Abstract
The stable ascorbic acid (AA) derivative, 2-O-α-d-glucopyranosyl-l-ascorbic acid (AA-2G), exhibits vitamin C activity after enzymatic hydrolysis to AA. The biological activity of AA-2G per se has not been studied in detail, although AA-2G has been noted as a stable source for AA supply. The protective effect of AA-2G against the oxidative cell death of human dermal fibroblasts induced by incubating with 2,2′-azobis(2-amidinopropane) dihydrochloride (AAPH) for 24 h was investigated in this study. AA-2G showed a significant protective effect against the oxidative stress in a concentration-dependent manner. AA-2G did not exert a protective effect during the initial 12 h of incubation, but had a significant protective effect in the later part of the incubation period. Experiments using a α-glucosidase inhibitor and comparative experiments using a stereoisomer of AA-2G confirmed that AA-2G had a protective effect against AAPH-induced cytotoxicity without being converted to AA. Our results provide an insight into the efficacy of AA-2G as a biologically interesting antioxidant and suggest the practical use of AA-2G even before being converted into AA as a beneficial antioxidant.
Graphical Abstract
2-O-α-D-Glucopyranosyl-L-ascorbic acid without being converted to ascorbic acid protected against free radical-induced cytotoxicity in human dermal fibroblasts.
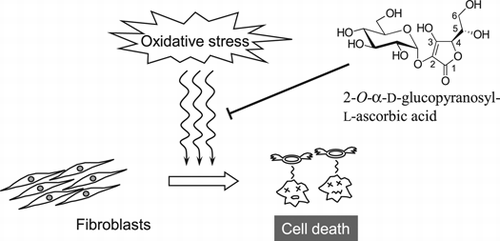
l-Ascorbic acid (AA, Fig. ), known as vitamin C, plays key roles in many biological processes as collagen formation, carnitine synthesis, and iron absorption.Citation1,2) It is also an important antioxidant in food and biological systems,Citation3) but AA is unstable under various oxidative conditions like exposure to neutral pH, heat, and heavy metals, resulting in rapid degradation.Citation4) Yamamoto et al. have developed the stable AA derivative, 2-O-α-d-glucopyranosyl-l-ascorbic acid (AA-2G, Fig. ), from AA and maltose or other α-glucans by regioselective transglucosylation with α-glucosidases from the digestive organs of mammals and rice seed and with cyclodextrin glucanotransferase.Citation5−Citation8) AA-2G has exhibited such in vitro and in vivo biological activities as an antiscorbutic effect,Citation9,10) collagen synthesis-enhancing effect,Citation11) melanin synthesis-inhibiting effect,Citation12) antibody production-enhancing effect,Citation13−Citation15) a promotive effect on B cell differentiation,Citation16) and antioxidative and antiaging effectsCitation17) after enzymatic hydrolysis to AA by α-glucosidase. AA-2G has been approved by the Japanese Government as a quasi-drug principal ingredient in skin care and as a food additive, and is now widely used as a medical additive in commercial cosmetics.
We have found that AA-2G without being converted to AA exerted radical-scavenging activity toward such unnatural model radicals as the 1,1-diphenyl-2-picrylhydrazyl (DPPH) radicalCitation18−Citation21) and 2,2′-azinobis(3-ethylbenzothiazoline-6-sulfonic acid) radical cation (ABTS•+).Citation20,22) The chemical property of AA-2G as a radical scavenger was greatly different from that of AA; i.e., the reaction rate with these model radicals of AA-2G was far slower than that of AA, but the long-lasting radical-scavenging ability per one molecule of AA-2G was superior to that of AA under optimal conditions. In order to resolve the question of whether these slow and long-lasting radical-scavenging reactions of AA-2G against unnatural model radicals would be physiologically relevant, we have further shown that AA-2G exhibited antioxidative activity in a cell-based antioxidative assay involving 2,2′-azobis(2-amidinopropane) dihydrochloride (AAPH)-induced erythrocyte hemolysis inhibition.Citation23) The oxidation of erythrocyte membranes serves as a good model to study peroxidative damage to biological membranes, because the erythrocyte membrane is rich in polyunsaturated fatty acids and erythrocytes are very susceptible to peroxidation.Citation24) Although the physiological antioxidative activity of AA-2G was found in the simple model of oxidative stress, AA-2G is not in contact with erythrocytes when it is orally administered. AA-2G administered orally could thus be hydrolyzed to AA by α-glucosidase on brush border membranes and absorbed as AA from the small intestine in guinea pigs.Citation25) AA-2G has been practically used as a skin-lightening cosmetic ingredient, and intact AA-2G has been shown to penetrate the skin.Citation26) The biological efficacy of AA-2G as a skin antioxidant without being converted to AA has therefore remained arguable.
