Abstract
Although previous reports have suggested that pectin induces morphological changes of the small intestine in vivo, the molecular mechanisms have not been elucidated. As heparan sulfate plays important roles in development of the small intestine, to verify the involvement of heparan sulfate (HS) in the pectin-induced morphological changes of the small intestine, the effects of pectin from Prunus domestica L. on cell-surface HS were investigated using differentiated Caco-2 cells. Disaccharide compositional analysis revealed that sulfated structures of HS were markedly changed by pectin administration. Real-time RT-PCR showed that pectin upregulated human HS 6-O-endosulfatase-2 (HSulf-2) expression and markedly inhibited HSulf-1 expression. Furthermore, inhibition analysis suggested that pretreatment with fibronectin III1C fragment, RGD peptide, and ERK1/2 inhibitor suppressed pectin-induced HSulf-2 expression. These observations indicate that pectin induced the expression of HSulf-2 through the interaction with fibronectin, α5β1 integrin, and ERK1/2, thereby regulating the sulfated structure of HS on differentiated Caco-2 cells.
Graphical Abstract
Pectin induced HSulf-2 expression through the interaction with fibronectin, α5β1 integrin, and ERK1/2, thereby changing sulfated structure of HS on differentiated Caco-2 cells.
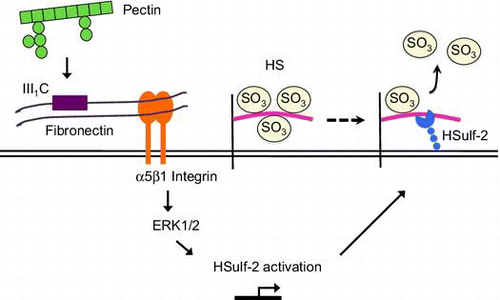
Pectin is a group of complex polysaccharides present in plant cell walls and is a component of dietary fibers that have generally been defined as the portion of edible plants that are resistant to digestion in the human stomach and small intestine.Citation1) Pectin is composed of various fragments of linear and ramified regions. The most abundant pectic polysaccharide is homogalacturonan, a linear homopolymer of α-1,4-linked d-galacturonic acid residues that comprises about 65% of pectin, with the remainder composed of rhamnogalacturonan-I and substituted galacturonans, such as rhamnogalacturonan-II.Citation2) Pectin has various physiological effects as dietary fiber for human health. Pectin reduces plasma and liver cholesterol levels,Citation3) prevents hepatic metastasis,Citation4) inhibits allergic responses,Citation5) exhibits anticancer activities,Citation2,6) and decreases the risks of intestinal infection and diarrhea in children.Citation7)
Heparan sulfate (HS) is a sulfated polysaccharide that is ubiquitously present on the cell surface and in the extracellular matrix of all animal cells. The backbone of HS is synthesized by polymerization of alternating d-glucuronic acid and N-acetyl-d-glucosamine residues in the Golgi apparatus. Once the backbone is synthesized, HS is randomly sulfated at the 2-O position of uronic acid and 6-O, 3-O, and N positions of glucosamine residues by multiple enzymes: HS 2-O-sulfotransferase-1 (2-OST-1), HS 6-O-sulfotransferases (6-OSTs), HS 3-O-sulfotransferases (3-OSTs), and HS N-deacetylase/N-sulfotransferases (NDSTs).Citation8) After HS is transported to the cell surface, HS 6-O-endosulfatases (Sulf1 and Sulf2) modify the sulfated structure of HS at the cell surface.Citation9,10) As these enzymes have multiple isoforms, except for 2-OST-1, they generate enormous structural diversity of HS. Based on the sulfated structure synthesized by the enzymes, HS plays essential roles in various physiological activities as a receptor or co-receptor for different ligands, such as Wnt, fibroblast growth factors, and transforming growth factor-β.Citation11,12)
Although many physiological effects of pectin as a dietary fiber are mediated by colonic microflora,Citation13) direct interaction of pectin with the small intestine has also been suggested. Several studies have shown that pectin caused morphological changes and promoted crypt branching in the small intestine.Citation14–16) However, the mechanism underlying the pectin-induced morphological changes in the small intestine is unknown. As HS participates in many physiological activities,Citation11) we hypothesized that cell-surface HS may be affected by pectin when it interacts with the small intestine. Recently, the involvement of cell-surface HS on normal development of the small intestine has been suggested.Citation17,18) To address the molecular mechanisms of underlying possible pectin–small intestine interactions, we focused on cell-surface HS and the effects of pectin on the sulfated structure of cell-surface HS on differentiated Caco-2 cells, which are often used as an intestinal epithelial cell model, and the mechanisms of pectin-induced structural changes of HS were investigated in this study.
