Abstract
From the pellicle formed on top of brewing coconut water vinegar in Sri Lanka, three Acetobacter strains (SL13E-2, SL13E-3, and SL13E-4) that grow at 42 °C and four Gluconobacter strains (SL13-5, SL13-6, SL13-7, and SL13-8) grow at 37 °C were identified as Acetobacter pasteurianus and Gluconobacter frateurii, respectively. Acetic acid production by the isolated Acetobacter strains was examined. All three strains gave 4% acetic acid from 6% initial ethanol at 37 °C, and 2.5% acetic acid from 4% initial ethanol at 40 °C. Compared with the two other strains, SL13E-4 showed both slower growth and slower acetic acid production. As well as the thermotolerant SKU1108 strain, the activities of the alcohol dehydrogenase and the aldehyde dehydrogenase of SL13E-2 and SL13E-4 were more stable than those of the mesophilic strain. The isolated strains were used to produce coconut water vinegar at higher temperatures than typically used for vinegar production.
Graphical Abstract
From the pellicle formed on top of brewing coconut water vinegar in Sri Lanka, three Acetobacter pasteurianus strains and four Gluconobacter frateurii strains were isolated.
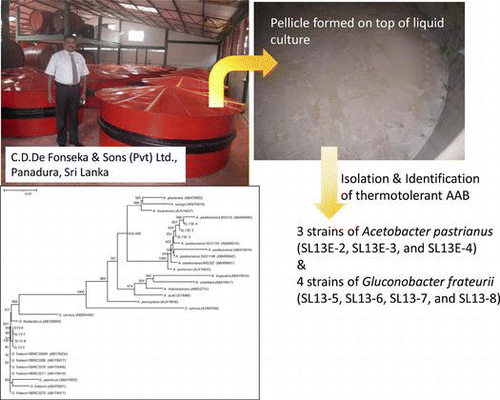
Vinegar made from fermented coconut water or the sap of coconut palm inflorescence is used extensively as a preservative and flavoring agent in pickles and many dishes in Sri Lanka. Naturally fermented sap collected from tapped tender inflorescence of the coconut palm, known as toddy in Sri Lanka, is a popular alcoholic beverage and is used as the major raw material in traditional vinegar fermentation in rural villages. Coconut water is a waste product from the production of copra and desiccated coconut and is generally discarded, but some is used as a raw material for industrial vinegar production. Like other kinds of vinegar, coconut vinegar is produced by a two-stage fermentation process, in which fermentable sugars are first converted into ethanol by yeasts, and then, the ethanol is oxidized to acetic acid by acetic acid bacteria (AAB).
During industrial coconut vinegar production, up to 10% (w/v) sugar is added to the matured coconut water, since raw coconut water contains only about 3% (w/v) sugar.Citation1) The fortified coconut water is then fermented in large vats with baker’s yeast (Saccharomyces cerevisiae) to an alcohol content of 5–8%. Alcohol fermentation is then stopped by removing the upper yeast layer, and the fermented coconut water is filtered and transferred to a new, aerated vat. After the addition of mother vinegar, the contents are fermented to a total acidity of 4.5% (w/v) and then pasteurized at 60 °C for 20 min. The pasteurized product is transferred to aging vats; after aging, it is filtered, bottled, and marketed.
AAB are an important group of bacteria that oxidize carbon sources rapidly and incompletely and hence are widely used in the commercial production of vinegar, d-gluconate, and l-sorbose. Recently, AAB were classified into 10 genera belonging to the family Acetobacteraceae.Citation2) Acetobacter and Gluconacetobacter are the primary species used in vinegar fermentation due to their strong ability to oxidize ethanol and to tolerate high acetic acid concentrations. They produce acetic acid from ethanol by two sequential oxidation reactions involving alcohol dehydrogenase (ADH) and aldehyde dehydrogenase (ALDH), which are localized on the outer surface of the cytoplasmic membrane.Citation3) ADH is a quinohemoprotein–cytochrome c complex consisting of three subunits with pyrroloquinoline quinone and heme c as the prosthetic groups. ALDH is also a cytochrome c complex consisting of three subunits with molybdenum–molybdopterin as the prosthetic group.
A group of microorganisms exists in nature that can grow at temperatures 5–10 °C higher than typical mesophilic strains of the same genus and sometimes even of the same species. These strains, called thermotolerant microbes, are different from thermophiles, which can grow above 60 °C and are in totally different genera. Thermotolerant strains may have acquired their growth phenotype by adapting to habitats characterized by higher temperatures, such as tropical regions. Thermotolerant AAB can produce acetic acid at temperatures of up to 40 °C. The fermentation efficiency of thermotolerant strains for vinegar production at 38–40 °C is similar to that of mesophilic strains at 30 °C, and they exhibit high acetate and ethanol tolerances.Citation4) Hence, thermotolerant AAB are useful for vinegar fermentation at higher temperatures, allowing a possible reduction in cooling expenses and other costs.
A description of the microbiology and biochemistry of the natural fermentation of coconut palm sap was published by Athputharajah et al.,Citation5) but the responsible AAB in Sri Lankan vinegar production is not known. Further, as Sri Lanka is a tropical country, presumably vinegar production would involve AAB that can grow and perform oxidative fermentation at higher temperatures. The aim of this study was to isolate, identify, and characterize thermotolerant AAB from Sri Lankan vinegar and to use these AAB for vinegar fermentation at higher temperatures.