We investigated in this present study the protective effect of AA-2G on AAPH-induced cytotoxicity in human dermal fibroblasts and compared it to the effects of AA and other 2-O-substituted AA derivatives. The time-course characteristics of the protective effect and stability of AA-2G were also investigated.
Materials and methods
Chemicals
AA-2G was presented by Hayashibara Biochemical Laboratories (Okayama, Japan). 2-O-β-d-Glucopyranosyl-l-ascorbic acid (AA-2βG) was isolated from Lycium fruit by a modification of a previous method.Citation27) l-Ascorbic acid (AA), AAPH, castanospermine, and a calcein-AM solution (1 mg/mL of DMSO) were purchased from Wako Pure Chemical Industries (Osaka, Japan). l-Ascorbic acid 2-phosphate sesquimagnesium salt (AA-2P) and l-ascorbic acid 2-sulfate dipotassium salt (AA-2S) were obtained from Sigma Chemicals (St. Louis, MO, USA). 6-Hydroxy-2,5,7,8-tetramethylchroman-2-carboxylic acid (Trolox) was obtained from Tokyo Kasei Kogyo (Tokyo, Japan). Dulbecco’s modified Eagle’s medium (DMEM) was purchased from Nissui Pharmaceuticals (Tokyo, Japan) or Sigma Chemicals. Penicillin G and streptomycin were obtained from Wako Pure Chemical Industries (Osaka, Japan) or Sigma Chemicals. Fetal bovine serum was from Gibco (Life Technologies, Carlsbad, CA, USA), SAFC Bioscience (Nichirei Biosciences, Tokyo, Japan) or HyClone (Logan, UT, USA). The reagents were used without further purification. All water used was of Milli-Q grade.
Oxidative cell death protection assay
Normal human dermal fibroblasts (CSC-2F0; Cell Systems, Kirkland, WA, USA) were cultured in DMEM supplemented with 10% fetal bovine serum, 100 U/mL of penicillin, and 100 μg/mL of streptomycin at 37 °C in a humidified atmosphere of 5% CO2 and 95% air. The cells were suspended in the medium at an initial density of 1 × 105 cells/mL. Each 100 μL of the cell suspension was seeded in a 96-well culture plate (Nunc, Roskilde, Denmark). After 24 h of incubation, 50 μL of AA or the AA derivative-containing medium and 50 μL of 15 or 60 mM (final concentration) AAPH were added. The skin fibroblasts were then cultured at 37 °C for the indicated times, washed three times with phosphate-buffered saline (PBS), and labeled with 100 μL of 5 μM calcein-AM in PBS. After 30 min with calcein-AM, the cells were lysed with the addition of 20 μL of Triton X-100 (0.6%, v/v) in PBS. The fluorescence from the calcein-positive viable cells was measured with excitation at 485 nm and emission at 527 nm, using a Fluoroskan Ascent instrument (Labosystems, Helsinki, Finland).
Stability of the antioxidants in a culture medium
The stability of the antioxidants was tested in DMEM supplemented with 10% fetal bovine serum, 100 U/mL of penicillin, and 100 μg/mL of streptomycin at 37 °C in a humidified atmosphere of 5% CO2 and 95% air. Each 100 μL of the medium was poured into a 96-well culture plate, and then 50 μL of 1 mM (final concentration) of the antioxidant-containing medium and 50 μL of 15 mM (final concentration) of AAPH were added. The resulting solution was stored at 37 °C for the indicated times, and 100-μL samples were periodically taken. The concentrations of the antioxidants were analyzed by HPLC after deproteinization. The HPLC analyses were carried out with a system consisting of a Hitachi L-2130 pump, L-2420 UV–Vis detector, L-2300 column oven, and D-2500 chromato-integrator (Hitachi High-Technologies, Tokyo, Japan), or with a system consisting of a Hitachi L-7100 pump, L-7420 UV–Vis detector, L-7300 column oven, and D-2500 chromato-integrator. AA and AA-2G were separated by isocratic elution in an Inertsil Diol column (ϕ 4.6 × 250 mm, 5 μm; GL Sciences, Tokyo, Japan) kept at 40 °C with 85% acetonitrile-66.7 mM ammonium acetate at a flow rate of 0.7 mL/min.Citation28) The absorbance at 260 nm was monitored. Trolox was separated by isocratic elution in an Inertsil Ph-3 column (ϕ 4.6 × 250 mm, 5 μm; GL Sciences) kept at 40 °C with 55% MeOH-H2O containing 0.2% formic acid at a flow rate of 0.7 mL/min. The absorbance at 290 nm was monitored.