Materials and methods
Materials
Prunes, Prunus domestica L. cv. d’Agen, imported from USA as material for a commercial prune extract (concentrated prune juice), were supplied by MIKI Corporation (Hyogo, Japan). Heparinase, heparitinase I, heparitinase II, and chondroitinase ABC were obtained from Seikagaku Biobusiness (Tokyo, Japan). The following unsaturated disaccharides from HS were purchased from Seikagaku Biobusiness: 2-acetoamido-2-deoxy-4-O-(4-deoxy-α-l-threo-hex-enopyranosyluronic acid)-d-glucose (ΔDi-0S), 2-deoxy-2-sulfamino-4-O-(4-deoxy-α-l-threo-hex-4-enopyranosyluronic acid)-d-glucose (ΔDi-NS), 2-acetoamido-2-deoxy-4-O-(4-deoxy-α-l-threo-hex-4-enopyranosyluronic acid)-6-O-sulfo-d-glucose (ΔDi-6S), 2-deoxy-2-sulfamino-(4-deoxy-α-l-threo-hex-4-enopyranosyluronic acid)-6-O-sulfo-d-glucose (ΔDi-diS1), 2-deoxy-2-sulfamino-(4-deoxy-2-O-sulfo-α-l-threo-hex-4-enopyranosyluronic acid)-d-glucose (ΔDi-diS2), and 2-deoxy-2-sulfamino-(4-deoxy-2-O-sulfo-α-l-threo-hex-4-enopyranosyluronic acid)-6-O-sulfo-d-glucose (ΔDi-triS). Trypsin/EDTA solution was from Nacalai Tesque (Kyoto, Japan). Anti-α5 antibody (ab150361) was from Abcam (Bristol, UK). PD98059 was from Wako Pure Chemical Industries (Osaka, Japan). FR180204 was from Calbiochem (San Diego, CA).
Purification of pectin from prunes
The concentrated prune juice was mixed with 99% ethanol so that the final concentration became 80%, and alcohol-insoluble solid (AIS) was obtained by vacuum filtration. After dehydration, AIS dissolved in distilled water was fractionated with a DEAE-cellulose (HCO3− form) column (4.6 × 20 cm). The column was eluted by stepwise elution of distilled water, 0.2 M NaHCO3, 0.3 M NaHCO3, 0.35 M NaHCO3, 0.4 M NaHCO3, and 0.1 M NaOH at a flow rate of 2.0 mL/min. The obtained fractions were combined based on the uronic acid content quantified using the carbazole reaction method.Citation19) The relevant fractions were collected, concentrated using a rotary evaporator at 40 °C, dialyzed against distilled water, and lyophilized.
Cell culture
Caco-2 cells were purchased from American Type Culture Collection (Manassas, VA). Passage 70–85 Caco-2 cells were cultured at 37 °C with 5% CO2 in Dulbecco’s Modified Eagle’s Medium (Nissui Pharmaceuticals, Tokyo, Japan) supplemented with 10% fetal bovine serum (FBS), 1% minimal essential medium non-essential amino acids, 80 units/mL of penicillin, 80 μg/mL of streptomycin, 0.06% of l-glutamine, and 0.19% of NaHCO3.