Materials and methods
Sampling, bacterial strains, culture media, and culture conditions
Samples were collected from the pellicle formed on top of brewing vinegar in fermentation tanks at a coconut water vinegar factory in Panadura, Sri Lanka, in 2010, where vinegar is produced by natural fermentation.
Acetobacter pasteurianus SKU1108 (NBRC101655), isolated in Thailand (optimum growth temperature, approximately 37 °CCitation4)), was used as the thermotolerant reference strain.
Potato medium (1 g of yeast extract, 1 g of polypeptone, 2 g of glycerol, 0.5 g of glucose, and 10 mL of potato extract, made up to 100 mL with tap water) was used for stock cultures on agar slants and in pre-cultures. YPG medium (0.5 g of yeast extract, 0.5 g of polypeptone, 1.0 g of glycerol, 0.5 g of CaCO3, and 1.5 g of agar) supplemented with 4 mL ethanol and 3 mg of bromocresol purple per 100 mL was used in the isolation of Acetobacter-type strains. YPS/YPM medium (0.1 g of yeast extract, 0.1 g of polypeptone, 1 g of d-sorbitol or d-mannitol, and 1.5 g of agar) supplemented with 3 mg of bromocresol purple per 100 mL was used in the isolation of the Gluconobacter-type strains. YPGD medium (0.5 g of yeast extract, 0.5 g of polypeptone, 0.5 g of glycerol, and 0.5 g of glucose per 100 mL tap water, and 1.5 g of agar for the agar plates), supplemented with either ethanol or acetic acid, was used in studying ethanol and acetic acid tolerances and acetic acid production. Salt agar (0.02 g of (NH4)2HPO4, 0.01 g of MgSO4.7 H2O, 0.01 g of Ca5(PO4)3(OH), 0.2 mL of glacial acetic acid, and 1.5 g of agar, made up to 100 mL with tap water) was used to study the utilization of various carbon sources.Citation6)
Isolation of AAB
An inoculating loop-full of sample was streaked onto an YPG (Acetobacter-type strains) agar plate supplemented with 4% (v/v) ethanol and onto an YPS/YPM agar plate (Gluconobacter-type strains). Colonies with halos on the YPG agar plates containing ethanol, and colonies creating a circular yellow zone on the YPS/YPM agar plates, were selected as Acetobacter-type and Gluconobacter-type strains, respectively. Pure cultures were obtained by repeated streaking. The cultures were maintained on potato agar slants. Stock cultures of the isolated strains were prepared by mixing equal volumes of the culture and sterile glycerol and were frozen at −80 °C until use.
Growth and acetic acid production by AAB
The growth of the isolated Acetobacter and Gluconobacter strains was examined on potato agar plates at 30, 37, and 40 °C for 5 d. The acetic acid and ethanol tolerances of the identified Acetobacter strains were examined with YPGD agar plates supplemented with 1–4% (v/v) acetic acid or 1–10% (v/v) ethanol at 30, 37, 40, 41, and 42 °C for 5 d. Acetic acid production was examined with YPGD medium supplemented with 4 and 6% (v/v) ethanol at 30, 37, 38, and 40 °C under both static and shaking (200 rpm) culture conditions.
Production of coconut water vinegar
Simultaneous coconut vinegar production was examined statically at 37, 39, and 40 °C by inoculating 0.2 g of commercially obtained dry yeast and the isolated strains together and sequentially in coconut water whose Brix value had been adjusted to 10% (w/v) by the addition of glucose.
Phenotypic and biochemical characterization
Physiological and biochemical characterization of the isolated strains was done following Bergy’s Manual of Systematic BacteriologyCitation7) and related publications.Citation8–10) Acetobacter strains were identified by their carbon source utilization.Citation6)
Preparation of membrane fractions
Cells grown in YPGD medium with and without ethanol at 37 °C were harvested by centrifugation at 9,000 × g for 10 min at 4 °C and washed twice with ice-cold 0.1 M Tris–HCl (pH 8.0) and ice-cold distilled water, respectively. The washed cells were suspended on 10 mM KPB (pH 6.0) and passed twice by a French pressure cell press at 16, 000 psi. The intact cells were removed by centrifugation at 9,000 × g for 10 min at 4 °C. Then, the supernatant was ultracentrifuged at 90, 000 × g for 90 min at 4 °C to separate the membrane fractions from the soluble fraction. The membrane fractions were homogenized in 10 mM KPB (pH 6.0) and were used as sources for the enzyme assay.
Enzyme assay
ADH (EC 1.1.99.8) and ALDH (EC 1.2.99.3) activities were measured with ferricyanide as electron acceptor, as described by Ameyama.Citation11) One unit of enzyme activity was defined as the amount of enzyme catalyzing oxidation of 1 μmol of substrate per min at 25 °C with 100 mM substrate and 10 mM ferricyanide. Protein was measured by a modification of the Lowry method with bovine serum albumin as standard.Citation12)
Analytical method
The acidity of the culture medium was measured by titration with 0.8 N NaOH with phenolphthalein as pH indicator.