Data analysis
Results are expressed as the mean and SD of three independent experiments. Multiple comparisons of the data were done by ANOVA followed by Dunnett’s t-test or Dunnett’s T3-test. p values less than 5% were regarded as significant.
Results and discussion
Protective effects of AA-2G, AA-2P, and AA-2S on oxidative-damaged fibroblasts
It has been found by using an AAPH-induced oxidative hemolysis inhibition assay that the radical-scavenging activityCitation20) of the 2-O-substituted ascorbic acid derivatives, ascorbic acid 2-glucoside (AA-2G), ascorbic acid 2-phosphate (AA-2P), and ascorbic acid 2-sulfate (AA-2S), was biologically relevant.Citation23) The chemical structures of AA, AA-2G, AA-2P, and AA-2S are shown in Fig. , the order of the inhibition effectiveness being AA-2S > AA-2P ≥ AA-2G = AA. Although the oxidation of erythrocyte membranes serves as a good model to study peroxidative damage to a biological membrane, the characteristics of the erythrocyte membrane with substantial unsaturated fatty acidCitation24) differ from those of the usual cell membrane.
The protective effect of AA-2G against the AAPH-induced cell death of human dermal fibroblasts was investigated and compared to the effects of AA and other 2-O-substituted AA derivatives (Fig. ). AA-2G, AA-2P, and AA-2S showed significant protective effects against oxidative damage to fibroblasts. AA-2G and AA-2P protected the cells against AAPH-induced cytotoxicity in a concentration-dependent manner. The order of the protective effectiveness was AA-2G ≥ AA-2P > AA-2S. This result is not in agreement with our previous result already described. This may be due to a different lipid constitution of the plasma membrane. Unexpectedly, AA did not show a significantly protective effect. On the other hand, in the absence of AAPH, AA and the 2-O-substituted AA derivatives showed no effect on cell viability at any of the concentrations tested.
Fig. 2. Cytoprotective effects of AA and the AA derivatives against AAPH-Induced oxidative stress.
Note: Plated cells (1.0 × 104 cells/well) were cultured for 24 h. The cells were then incubated with the indicated concentrations of AA and the AA derivatives in the presence and absence of AAPH (15 mM). After 24-h incubation, cell viability was determined by using calcein-AM. Data are presented as the mean of three independent experiments. Bars indicate SD. ##p < 0.01 (Dunnett’s T3-test). *p < 0.05, **p < 0.01, ***p < 0.001 (Dunnett’s T3-test) vs. AAPH alone.
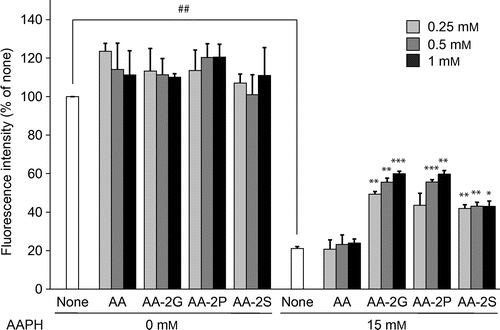
Taniguchi et al. have reported that AA-2G was enzymatically hydrolyzed into AA to give antioxidative and antiaging activities in human dermal fibroblasts.Citation17) AA-2GCitation9−Citation11) and AA-2PCitation29) have been shown to exert such an inherent biological effect as antiscorbutic activity, after their enzymatic hydrolysis to free AA in vitro and in vivo. On the other hand, AA-2SCitation30) did not have such activity, because it cannot be enzymatically converted into AA. It is therefore not clear whether or not AA-2G and AA-2P without being converted to AA protected against AAPH-induced cytotoxicity, but it is evident that AA-2S per se reduced the cytotoxicity.
