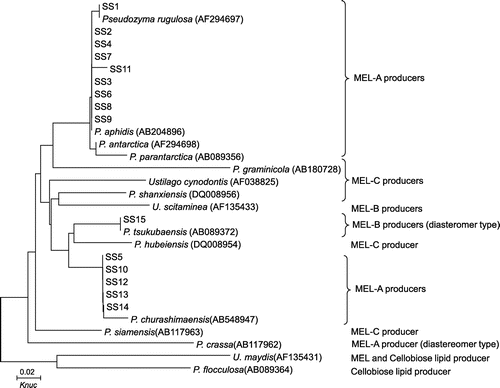
Strain SS1 is positioned close to Pseudozyma rugulosa on the phylogenetic tree, while SS2, SS3, SS4, SS6, SS7, SS8, SS9, and SS11, positioned close to Pseudozyma aphidis. These nine strains produced a mixture of glycolipids corresponding to MEL-A, MEL-B, and MEL-C (Figs. and ). Pseudozyma aphidisCitation28) and Pseudozyma rugulosaCitation29) are known as high-level MEL producers. They provide a mixture of MEL-A, MEL-B, and MEL-C efficiently. It thus seems reasonable that the nine strains related to the high-level producers gave high production of glycolipid BS. Strains SS5, SS10, SS12, SS13, and SS14 are related to Pseudozyma churashimaensis, recently isolated as a new MEL producer from sugar cane, and produced mainly a glycolipid corresponding to MEL-A.Citation30)
In contrast, strain SS15 is closely related to Pseudozyma tsukubaensis,Citation20) and predominantly produced a glycolipid corresponding to MEL-B. Pseudozyma tsukubaensis was recently reported to produce only MEL-B, bearing an erythritol configuration (1-O-β-mannopyranosyl-d-erythritol) opposite to that of conventional MEL-B (4-O-β-mannopyranosyl-d-erythritol) produced by the two high-level producers.Citation20) The glycolipid produced by strain SS15 must thus have the same carbohydrate configuration as that of the MEL-B produced by Pseudozyma tsukubaensis. Accordingly, all 15 strains isolated as glycolipid BS producers were estimated to be of the genus Pseudozyma and were categorized into three groups based on MEL production patterns.
Structural characterization of glycolipid BSs.
Based on TLC analysis, SS11 and SS15 were assumed to be the best producers of MEL-A and MEL-B, respectively, among the strains selected. Hence, we investigated the structure of the glycolipid BSs produced by strain SS11 and strain SS15. The 1H and 13C NMR spectra of the major glycolipid of SS11 corresponded well to those of the purified MEL-A obtained from Pseudozyma antarctica T-34 (Table ).Citation19) The same results were obtained for the major products of the other 13 strains (data not shown). On the other hand, the spectra of the major glycolipid of SS15 corresponded well to those of the MEL-B produced by Pseudozyma tsukubaensis JCM 10324T (Table ), a diastereomer of conventional MEL-B.Citation20) All the 15 strains isolated were confirmed to be producers of MEL.
Table 2. NMR data for the major glycolipids produced by strain SS11.
Table 3. NMR data for the major glycolipids produced by strain SS15.
The fatty-acid profiles of the major glycolipids produced by strains SS11 and SS15 were also analyzed by the GC-MS method (Table ). The fatty-acid profile of the MEL-A produced by strain SS11 was nearly the same as that of MEL-A by Pseudozyma antarctica T-34. The major fatty acids were C8 and C10.Citation29) On the other hand, the fatty-acid profile of MEL-B produced by strain SS15 closely resembled that of MEL-B by Pseudozyma tsukubaensis. The major fatty acids were C8, C12, and C14.Citation20)
Table 4. Fatty acid profiles of MEL-A and MEL-B (diastereomer type) produced by strains SS11 and SS15.
The molecular weight of the MEL-A of SS11 was 672.0 (C8:0 and C10:0 acids) as determined by the main peak ([M+Na]+) on MALDI-TOF/MS analysis. This is consistent with the above described fatty-acid profile. In the same way, the molecular weight of the MEL-B of SS15 was determined to be 658.0 (C8:0 and C12:0 acids), 656.0 (C8:0 and C12:1 acids), and 684.1 (C8:0 and C14:1 acids). By these results, strain SS11 and SS15 were confirmed to be producers of MEL-A and MEL-B (diastereomer type), respectively.