16S rRNA gene sequencing and phylogenetic analysis
For sequencing, 16S rDNA was amplified by PCR Go Taq Green Master Mix (Promega, Madison, WI). Cell lysate was used as template DNA without purification. Specific primers 27f (5′-AGA GTT TGA TCC TGG CAG-3′) and 1492r (5′-GGC TAC CTT GTT ACG ACT T-3′), selected from highly conserved regions of the nucleotide sequence of 16S rDNA of the proteobacteria, were used.Citation13) The PCR product was not obtained from SL13-7 with the 27f and 1492r set of primers, so another set of primers, Alf28f (5′-ARC GAA CGC TGG CGG CA-3′) and 1512uR (5′-ACG GHT ACC TTG TTA CGA CTT-3′),Citation14) was used. The amplified PCR products were purified with a LaboPass PCR product purification kit (Cosmo Genetech, Seoul, South Korea) and were sequenced at Sol Gent (Taejon, South Korea). The 16S rDNA nucleotide sequences were aligned and compared to the sequences of representative strains of Acetobacter and Gluconobacter obtained from GenBank (htts://www.ncbi.nlm.nih.gov/). Multiple alignments of the determined 16S rDNA sequences were performed by CLUSTALW.Citation15)
Phylogenetic analysis
For phylogenetic analysis of the isolated strains, the 16S rDNA gene sequences of A. pasteurianus, Gluconobacter frateurii stains, and related species were obtained from the NCBI website at http://www.ncbi.nlm.nih.gov. To construct the phylogenetic tree of isolated AAB, all the sequences were aligned by CLUSTALW. The Genetyx ver. 10 package was used to generate a phylogenetic tree to study the phylogenetic relationship based on the 16S rDNA gene by the neighbor-joining (NJ) approach and 1,000 bootstrap replicates.
Amplification and sequencing of the 16S–23S ITS region
PCR amplification of the 16S–23S rRNA gene internal transcribed spacer (ITS) region was done with primers ITS 1 (5′-TGC GGY TGG ATC ACC TCC T-3′, position 1,522–1,540 on the 16S rDNA, Escherichia coli numbering) and ITS 2 (5′-GTG CCW AGG CAT CCA CCG-3′, position 38-22 on the 23S rDNA, E. coli numbering).Citation16) The amplified PCR product (0.7 kb) was purified and cloned into pGEM-T™ EASY vector (Promega, Madison, WI) and transformed into competent E. coli DH5α cells. Recombinant plasmids with the 16S–23S ITS region were extracted from the cells with a LaboPass plasmid purification kit (Cosmo Genetech, Seoul, South Korea) and sequenced.
Nucleotide sequence accession numbers
The nucleotide sequence data reported here have been deposited in the DNA Data Bank of Japan under Accession Nos. AB753861 (SL13E-2), AB753862 (SL13E-3), AB753863 (SL13E-4), AB819116 (SL13-5), AB819117 (SL13-6), AB819118 (SL13-7), AB819119 (SL13-8) for 16S rDNA sequences, and AB754591 (SL13E-2), AB754592 (SL13E-3), AB754593 (SL13E-4), AB819120 (SL13-5), AB819121 (SL13-6), AB819122 (SL13-7), and AB819123 (SL13-8) for the 16S–23S ITS sequences.
Results
Identification and characterization of Acetobacter-type strains from Sri Lankan vinegar
By means of YPG medium containing ethanol, three Acetobacter-type AABs (SL13E-2, SL13E-3, and SL13E-4) producing different colony sizes were isolated from the pellicle formed in the fermenter tank. They were Gram-negative, rod-shaped, and catalase-positive. Since they oxidized acetate into CO2 and H2O, they were assigned to the genus Acetobacter (Table ).Citation7)
Table 1. Differential characteristics of Acetobacter strains isolated from Sri Lankan coconut vinegar.
For physiological characterization of the three Acetobacter strains, their carbon source utilization on salt agar medium was examined. The three isolated strains grew well on glycerol but poorly on d-glucose and d-mannitol. They did not grow on ethanol or on methanol, similarly to strains of A. pasteurianus, but differently from Acetobacter pomorum, which grows well on both methanol and ethanol.Citation6) As with A. pasterurianus,Citation17) all three isolates produced acid from d-glucose, d-fructose, d-galactose, d-mannose, d-xylose, and l-arabinose, and weakly from sucrose, but not from meso-erythritol.
The physiological and biochemical characteristics of the three Acetobacter strains are summarized in Table . The three isolates were very similar to A. pasteurianus.Citation17)
Next, the 16S rDNA from the three isolates was sequenced. According to a Blast search done on the GenBank database, all three Acetobacter strains have greater than 99% identity to several A. pasteurianus strains (e.g. SL13E-2, SL13E-3, and SL13E-4 have 100%, 99.85% (a 2-bp difference), and 99.92% (1-bp difference) identity to A. pasteurianus IFO3283, respectively). Thus, the three strains were confirmed to belong to A. pasteurianus. Fig. shows the phylogenetic tree and was in agreement that SL13E-2, SL13E-3, and SL13E-4 belonged definitely to the A. pasteurianus cluster. A comparison of the 16S rDNA sequence data among the three isolates indicated that SL13E-2 shows a 2-bp and a 1-bp difference from SL13E-3 and SL13E-4, respectively, while a 3-bp difference was seen between SL13E-3 and SL13E-4.