Protective effect of AA-2G on oxidative-damaged fibroblasts without being converted to AA
To address the issue of whether AA-2G without being converted to AA could protect cells against AAPH-induced cytotoxicity, the effect of AA-2G was investigated in the presence of the α-glucosidase inhibitor, castanospermine, and its effect was compared to that of 2-O-β-d-glucopyranosyl-l-ascorbic acid (AA-2βG, Fig. ). Castanospermine has been reported to completely prevent AA-2G-induced stimulation of collagen synthesis after 24 h of cultureCitation11) and antibody production after 5 or 7 d of cultureCitation13,14,16) by inhibiting the release of AA. The protective effect of AA-2G on AAPH-induced cytotoxicity was not affected at all by the addition of castanospermine (Fig. ). Castanospermine also showed no influence on cell viability in the absence of AAPH. AA-2βG, a stereoisomer of AA-2G, has been isolated from Lycium fruit, a popular traditional Chinese food.Citation27) The level of intact AA-2βG as well as AA was increased in blood when AA-2βG was orally administered to rats,Citation27) while intact AA-2G was not present in the blood when AA-2G was orally administered to rats and guinea pigs,Citation9) suggesting that there is a lower distribution of β-glucosidase than that of α-glucosidase in tissues. AA-2βG as well as AA-2G protected the cells against AAPH-induced cytotoxicity in a concentration-dependent manner (Fig. ). No significant difference was apparent between the protective effects in the two groups. These results suggest that intact AA-2G exerted a protective effect on AAPH-induced cytotoxicity in human dermal fibroblasts, but that AA released from AA-2G by α-glucosidase did not.
Fig. 3. Effect of castanospermine, a neutral α-Glucosidase inhibitor, on the cytoprotective effect of AA-2G.
Note: Plated cells (1.0 × 104 cells/well) were cultured for 24 h. The cells were then incubated with or without AA-2G (1 mM), castanospermine (100 μM), and AAPH (15 mM). After 24-h incubation, cell viability was determined by using calcein-AM. Data are expressed as the mean of three independent experiments. Bars indicate SD. ##p < 0.01 (Dunnett’s T3-test). **p < 0.01 (Dunnett’s T3-test). n.s. not significant.
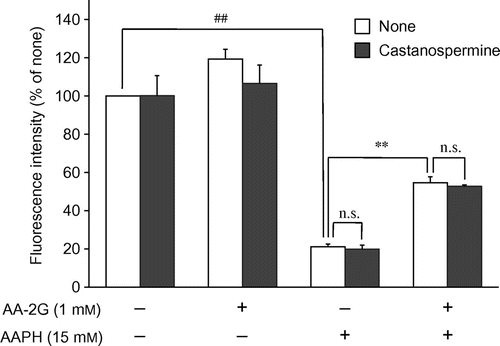
Fig. 4. Comparison of the cytoprotective effects of AA-2G and AA-2βG.
Note: Plated cells (1.0 × 104 cells/well) were cultured for 24 h. The cells were then incubated with the indicated concentrations of AA-2G, AA-2βG, and AAPH. After 24-h incubation, cell viability was determined by using calcein-AM. Data are presented as the mean of three independent experiments. Bars indicate SD. *p < 0.05, **p < 0.01, ***p < 0.001 (Dunnett’s T3-test) vs. AAPH alone. n.s. not significant.
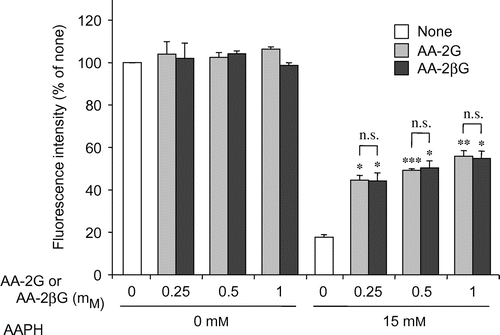
Time-course characteristics for the protective effects of AA-2G, AA, and Trolox on oxidative-damaged fibroblasts
AA-2G exerted a protective effect on AAPH-induced cytotoxicity after 24 h of treatment (Fig. ). However, under this experimental condition, AA, which is well known as an antioxidant, did not show a protective effect at all. AA as well as AA-2G has exhibited antioxidative activity in the AAPH-induced erythrocyte hemolysis inhibition assay with 3-h incubation.Citation23) It is thought that AA could not show an effect in the 24-h evaluation system due to its instability. The reaction time was then shortened from 24 to 4 h to examine whether a protective effect of AA could be seen with incubation for a short time. In order to clearly detect the cytotoxicity with a short exposure, 60 mM of AAPH was used. The viability of fibroblasts treated with 60 mM of AAPH was reduced by 30% in comparison to that of control fibroblasts (Fig. ). AA completely protected against the cytotoxicity, while AA-2G did not. These results suggest that the reactivity of AA-2G against AAPH-derived radicals was less than that of AA. It is therefore thought that AA-2G slowly and continuously reacted with radicals and that AA quickly reacted with radicals.