To conduct transepithelial electrical resistance (TEER) experiments, Caco-2 cells were seeded in 30 mm i.d. Transwell® inserts (polycarbonate membrane, 0.45 μm pore size; Merck Millipore, Billerica, MA) in 6-well plates at a density of 2.0 × 106 cells/well. The basolateral and apical compartments each contained 2.0 mL of culture medium. Culture medium was replaced every two days. The TEER was measured with a Millicell electrical resistance system (Merck Millipore) every 4 d.
Structural analysis of HS by high-performance liquid chromatography (HPLC)
HS on the cell surface of Caco-2 cells was prepared from 10-cm2 dishes at a density of 1.0 × 106 cells/flask. After cultivation for more than 20 d, the medium was changed to that containing 0.1 mg/mL pectin and incubated for 24 h at 37 °C with 5% CO2. Monolayers of the cells were extensively washed with phosphate-buffered saline (PBS) and then incubated in 2 mL of trypsin/EDTA at room temperature for 30 min, followed by 37 °C for 10 min.Citation20) The cell suspension was collected and the dish was rinsed with cold PBS. Cells were removed by centrifugation at 1500 × g for 10 min at 4 °C. The β-elimination treatment was not performed because the amount of HS collected from the cells with and without β-elimination was not changed (data not shown). Glycosaminoglycans were purified by phenol extraction and ethanol precipitation. Chondroitin sulfate was removed by digestion with a mixture of 40 milliunits of chondroitinase ABC in 240 μL of 33 mm Tris-HCl buffer (pH 8.0, containing 33 mm calcium acetate, and 20 ng of bovine serum albumin) at 37 °C for 16 h. HS was purified by phenol extraction and ethanol precipitation, and dissolved in water to make a sample solution of HS.
To investigate the structure of sulfated HS, the disaccharide composition enzymatically derived from HS on the cell surface was analyzed by HPLC with a UV detection system as described by Yoshida et al. with slight modifications.Citation21) The digests of HS were dried with a centrifugal concentrator (CC-105; TOMY, Tokyo, Japan) and dissolved in 10 μL of 16 mm sodium dihydrogen phosphate. Aliquots of 10 μL of disaccharide mixtures were injected onto a 4.6 × 250 mm YMC-Pack PA-G column (YMC Co., Ltd., Kyoto, Japan). The column was equilibrated with buffer A consisting of 16 mm sodium dihydrogen phosphate at a flow rate of 1.0 mL/min. Buffer B consisted of 538 mm sodium dihydrogen phosphate. After injection of samples, buffer B was increased from 0 to 100% in a linear gradient between 0 and 45 min with PU-2089 Plus (JASCO Corp., Tokyo, Japan). Each disaccharide was detected at 232 nm with JASCO UV-2075 Plus. The chromatograms were analyzed with Chromato PRO software (Runtime Instrument Corp., Sagamihara, Japan). The elution time of each disaccharide was determined using standard disaccharides.
Analysis of the expression of mRNA encoding HSulfs, 6-OSTs, and 2-OST-1 by RT-PCR and real-time RT-PCR
Total RNA from differentiated Caco-2 cells was prepared with RNAiso Plus (Takara Bio, Otsu, Japan) according to the manufacturer’s instructions. Aliquots of 1 μg of total RNA were treated with RNase-free DNase I (Toyobo, Osaka, Japan) and reverse-transcribed with oligo(dT)20 primer (Toyobo) and ReverTra Ace (Toyobo). The sequences of the primers used were as follows:
For HSulf-1 (266 bp), 5′-CCATGCTCACTGGGAAGTACGTG-3′ (F), 5′-CTTCTCCTTGATGCCGTTGCGA-3′ (R); for HSulf-2 (419 bp), 5′-AGTGGGTCGGCCTACTTAAGAACTC-3′ (F), 5′-ATAGATCGTCTCCATGGAGTCA-3′ (R); for 6-OST-1 (225 bp), 5′-CGCCCAGAAAGTTCTACTACATC-3′ (F), 5′-GGTTGTTAGCCAGGTTATAGGG-3′ (R); for 6-OST-2 (197 bp), 5′-ACCTCTTTCTGCAAAGGTATCAG-3′ (F), 5′-GTTCTGATTTGGGTTAGGATTTG-3′ (R); for 6-OST-3 (190 bp), 5′-GGACATGCAGCTTTATGAGTATG-3′ (F), 5′-GCTGTTGTAGTCCTCAGTGACAG-3′ (R); for 2-OST-1 (198 bp), 5′-TTTTCCTGGCTAAACGTTGG-3′ (F), 5′-TGAAGGCAGAAGAGGCAAAT-3′ (R), and for glyceraldehyde-3-phosphate dehydrogenase (GAPDH, 457 bp), 5′-CATCACCATCTTCCAGGAGC-3′ (F), 5′-GTTCAGCTCTGGGATGACCT-3′ (R). PCR was carried out with KOD-Plus-Neo (Toyobo) in a final volume of 15 μL containing 0.2 μm of each primer, PCR buffer for KOD-plus-Neo, 1 mm MgSO4, 200 μm dNTP, and 0.15 U of enzyme for 35 cycles of denaturing at 98 °C for 10 s, annealing at 62 °C for 15 s, and extension at 72 °C for 5 s.