Fig. 1. Phylogenetic tree of isolated strains and related acetic acid bacteria.
Note: NJ phylogenetic tree calculated by CLUSTALW from 16S rDNA gene sequences of AAB. It was constructed by means of Genetyx ver.10.
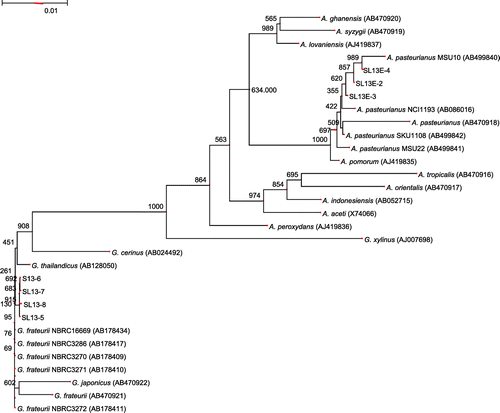
The 16S–23S ITS region of each strain was amplified and the nucleotide sequences (784 bp) were determined. The ITS sequence data for SL13E-2 and SL13E-3 were identical, while the ITS sequence of SL13E-4 was 4-bp different from those of SL13E-2 and SL13E-3.
Since these strains grew well on potato agar plates even at 42 °C, the acetic acid and ethanol tolerance of the three Acetobacter strains was examined on YPGD agar plates containing 1–4% (v/v) acetic acid or 1–10% (v/v) ethanol. Their tolerance was compared to that of a thermotolerant A. pasteurianus strain, SKU1108, isolated in Thailand.Citation4) Thermotolerant SKU1108, SL13E-2, SL13E-3, and SL13E-4, tolerated 1% (v/v) acetic acid at 42 °C on YPGD agar plates. All three SL13E strains tolerated up to 10% (v/v) ethanol at 37 °C and 8% ethanol at 41 °C, while SKU1108 grew in up to 10% ethanol at 41 °C. At 42 °C, SL13E-2, SL13E-3, and SL13E-4 tolerated only up to 3% (v/v) ethanol, while SKU1108 tolerated 4% (v/v) ethanol on YPGD agar plates. Thus, the three isolates obtained in this study were thermotolerant and highly tolerant of ethanol and acetic acid, comparably to SKU1108.
Identification and characterization of Gluconobacter-type strains from Sri Lankan vinegar
From the same samples, four Gluconobacter-type AABs (SL13-5, SL13-6, SL13-7, and SL13-8) showing different colony sizes were isolated by means of YPS (SL13-5 and SL13-7) and YPM (SL13-6 and SL13-8) media. These isolates showed no acetate oxidation.
In order to identify the species of the four isolates, their production of acid from pentitols (d-arabitol, l-arabitol, and d-ribitol) was examined. This characteristic is useful in discriminating among the three Gluconobacter species, Gluconobacter cerinus, G. frateurii, and Gluconobacter asaii.Citation18) G. frateurii produces acids from d-arabitol, l-arabitol, and d-ribitol, while G. cerinus produces acids from d-arabitol but not from l-arabitol or ribitol. G. oxydans does not produce acids from any of these pentitols.Citation18,19) Since all four isolates showed acid production from d-arabitol, l-arabitol, and d-ribitol, they were identified as G. frateurii.
For 16S rDNA sequencing, PCR products were obtained with 27f and 1492r as primers from isolates other than SL13-7. Another set of primers, Alf28f and 1512uR,Citation14) was used to obtain the PCR product for SL13-7. The 16S rDNA sequencing data for all four Gluconobacter isolates were identical except for 9 bp in the 5′-region of SL13-7. This 16S rDNA sequence was almost identical to those of G. frateurii (more than 99% identity, with a 3-bp difference from that of G. frateurii NBRC3264, for example). Phylogenetic analysis also indicated that these four strains belong to the G. frateurii cluster (Fig. ). Moreover, the ITS sequence data for SL13-5 and SL13-6 were identical with to SL13-7 and SL13-8, respectively, while a mere 1-bp difference was seen between SL13-5/SL13-7 and SL13-6/SL13-8. In sum, the four isolates were almost identical: the 16S rDNA and the ITS sequences of SL13-6 and SL13-8 were entirely identical and that of SL13-5 was different only in 1 bp from the other three. In the case of SL13-7, the 5’-region of the 16S rDNA sequence was slightly different from those of the other three isolates.