Fig. 5. Cytoprotective effects of AA and AA-2G against oxidative stress induced by the short AAPH treatment.
Note: Plated cells (1.0 × 104 cells/well) were cultured for 24 h. The cells were then incubated with the indicated concentrations of AA, AA-2G, and AAPH. After 4-h incubation, cell viability was determined by using calcein-AM. Data are presented as the mean of three independent experiments. Bars indicate SD. ##p < 0.01 (Dunnett’s T3-test). **p < 0.01 (Dunnett’s T3-test) vs. AAPH alone.
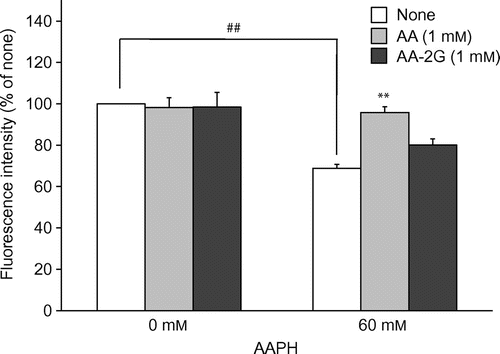
To evaluate the relationship between the protective effect against AAPH-induced cytotoxicity and the reactivity against AAPH-derived radicals, a time-course study of the protective effects of AA-2G and AA was carried out in the presence of 15 mM of AAPH (Fig. ). The protective effects of AA-2G and AA were also compared to those of Trolox (a water-soluble vitamin E analog, Fig. ). Slight protective effects of AA were observed until 8 h after the treatment, although these effects were not significant. AA did not protect against cytotoxicity after treating for 12 h. In contrast, AA-2G significantly protected the cells from cytotoxicity after treating for 16 h, although its protective effect was not apparent until after 12 h of treatment. These results are in agreement with those shown in Figs. and . On the other hand, Trolox showed its protective effects during the whole experimental period, although its effects were not significant by 4 h and 8 h. These results indicate that AA-2G, which slowly and continuously reacted with radicals, exerted its protective effect in the latter part of the experimental period, and that AA, which quickly reacted with radicals, showed its protective effect in the initial part of the experimental period.
Fig. 6. Time-course characteristics for the cytoprotective effects of AA, AA-2G, and Trolox against AAPH-Induced oxidative stress.
Note: Plated cells (1.0 × 104 cells/well) were cultured for 24 h. The cells were then incubated with the indicated concentrations of antioxidants and AAPH (15 mM). After 4, 8, 12, 16, and 20 h, the medium was replaced with a fresh one, and cell viability was determined after 24-h incubation by using calcein-AM. Data are expressed as the mean of three independent experiments. Bars indicate SD. ##p < 0.01 (Dunnett’s T3-test), **p < 0.01, ***p < 0.001 (Dunnett’s t-test) vs. the respective control (None).