Production of MELs by isolated yeast strains
To confirm the MEL productivity of the 15 strains isolated (SS1 to SS15), we further checked the strains with a production medium including 5% (w/v) of olive oil in an Erlenmeyer flask at 28 °C over 7 d. The total amounts and compositions of the MELs produced by these strains were determined by HPLC (Fig. ). The amount of MELs depended significantly on the producer. As expected, strain SS11, isolated from scallion (Allium fistulosum), gave the best yield of MELs at 24.3 g/L. This corresponds to a yield coefficient of 0.49 g/g (on a weight basis to the olive oil supplied). Strain SS11 appeared to be a promising MEL producer, considering our previous result that Pseudozyma rugulosa, a high-level MEL producer, provided a yield coefficient of 0.5 g/g from soybean oil.Citation29) Although strain SS12 showed the lowest yield among the strains tested, the amount of MELs reached 8.5 g/L.
Fig. 6. Production of glycolipid BSs by isolated yeast strains.
Notes: Cultivation was performed in a production medium (50 g/L of olive oil, 3 g/L of NaNO3, 0.25 g/L of KH2PO4, 0.25 g/L of MgSO4 7H2O, 1 g/L of yeast extract, pH 6.0) at 28 °C for 7 d. The amounts and compositions of the glycolipid BSs produced were determined by HPLC with a light-scattering detector.
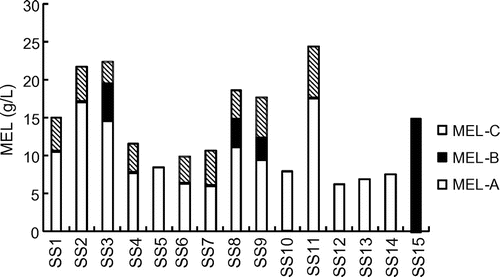
Strain SS15, isolated from leaves of shiso (Perilla frutescens), also gave a relatively high yield of MEL-B (diastereomer type) at 14.8 g/L. This corresponds to a yield coefficient of 0.30 g/g. Likewise, strain SS15 was estimated to be a promising MEL-B producer, considering that P. tsukubaensis JCM 10324T provides MEL-B at a yield coefficient of 0.31 g/g from soybean oil.Citation31)
In this study, we attempted to isolate new BS producers from food materials by an improved drop collapse method and succeeded in obtaining 48 glycolipid BS producers from various vegetables and fruits. Some of these showed relatively high production yields of MELs from olive oil, indicating the MEL producers can be obtained by the present screening method with glucose as carbon source. Judging by these results, glycolipid BS-producing yeasts are likely to be widely present in the environment, especially in dairy foods. The strains of the genus Pseudozyma, which are easily isolated from the phyllosphere of healthy plants, including paddy rice (Oryza sativa) and vegetables, are known as producers of biodegradable plastic-degrading enzymes.Citation32) The present results should facilitate their application in the food and related industries.
Supplemental material
The supplemental material for this paper is available at http://dx.doi.org/10.1080/09168451.2014.882754.
Supplementary Fig. 1 caption
Download MS Word (22.5 KB)Supplementary Fig. 1
Download MS Power Point (156 KB)Acknowledgments
We thank Ms A. Sugimura, a fellow of the Japan Industrial Technology Association, for technical assistance. This study was partially supported by the Industrial Technology Research Grant Program (06A17501c) of the New Energy and Industrial Technology Development Organization (NEDO) of Japan.
References
- Lang S. Biological amphiphiles (microbial biosurfactants). Curr. Opin. Colloid Interface Sci. 2002;7:12–20.