The growth of the four Gluconobacter isolates was examined on potato agar plates at various temperatures. All four strains showed good growth at up to 37 °C, similarly to the thermotolerant Gluconobacter strains isolated in Thailand previously.Citation19)
Acetic acid production by isolated Acetobacter strains
Acetic acid production by the three isolated Acetobacter strains was compared to that by the A. pasteurianus SKU1108 strain in YPGD medium with 4% (v/v) ethanol in shaking and static cultures at various temperatures (Fig. ). All the strains showed good growth at up to 40 °C (data not shown), although they showed different lag phases at 40 °C (SL13E-2, SL13E-3, and SL13E-4 for 2, 3, and 6 d, respectively). SL13E-4 showed slower growth and acetic acid production than the other strains. In shaking culture, as compared to the SL13E-4 strain, the acetic acid production of SL13E-2 and SL13E-3 was lower at 30 °C, showing a maximum at about 2% (w/v). On the other hand, SL13E-4 gave more than 3% (w/v) acetic acid production, comparably to the SKU1108 strain. At 37 °C, all three strains grew, and they oxidized ethanol more rapidly than at 30 °C and accumulated more than 3% (w/v) acetic acid in the culture medium. At 38 °C, all three isolates produced more than 3% (w/v) acetic acid, although they showed a very long lag phase, of 5 d, in their growth profiles, whereas SKU1108 showed a very short lag phase, less than 1 d. Even at 40 °C, SL13E-2 and SL13E-3 gave 3% (w/v) acetic acid production with a longer lag phase, whereas SL13E-4 grew very slowly (a lag phase of 6 d) and gave only 2.5% (w/v) acetic acid production. Thus, at all temperatures examined, the isolated strains produced significant amounts of acetic acid, but less than SKU1108, in both shaking and static culture.
Fig. 2. Comparison of acetic acid production with 4% ethanol.
Note: Acetic acid production was compared in shaking, 200 rpm (A), and static (B) cultures of SL13E-2, 13E-3, 13E-4, and SKU1108 at 30 °C (diamonds), 37 °C (squares), 38 °C (triangles), and 40 °C (crosses) in YPDG medium supplemented with 4% ethanol.
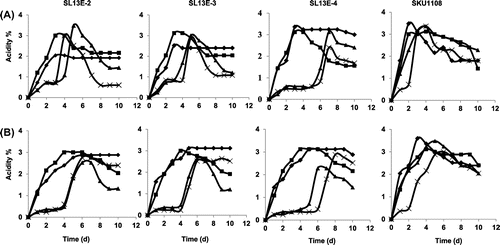
At all tested temperatures except for 30 °C in YPGD medium supplemented with 4% (v/v) ethanol, the three isolated strains and SKU1108 showed acetate oxidation, which is unfavorable for vinegar production. Consistently with these results, Saeki et al.Citation20) have reported that acetate oxidation was observed when the final concentration of acetic acid was less than 3.7% (w/v).
All three strains showed their best production of acetic acid at 37 °C in shaking and in static cultures when the initial ethanol concentration was 6% (v/v) (Fig. ). At 38 and 40 °C, even though it showed a long lag phase (6 d), SKU1108 showed significant acid production in a medium containing 6% (v/v) ethanol, whereas all three isolated strains showed very poor production (Fig. ) and growth (data not shown). All three strains yielded the highest acetic acid production (more than 4% w/v) at 37 °C in both shaking and static cultures (Fig. ). However, at 38 and 40 °C, none of the strains showed significant acetic acid production, while the SKU1108 strain gave more than 2% (w/v) and 3.5% (w/v) acetic acid production in shaking and static cultures, respectively. The isolated strains, especially SL13E-2 and SL13E-3, provided a higher concentration of acetic acid at 37 °C in shaking culture than SKU1108 did.
Fig. 3. Comparison of acetic acid production with 6% ethanol.
Note: Acetic acid production was compared in shaking, 200 rpm (A), and static (B) cultures of SL13E-2, 13E-3, 13E-4, and SKU1108 at 30 °C (diamonds), 37 °C (squares), 38 °C (triangles), and 40 °C (crosses) in YPDG medium supplemented with 6% ethanol.
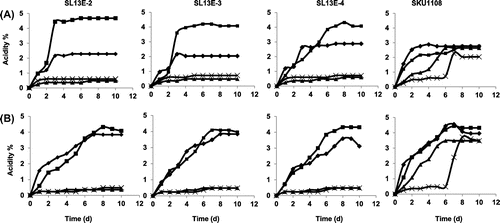
When the initial ethanol concentration was 6% (v/v), very little acetate oxidation by any of the four strains was observed at any temperature, even though in some cultures, the final acetic acid concentration was less than 3.7% (w/v) (Fig. ).
ADH and ALDH activities of the thermotolerant Acetobacter strains
ADH and ALDH activities in the membrane fractions were measured by means of thermotolerant SL13E-2, SL13E-4, and SKU1108 grown at 37 °C for 24 h with and without ethanol and were compared with mesophilic A. pasteurianus IFO3284. The results revealed that both the ADH and the ALDH activities of the thermotolerant strains were stronger than those of the mesophilic IFO3284 strain when the cells were grown at 37 °C (Fig. ), although the activities were variable among different batches of membrane fractions, and this is probably due to impaired growth at 37 °C. As shown in Fig. (A), by the ethanol to the culture medium, ADH activities of SL13E-2 and SL13E-4 increased about 3.2-fold and 2.3-fold, respectively, but not so greatly (1.2-fold) in the case SKU1108. But in the case of the mesophilic IFO3284 strain, no such induction of ADH activity was observed due to the addition of ethanol at 37 °C, which is beyond the optimum growth temperature. On the other hand, the ALDH activities of a given strain were unchanged by the addition of ethanol (Fig. (B)), except for SL13E-2, for which slight induction was observed (up to 1.5-fold). Thus, the enzyme activities of ADH were induced in response to the addition of ethanol although the induction levels varied among the strains, whereas ALDH activities were almost unchanged in all strains.