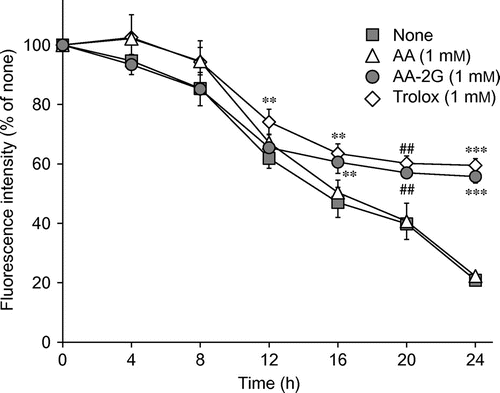
Finally, the stability of AA-2G, AA, and Trolox in the culture medium was investigated in the presence and absence of 15 mM of AAPH (Fig. ). AA-2G was very stable in the absence of AAPH, but gradually decreased with time in the presence of AAPH. The net decrease ratio of AA-2G, which was obtained by subtracting the remaining ratio of AA-2G (+) from that of AA-2G (−), was 34.3% after 24 h of incubation. Trolox slightly decreased in the absence of AAPH, but gradually decreased with time in the presence of AAPH. The net decrease ratio of Trolox was 51.7% after 24 h. The protective effect of AA-2G after 24 h was almost the same as that of Trolox (Fig. ), although a smaller amount of AA-2G had been consumed than that of Trolox (Fig. ). These results suggest that the protective effectiveness of AA-2G per one molecule was superior to that of Trolox. In contrast, AA rapidly decreased and disappeared within 8 h in both the presence and absence of AAPH, although the addition of AAPH slightly accelerated the consumption of AA. The instability of AA caused a loss in the protective effect against AAPH-induced cytotoxicity determined after 24 h of incubation. These results agree well with the results of our previous study, showing that AA-2G slowly and continuously reacted with DPPH radicals and ABTS•+, and that the number of radicals scavenged by one molecule of AA-2G was greater than that by AA and Trolox under appropriate conditions.Citation20) The results obtained in this study therefore indicate that intact AA-2G slowly and continuously scavenged the AAPH-derived radicals to protect dermal fibroblasts against oxidative stress.
Fig. 7. Stability of AA, AA-2G, and Trolox in the culture medium.
Note: AA (1 mM), AA-2G (1 mM), and Trolox (1 mM) dissolved in the culture medium were incubated in the presence (+) or absence (−) of AAPH (15 mM). After 2, 4, 8, 12, 16, 20, and 24 h, the remaining AA, AA-2G, and Trolox was determined by HPLC. Data are expressed as the mean of three independent experiments. Bars indicate SD.
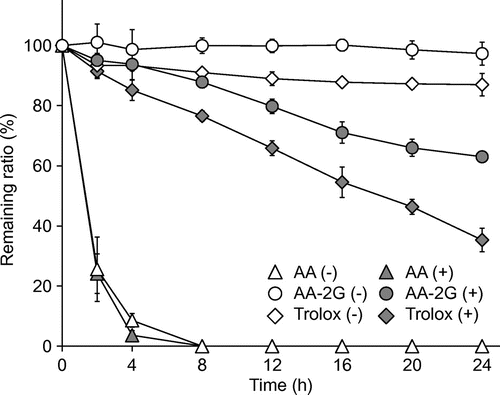
We found in this study that intact AA-2G exerted a protective effect on AAPH-induced cytotoxicity in human dermal fibroblasts incubated for 24 h, but that AA released from AA-2G by α-glucosidase did not. We have shown that AA-2G, which slowly and continuously reacted with radicals, exerted a protective effect in the latter part of the experimental period, and that AA, which quickly reacted with radicals, exerted its effect in only the initial part of the experimental period. It has been reported that AA-2G and AA-2P could protect the skin from UVB/A-induced photodamage ex vivo by themselves more effectively than could AA,Citation31) and that AA-2G could inhibit the formation of sunburn cells in UVB-irradiated human skin.Citation12) Hence, it may be necessary to consider the antioxidative activity of AA-2G per se not only in vitro but also in vivo. The results obtained in this study suggest that AA-2G acted as a moderately reactive antioxidant as well as AA which is metabolized from AA-2G as a highly reactive antioxidant in vivo. AA-2G is currently being used as a medical additive in commercial cosmetics and as a food additive in Japan. Our results provide insight into the efficacy of AA-2G per se as a biologically interesting antioxidant and suggest the practical value of AA-2G, even before being converted into AA in vivo, as a beneficial antioxidant in food and cosmetic applications.
Acknowledgments
We thank Hayashibara Biochemical Laboratories (Okayama, Japan) for the supply of AA-2G.