- Kitamoto D, Isoda H, Nakahara T. Functions and potential applications of glycolipid biosurfactants - from energy-saving materials to gene delivery carriers-. J. Biosci. Bioeng. 2002;94:187–201.10.1016/S1389-1723(02)80149-9
- Kitamoto D, Morita T, Fukuoka T, Konishi M, Imura T. Self-assembling properties of glycolipid biosurfactants and their potential applications. Curr. Opin. Colloid Interface Sci. 2009;14:315–328.10.1016/j.cocis.2009.05.009
- Van Bogaert INA, Saerens K, De Muynck C, Develter D, Soetaert W, Vandamme EJ. Microbial production and application of sophorolipids. Appl. Microbiol. Biotechnol. 2007;76:23–34.10.1007/s00253-007-0988-7
- Morita T, Fukuoka T, Imura T, Kitamoto D. Production of mannosylerythritol lipids and their application in cosmetics. Appl. Microbiol. Biotechnol. 2013;97:4691–4700.10.1007/s00253-013-4858-1
- Fukuoka T, Morita T, Imura T, Kitamoto D. Development of highly functional biosurfactants produced by yeasts. Household Pers. Care Today. 2007;1:74–76.
- Banat IM, Makkar RS, Cameotra SS. Potential commercial applications of microbial surfactants. Appl. Microbial. Biotechnol. 2000;53:495–508.10.1007/s002530051648
- Fujii T. Biodetergents. In: Karsa EA, editor. New products and applications in surfactant technology. Sheffield: Sheffield Academic Press; 1998. p. 88–108.
- Imura T, Hikosaka Y, Worakitkanchanakul W, Sakai H, Abe M, Konishi M, Minamikawa H, Kitamoto D. Aqueous-phase behavior of natural glycolipid biosurfactants mannosylerythritol lipid A: Sponge, cubic, and lamella phases. Langmuir. 2007;23:1659–1663.10.1021/la0620814
- Imura T, Ohta N, Inoue K, Yagi N, Negishi H, Yanagishita H, Kitamoto D. Naturally engineered glycolipid biosurfactants leading to distinctive self-assembled structures. Chem. Eur. J. 2006;12:2434–2440.10.1002/(ISSN)1521-3765
- Rodrigues L, Banat IM, Teixeira J, Oliveira R. Biosurfactants: potential applications in medicine. J. Antimicrob. Chemothera. 2006;57:609–618.10.1093/jac/dkl024
- Hardin R, Pierre J, Schulze R, Mueller C, Fu S, Wallner S, Stanek A, Shah V, Gross R, Weedon J. Sophorolipids improve sepsis survival: Effects of dosing and derivatives. J. Surg. Res. 2007;142:314–319.10.1016/j.jss.2007.04.025
- Coton E, Coton M, Levert D, Casaregola S, Sohier D. Yeast ecology in French cider and black olive natural fermentations. Internat. J. Food Microbiol. 2006;108:130–135.10.1016/j.ijfoodmicro.2005.10.016
- Nishida O, Kuwazaki S, Suzuki C, Shima J. Superior molasses assimilation, stress, tolerance, and trehalose accumulation of baker's yeast isolated from dried sweet potatoes. Biosci. Biotechnol. Biochem. 2004;68:1442–1448.10.1271/bbb.68.1442
- Bodour AA, Miller-Maier RM. Application of a modified drop collapse technique for surfactant quantification and screening of biosurfactant-producing microorganisms. J. Microbiol. Methods. 1998;32:273–280.10.1016/S0167-7012(98)00031-1
- Tugrul T, Cansunar E. Detecting surfactant-producing microorganisms by the drop-collapse test. World J. Microbiol. Biotechnol. 2005;21:851–853.