Fig. 4. ADH and ALDH activities in cells grown with and without ethanol.
Note: ADH (A) and ALDH (B) activities of the A. pasteurianus SL13E-2, SL13E-4, SKU1108, and IFO3284 strains grown at 37 °C without ethanol (white bars) and with ethanol (ash bars) in the culture medium. Assays were performed in triplicate. Bars indicate averages, and the error indicates standard deviation.
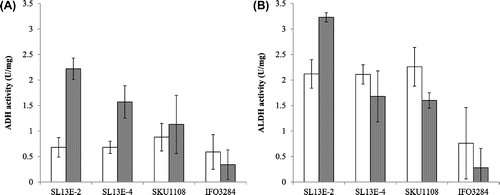
In addition, the thermostability of the ADH and ALDH of thermotolerant SL13E-2, SL13E-4, and SKU1108 was examined by incubation of the membrane fractions at 50, 55, and 60 °C. As shown in Fig. , as well as the thermotolerant SKU1108 strain, SL13E-2 and SL13E-4 showed almost the same thermal denaturation profiles for both ADH and ALDH. The inactivation of ALDH by thermal treatment was slightly slower than that of ADH. Thus, ALDH in the isolated strains was also more thermostable than ADH, as previously reported.Citation4,21,22) All three strains accounted for more than 50% of remaining ADH and ALDH activities after incubation at 50 °C for 30 min. Both the ADH and the ALDH of the three strains became unstable over 55 °C, and rapidly inactivated at 60 °C, as in the case of SKU1108. Similar results were found for the membrane fraction obtained from the cells grown on the medium in the presence of ethanol (data not shown).
Fig. 5. Thermal stabilities of ADH and ALDH activities in membrane fractions.
Note: Remaining ADH (A) and ALDH (B) activities of A. pasteurianus SL13E-2 (diamonds), SL13E-4 (squares), and SKU1108 (triangles) were assayed after incubation of the membrane fractions for 5–30 min at 50 °C (black), 55 °C (white), and 60 °C (ash).
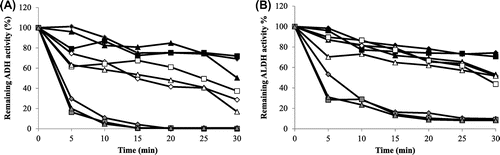
Production of coconut water vinegar
Simultaneous vinegar production by the isolated strains in coconut water with baker`s yeast was examined without shaking at 37, 39, and 40 °C (Fig. ). When both yeast and Acetobacter strains were added, higher acid production was obtained than when the isolates were added after 1 d of cultivation with baker’s yeast alone (Fig. ). At 37 °C, all three isolated strains provided 4% acetic acid production, as SKU1108 did. Although they showed acetate oxidation in YPGD medium with 4% (v/v) ethanol (Fig. ), acetate oxidation was not observed in coconut vinegar production (Fig. (A)). Both SL13E-2 and SL13E-3 provided about 3% (w/v) acetic acid production at 39 °C and 2.5% (w/v) at 40 °C, slightly better than SKU1108. When a mixed culture of the three isolates was used, acid production similar to SL13E-2 or SL13E-3 was observed (data not shown).
Fig. 6. Simultaneous coconut vinegar production.
Note: Acetic acid production was monitored in coconut water, the Brix value of which had been adjusted to 10% (w/v) by the addition of glucose with SL13E-2 (diamonds), SL13E-3 (squares), SL13E-4 (triangles), and SKU1108 (crosses) at 37 °C, 39 °C, and 40 °C. Dry yeast and AAB were added simultaneously (A) or sequentially (B). Arrows show points of addition of AAB in sequential addition.
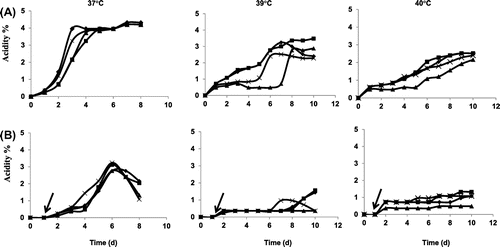
Discussion
In this study, we isolated, identified, and characterized thermotolerant AAB from Sri Lankan coconut water vinegar. The fermentation capacities of the three Acetobacter strains at higher temperatures were compared with that of a thermotolerant A. pasteurianus strain, SKU1108, isolated in Thailand previously.Citation4)
The three Acetobacter-type isolates (SL13E-2, SL13E-3, and SL13E-4) grew as well at 42 °C as the SKU1108 strain on potato agar plates and tolerated up to 1% (w/v) acetic acid and 8% (v/v) ethanol in YPGD agar medium. The results obtained were similar to those published for other thermotolerant AAB.Citation21,23) On the other hand, mesophilic A. pasteurianus IFO3191 did not grow on potato agar plates at 39 °C even in the absence of ethanol and of acetic acid, while A. pasteurianus IFO3284 grew on a potato agar plate at 39 °C but not at 41 °C. It tolerated 1% acetic acid at 39 °C and only 4% ethanol at 37 °C.Citation23) Since SL13E-2, SL13E-3, and SL13E-4 grew well at up to 42 °C and tolerated acetic acid and ethanol at elevated temperatures, they are considered thermotolerant AAB. Similarly, SL13-5, SL13-6, SL13-7, and SL13-8 are also considered thermotolerant Gluconobacter AABs, because although the mesophilic type strain, G. frateurii IFO3276, does not grow at 37 °C, these strains do grow, like the thermotolerant isolates from Thailand.Citation19) Even though our strains tolerated up to 8% ethanol at 41 °C on agar plates, they did not show acetic acid production at temperatures higher than 38 °C with 6% (v/v) ethanol in liquid culture (Fig. ). This might have been due to higher stress caused by increased temperature and lower oxygen availability in the liquid culture.