References
- Englard S, Seifter S. The biochemical functions of ascorbic acid. Annu. Rev. Nutr. 1986;6:365–406.10.1146/annurev.nu.06.070186.002053
- Naidu KA. Vitamin C in human health and disease is still a mystery? An overview. Nutr. J. 2003;2:7.10.1186/1475-2891-2-7
- Bendich A, Machlin LJ, Scandurra O, Burton GW, Wayner DDM. The antioxidant role of vitamin C. Adv. Free Radic. Biol. Med. 1986;2:419–444.10.1016/S8755-9668(86)80021-7
- Tolbert BM, Downing M, Carlson RW, Knight MK, Baker EM. Chemistry and metabolism of ascorbic acid and ascorbate sulfate. Ann. N. Y. Acad. Sci. 1975;258:48–69.10.1111/nyas.1975.258.issue-1
- Yamamoto I, Muto N, Nagata E, Nakamura T, Suzuki Y. Formation of a stable l-ascorbic acid α-glucoside by mammalian α-glucosidase-catalyzed transglucosylation. Biochim. Biophys. Acta. 1990;1035:44–50.10.1016/0304-4165(90)90171-R
- Yamamoto I, Muto N, Murakami K, Suga S, Yamaguchi H. l-Ascorbic acid α-glucoside formed by regioselective transglucosylation with rat intestinal and rice seed α-glucosidases: its improved stability and structure determination. Chem. Pharm. Bull. 1990;38:3020–3023.10.1248/cpb.38.3020
- Aga H, Yoneyama M, Sakai S, Yamamoto I. Synthesis of 2-O-α-d-glucopyranosyl l-ascorbic acid by cyclomaltodextrin glucanotransferase from Bacillus stearothermophilus. Agric. Biol. Chem. 1991;55:1751–1756.10.1271/bbb1961.55.1751
- Mandai T, Yoneyama M, Sakai S, Muto N, Yamamoto I. The crystal structure and physicochemical properties of l-ascorbic acid 2-glucoside. Carbohydr. Res. 1992;232:197–205.10.1016/0008-6215(92)80054-5
- Yamamoto I, Suga S, Mitoh Y, Tanaka M, Muto N. Antiscorbutic activity of l-ascorbic acid 2-glucoside and its availability as a vitamin C supplement in normal rats and guinea pigs. J. Pharmacobio-Dyn. 1990;13:688–695.10.1248/bpb1978.13.688
- Wakamiya H, Suzuki E, Yamamoto I, Akiba M, Otsuka M, Arakawa N. Vitamin C activity of 2-O-α-d-glucopyranosyl-l-ascorbic acid in guinea pigs. J. Nutr. Sci. Vitaminol. 1992;38:235–245.10.3177/jnsv.38.235
- Yamamoto I, Muto N, Murakami K, Akiyama J. Collagen synthesis in human skin fibroblasts is stimulated by a stable form of ascorbate, 2-O-α-d-glucopyranosyl-l-ascorbic acid. J. Nutr. 1992;122:871–877.
- Kumano Y, Sakamoto T, Egawa M, Iwai I, Tanaka M, Yamamoto I. In vitro and in vivo prolonged biological activities of novel vitamin C derivative, 2-O-α-d-glucopyranosyl-l-ascorbic acid (AA-2G), in cosmetic fields. J. Nutr. Sci. Vitaminol. 1998;44:345–359.10.1248/bpb.21.662
- Yamamoto I, Tanaka M, Muto N. Enhancement of in vitro antibody production of murine splenocytes by ascorbic acid 2-O-α-glucoside. Int. J. Immunopharmacol. 1993;15:319–325.10.1016/0192-0561(93)90042-W
- Tanaka M, Muto N, Gohda E, Yamamoto I. Enhancement by ascorbic acid 2-glucoside or repeated additions of ascorbate of mitogen-induced IgM and IgG productions by human peripheral blood lymphocytes. Jpn. J. Pharmacol. 1994;66:451–456.10.1254/jjp.66.451
- Mitsuzumi H, Kusamiya M, Kurimoto T, Yamamoto I. Requirement of cytokines for augmentation of the antigen-specific antibody responses by ascorbate in cultured murine T-cell-depleted splenocytes. Jpn. J. Pharmacol. 1998;78:169–179.10.1254/jjp.78.169
- Ichiyama K, Mitsuzumi H, Zhong M, Tai A, Tsuchioka A, Kawai S, Yamamoto I, Gohda E. Promotion of IL-4- and IL-5-dependent differentiation of anti-µ-primed B cells by ascorbic acid 2-glucoside. Immunol. Lett. 2009;122:219–226.10.1016/j.imlet.2009.01.007