- Morita T, Konishi M, Fukuoka T, Imura T, Kitamoto D. Physiological differences in the formation of the glycolipid biosurfactants, mannosylerythritol lipids, between Pseudozyma antarctica and Pseudozyma aphidis. Appl. Microbial. Biotechnol. 2007;74:307–315.10.1007/s00253-006-0672-3
- Kitamoto D, Akiba S, Hiok C, Tabuchi T. Extracellular accumulation of mannosylerythritol lipids by a strain of Candida antarctica. Agric. Biol. Chem. 1990;54:31–36.10.1271/bbb1961.54.31
- Konishi M, Morita T, Fukuoka T, Imura T, Kakugawa K, Kitamoto D. Production of different types of mannosylerythritol lipids as biosurfactants by the newly isolated yeast strains belonging to the genus Pseudozyma. Appl. Microbial. Biotechnol. 2007;75:521–531.10.1007/s00253-007-0853-8
- Fukuoka T, Morita T, Konishi M, Imura T, Kitamoto D. A basidiomycetous yeast, Pseudozyma tsukubaensis, efficiently produces a novel glycolipid biosurfactant. The identification of a new diastereomer of mannosylerythritol lipid-B. Carbohydrate Res. 2008;343:555–560.10.1016/j.carres.2007.11.023
- Morita T, Konishi M, Fukuoka T, Imura T, Yamamoto S, Kitagawa M, Sogabe A, Kitamoto D. Identification of Pseudozyma graminicola CBS 10092 as a producer of glycolipid biosurfactants, mannosylerythritol lipids. J. Oleo Sci. 2008;57:123–131.10.5650/jos.57.123
- Makimura K, Murayama SY, Yamaguchi H. Detection of a wide range of medically important fungi by the polymerase chain reaction. J. Med. Microbiol. 1994;40:358–364.10.1099/00222615-40-5-358
- Thompson JD, Higgins DG, Gibson TJ. CLUSTAL W: Improving the sensitivity of progressive multiple sequence alignment through sequence weighting, position-specific gap penalties and weight matrix choice. Nucleic. Acids. Res. 1994;22:4673–4680.10.1093/nar/22.22.4673
- Hokazono H, Omori T, Ono K. Effects of single and combined administration of fermented barley extract and gamma-aminobutyric acid on the development of atopic dermatitis in NC/Nga mice. Biosci. Biotechnol. Biochem. 2010;74:135–139.10.1271/bbb.90653
- Furuta Y, Maruoka N, Nakamura A, Omori T, Sonomoto K. Utilization of fermented barley extract obtained from a by-product of barley shochu for nisin production. J. Biosci. Bioeng. 2008;106:393–397.10.1263/jbb.106.393
- Barnett JA, Payne RW, Yarrow D. Yeasts: characteristics and identification. 3rd ed., Cambridge: Cambridge University Press; 2000.
- Trindade RC, Resende MA, Silva CM, Rosa CA. Yeasts associated with fresh and frozen pulps of Brazilian tropical fruits. System. Apppl. Microbiol. 2002;25:294–300.10.1078/0723-2020-00089
- Rau U, Nguyen LA, Schulz S, Wray V, Nimtz M, Roeper H, Koch H, Lang S. Formation and analysis of mannosylerythritol lipids secreted by Pseudozyma aphidis. Appl. Microbial. Biotechnol. 2005;66:551–559.10.1007/s00253-004-1672-9
- Morita T, Konishi M, Fukuoka T, Imura T, Kitamoto D. Discovery of Pseudozyma rugulosa NBRC 10877 as a novel producer of glycolipid biosurfactants, mannosylerythritol lipids, based on rDNA sequence. Appl. Microbiol. Biotechnol. 2006;73:305–313.10.1007/s00253-006-0466-7
- Morita T, Ogura Y, Takashima M, Hirose N, Fukuoka T, Imura T, Kondo Y, Kitamoto D. Isolation of Pseudozyma churashimaensis sp. nov., a novel ustilaginomycetous yeast species as a producer of glycolipid biosurfactants, mannosylerythritol lipids. J. Biosci. Bioeng. 2011;112:137–144.10.1016/j.jbiosc.2011.04.008
- Morita T, Konishi M, Fukuoka T, Imura T, Kitamoto HK, Kitamoto D. Characterization of the genus Pseudozyma by the formation of glycolipid biosurfactants, mannosylerythritol lipids. FEMS Yeast Res. 2007;7:286–292.10.1111/fyr.2007.7.issue-2
- Kitamoto HK, Shinozaki Y, Cao XH, Morita T, Konishi M, Tago K, Kajiwara H, Koitabashi M, Yoshida S, Watanabe T, Sameshima-Yamashita Y, Nakajima-Kambe T, Tsushima S. Phyllosphere yeasts rapidly break down biodegradable plastics. AMB Express. 2011;1:44. doi: 10.1186/2191-0855-1-44.10.1186/2191-0855-1-44