According to Bergy’s Manual of Systematic Bacteriology,Citation7) all Acetobacter species can use acetic acid as carbon source. This so-called over-oxidation is unfavorable for vinegar production. According to Saeki et al.Citation20) over-oxidation does not occur when oxidizable ethanol and other carbon sources remain in the culture medium, and no apparent over-oxidation occurs when the acetic acid concentration rises above 4.5% (w/v). For many strains, the threshold acetic acid concentration to overcome over-oxidation is about 3.7% (w/v). Thus, in general, over-oxidation can be avoided by terminating vinegar fermentation when a small amount of ethanol remains in the vinegar mash, or by allowing accumulation of more than 4% (w/v) acetic acid in the vinegar mash. In coconut vinegar production, over-oxidation of acetic acid is a frequent problem, since a lower acetic acid concentration is achieved (about 3% w/v) than in rice vinegar production (about 5% w/v). Our results showed significant over-oxidation for all four strains tested at an initial ethanol concentration of 4% (v/v), especially at higher temperatures (Fig. ), but at a 6% (v/v) initial ethanol level, no over-oxidation was observed (Fig. ) even though acetic acid was produced at less than 3.7% (w/v). These results indicate that the strains isolated in this study are suitable for the production of coconut water vinegar.
In acetic acid fermentation, ethanol is converted to acetic acid by two membrane-bound dehydrogenases, ADH and ALDH. Hence, we also examined the ethanol induction pattern and thermal resistance of the ADH and the ALDH of the isolated strains and compared them with thermotolerant SKU1108. According to our results, the ADH activity of SL13E-2 and SL13E-4 grown at 37 °C was induced by the addition of ethanol by 3.2-fold and 2.3-fold, respectively. A study by Takemura et al.Citation24) reported that the ADH activity of A. pasteurianus NCI1380 grown at 30 °C was enhanced more than 10-fold by the addition of ethanol to the medium.
The ADHs from all four thermotolerant strains became unstable at above 55 °C and became inactive at 60 °C irrespective of the addition of ethanol to the culture medium (Fig. ). Kanchanarach et al.Citation23) reported that ADH from thermotolerant A. pasteurianus strains were stable at up to 45 °C for 30 min, but become unstable at above 50 °C and were rapidly inactivated at 55 °C. Thus, the ADHs from SL13E-2 and SL13E-4 were slightly more heat stable than the ADH from thermotolerant A. pasteurianus MSU10. According to Adachi et al.,Citation22) the ALDH from mesophilic strains showed high thermostability, and hence, thermostability of ADH is more important for vinegar production at higher temperatures. The ALDH activities of the isolated strains were more thermostable against heating than the ADH activities, and more than 30% of the original ALDH activity remained after 10 min of heating at 60 °CCitation4,21,22) (Fig. ).
The potential use of the three Acetobacter-type strains for coconut vinegar production at higher temperatures was examined. We tested two procedures: simultaneous addition of yeast and AAB, and sequential addition of AAB after yeast was cultivated alone for 1 d at an ethanol concentration of about 4% (v/v) (data not shown). As shown in Fig. , simultaneous addition gave better results than sequential addition, up to 4% (w/v) and 2.5% (v/v) acetic acid at 37 and 40 °C, respectively, in the cases of SL13E-2 and SL13E-3. This is slightly higher than the concentrations produced with SKU1108 (Fig. ). In sequential addition, within the first day of incubation, the yeast grew and produced ethanol rapidly by consuming most of the available glucose (data not shown). This led to poor glucose availability for AAB at the time they were introduced to the medium. All four AAB strains required glucose for better growth. Thus, the poor availability of glucose in the medium caused poor growth and acetic acid production in sequential vinegar production. No over-oxidation was observed during coconut water vinegar production within the periods examined, except when sequential addition at 37 °C was attempted (Fig. ). These results are inconsistent those shown in Fig. , probably due to the differences in carbon sources in the medium. For over-oxidation, complete exhaustion of ethanol and the availability of another carbon source that supplies oxaloacetate to convert acetyl-CoA to citrate are required.Citation20) In coconut water vinegar production, glucose and sucrose were carbon sources, and they were almost completely consumed by yeast and AAB, but, as in Fig. , glucose and glycerol were carbon sources, and probably some amount of glycerol remained even after the ethanol was completely oxidized to acetic acid.