- Taniguchi M, Arai N, Kohno K, Ushio S, Fukuda S. Anti-oxidative and anti-aging activities of 2-O-α-glucopyranosyl-l-ascorbic acid on human dermal fibroblasts. Eur. J. Pharmacol. 2012;674:126–131.10.1016/j.ejphar.2011.11.013
- Fujinami Y, Tai A, Yamamoto I. Radical scavenging activity against 1,1-diphenyl-2-picrylhydrazyl of ascorbic acid 2-glucoside (AA-2G) and 6-acyl-AA-2G. Chem. Pharm. Bull. 2001;49:642–644.10.1248/cpb.49.642
- Takebayashi J, Tai A, Yamamoto I. Long-term radical scavenging activity of AA-2G and 6-acyl-AA-2G against 1,1-diphenyl-2-picrylhydrazyl. Biol. Pharm. Bull. 2002;25:1503–1505.10.1248/bpb.25.1503
- Takebayashi J, Tai A, Gohda E, Yamamoto I. Characterization of the radical-scavenging reaction of 2-O-substituted ascorbic acid derivatives, AA-2G, AA-2P, and AA-2S: a kinetic and stoichiometric study. Biol. Pharm. Bull. 2006;29:766–771.10.1248/bpb.29.766
- Takebayashi J, Asano R, Nakae Y, Saito M, Gohda E, Yamamoto I, Tai A. 2-O-α-d-Glucopyranosyl-l-ascorbic acid scavenges 1,1-diphenyl-2-picrylhydrazyl radicals via a covalent adduct formation. Biosci. Biotechnol. Biochem. 2007;71:754–760.10.1271/bbb.60602
- Takebayashi J, Tai A, Yamamoto I. pH-dependent long-term radical scavenging activity of AA-2G and 6-Octa-AA-2G against 2,2′-azinobis(3-ethylbenzothiazoline-6-sulfonic acid) radical cation. Biol. Pharm. Bull. 2003;26:1368–1370.10.1248/bpb.26.1368
- Takebayashi J, Kaji H, Ichiyama K, Makino K, Gohda E, Yamamoto I, Tai A. Inhibition of free radical-induced erythrocyte hemolysis by 2-O-substituted ascorbic acid derivatives. Free Radic. Biol. Med. 2007;43:1156–1164.10.1016/j.freeradbiomed.2007.07.002
- Yamamoto Y, Niki E, Eguchi J, Kamiya Y, Shimasaki H. Oxidation of biological membranes and its inhibition. Free radical chain oxidation of erythrocyte ghost membranes by oxygen. Biochim. Biophys. Acta. 1985;819:29–36.10.1016/0005-2736(85)90192-0
- Wakamiya H, Suzuki E, Yamamoto I, Akiba M, Arakawa N. In situ intestinal absorption of 2-O-α-d-glucopyranosyl-l-ascorbic acid in guinea pigs. J. Nutr. Sci. Vitaminol. 1995;41:265–272.10.3177/jnsv.41.265
- Yamamoto I, Tai A, Fujinami Y, Sasaki K, Okazaki S. Synthesis and characterization of a series of novel monoacylated ascorbic acid derivatives, 6-O-acyl-2-O-α-d-glucopyranosyl-l-ascorbic acids, as skin antioxidants. J. Med. Chem. 2002;45:462–468.10.1021/jm010379f
- Toyoda-Ono Y, Maeda M, Nakao M, Yoshimura M, Sugiura-Tomimori N, Fukami H. 2-O-(β-d-Glucopyranosyl)ascorbic acid, a novel ascorbic acid analogue isolated from Lycium fruit. J. Agric. Food Chem. 2004;52:2092–2096.10.1021/jf035445w
- Tai A, Gohda E. Determination of ascorbic acid and its related compounds in foods and beverages by hydrophilic interaction liquid chromatography. J. Chromatogr. B. 2007;853:214–220.10.1016/j.jchromb.2007.03.024
- Machlin LJ, Garcia F, Kuenzig W, Brin M. Antiscorbutic activity of ascorbic acid phosphate in the rhesus monkey and the guinea pig. Am. J. Clin. Nutr. 1979;32:325–331.
- Machlin LJ, Garcia F, Kuenzig W, Richter CB, Spiegel HE, Brin M. Lack of antiscorbutic activity of ascorbate 2-sulfate in the rhesus monkey. Am. J. Clin. Nutr. 1976;29:825–831.
- Masatsuji-Kato E, Tsuzuki T, Kobayashi S. Reduction of UVB/A-generated free radicals by sodium l-ascorbyl-2-phosphate in cultured mouse skin. J. Health Sci. 2005;51:122–129.10.1248/jhs.51.122