Our results indicate that as well as the thermotolerant A. pasteurianus SKU1108 strain, these Acetobacter-type strains can be used for coconut water vinegar production at higher temperatures and can be used as starter cultures in the vinegar industry.
Supplemental material
The supplemental material for this paper is available at http://dx.doi.org/10.1080/09168451.2014.882758.
Supplementary Fig. 1
Download MS Power Point (547.5 KB)Supplementary Fig. 1 caption
Download MS Word (19 KB)Acknowledgment
We wish to thank C.D. De Fonseka & Sons (Pvt) Ltd., Panadura, Sri Lanka, for providing coconut vinegar samples. We are grateful to Professor Kazunobu Matsushita of Yamaguchi University for providing A. pasteurianus SKU1108.
Notes
Abbreviations: AAB, acetic acid bacteria; ADH, alcohol dehydrogenase; ALDH, aldehyde dehydrogenase; ITS, internal transcribed spacer; TCA, tricarboxylic acid; v/v, volume/volume; w/v, weight/volume.
References
- Jean WH, Yong LG, Yan FN, Swee NT. Molecules. 2009;14:5144–5164.
- Yamada Y, Yukphan P. Int. J. Food Microbiol. 2008;125:15–24.10.1016/j.ijfoodmicro.2007.11.077
- Adachi O, Moonmangmee D, Toyama H, Yamada M, Shinagawa E, Matsushita K. Appl. Microbiol. Biotechnol. 2003;60:643–653.10.1007/s00253-002-1155-9
- Saeki A, Theeragool G, Matsushita K, Toyama H, Lotong N, Adachi O. Biosci. Biotechnol. Biochem. 1997;61:138–145.
- Atputharajah JD, Widanapathirana S, Samarajeewa U. Food Microbiol. 1986;3:273–280.10.1016/0740-0020(86)90009-2
- Sokollek SJ, Hertel C, Hammes WP. Int. J. Syst. Bacteriol. 1998;48:935–940.10.1099/00207713-48-3-935
- Brenner DJ, Krieg NR, Staley JT. In: Garrity GM, editor. Bergy’s Manual of Systematic Bacteriology. vol. 2. New York, NY: Springer-Verlag; 2005. p. 51–54.
- Asai T, Iizuka H, Komagata K. J. Gen. Appl. Microbiol. 1964;10:95–126.10.2323/jgam.10.95
- Yamada Y, Okada Y, Kondo K. J. Gen. Appl. Microbiol. 1976;22:237–245.10.2323/jgam.22.237
- Katsura K, Yamada Y, Uchimura T, Komagata K. Int. J. Syst. Evol. Microbiol. 2002;52:1635–1640.10.1099/ijs.0.02093-0
- Ameyama M. In: Wood WA, editor. Methods in enzymology. vol. 89. New York, NY: Academic Press; 1982. p. 20–29.
- Dulley JR, Grieve PA. Anal. Biochem. 1975;64:136–141.10.1016/0003-2697(75)90415-7
- Hiraishi A. Lett. Appl. Microbiol. 1992;15:210–213.10.1111/j.1472-765X.1992.tb00765.x
- Mühling M, Woolven-Allen J, Murrell JC, Joint I. ISME J. 2008;2:379–392.10.1038/ismej.2007.97
- Thompson JD, Higgins DG, Gibson TG. Nucleic Acids Res. 1994;22:4643–4680.
- Tanasupawat S, Kommanee J, Yukphan P, Nakagawa Y, Yamada Y. J. Sci. Food Agric. 2011;91:2652–2659.10.1002/jsfa.v91.14
- Tanasupawat S, Kommanee J, Malimas T, Yukphan P, Nakagawa Y, Yamada Y. Microb. Environ. 2009;24:135–143.10.1264/jsme2.ME08564
- Yamada Y, Hosono R, Lisdyanti P, Widyastuti Y, Saono S, Uchimura T, Komagata K. J. Gen. Appl. Microbiol. 1999;45:23–28.10.2323/jgam.45.23
- Moonmangmee D, Adachi O, Ano Y, Shinagawa E, Toyama H, Theeragool G, Lotong N, Matsushita K. Biosci. Biotechnol. Biochem. 2000;64:2306–2315.10.1271/bbb.64.2306
- Saeki A, Taniguchi M, Matsushita K, Toyama H, Theeragool G, Lotong N, Adachi O. Biosci. Biotechnol. Biochem. 1997;61:317–323.10.1271/bbb.61.317
- Ndoye B, Lebecque S, Dubois-Dauphin R, Tounkara L, Guiro A-T, Kere C, Diawara B, Thonart P. Enzyme Microb. Technol. 2006;39:916–923.10.1016/j.enzmictec.2006.01.020
- Adachi O, Shinagawa E, Matsushita K, Ameyama M. Agric. Biol. Chem. 1988;52:2083–2084.10.1271/bbb1961.52.2083
- Kanchanarach W, Theeragool G, Yakushi T, Toyama H, Adachi O, Matsushita K. Appl. Microbiol. Biotechnol. 2010;85:741–751.10.1007/s00253-009-2203-5
- Takemura H, Kondo K, Horinouchi S, Beppu T. J. Bacteriol. 1993;175:6857–6866.