To investigate the effects of pectin on each mRNA expression, the cells in 12-well plates were incubated with 0.1 mg/mL pectin for 1 h. After the medium was replaced with fresh medium, the cells were incubated for several hours. Relative expression of each mRNA was analyzed by real-time RT-PCR using THUNDERBIRD SYBR qPCR Mix (Toyobo) with ABI Step One Plus (Applied Biosystems, Foster City, CA). Real-time RT-PCR was performed with hot start at 95 °C for 60 s, followed by 40 cycles of denaturing for 15 s at 95 °C, annealing for 15 s at 62 °C, and extension for 20 s at 72 °C.
Effects of fibronectin and RGD peptide on HSulf-2 mRNA expression
Differentiated Caco-2 cells were incubated with 0.1 mg/mL pectin containing 0.8 μm fibronectin fragment III1-C (F3542; Sigma-Aldrich, St. Louis, MO) or RGD peptide (Sigma-Aldrich) for 1 h. After exchanging to fresh medium, the cells were incubated for 6 h. Relative expression level of HSulf-2 mRNA was detected by real-time RT-PCR as described above.
Analysis of α5β1 integrin expression in Caco-2 cells by Western blotting
To investigate the expression of α5β1 integrin in differentiated Caco-2 cells, its expression was analyzed by Western blotting. Differentiated Caco-2 cells cultured in 10-cm2 dishes at a cell density of 6.0 × 105 cells/dish were washed with ice-cold PBS, homogenized in cold cell lysis buffer containing 25 mM Tris-HCl, pH 7.4, 150 mM NaCl, 1 mM EDTA, 1% Triton X-100, 5% glycerol, and Protease Inhibitor Cocktail (Nacalai Tesque), and then centrifuged at low speed (800 × g, 10 min, 4 °C). The supernatant was centrifuged (20000 × g, 60 min, 4 °C) and collected. Protein concentration was measured using a BCA Protein Assay Kit (Thermo Fisher Scientific Inc., Rockford, IL). Samples were mixed with an equal volume of SDS sample buffer containing 62.5 mM Tris (pH 6.8), 2% SDS, 10% glycerol, 0.72 M 2-mercaptoethanol, and 0.1% bromophenol blue, and then boiled for 3 min. Samples were separated by electrophoresis with 12% polyacrylamide gel and transferred onto Immobilon-P transfer membranes (Merck Millipore). Membranes were blocked with 1% bovine serum albumin (Roche Applied Science, Indianapolis, IN) in Tris-buffered saline (pH 7.4) containing 0.1% Tween 20 (TBST). Protein bands of α5β1 integrin were detected by enhanced chemiluminescence (Immobilon Western Chemiluminescent HRP Substrate; Merck Millipore), using a primary antibody to α5β1 integrin (ab150361; Abcam) and anti-rabbit horseradish peroxidase secondary antibody (GE Healthcare UK Ltd., Little Chalfont, UK).
Effects of ERK1/2 inhibitor on HSulf-2 mRNA expression
Differentiated Caco-2 cells cultured in 12-well plates were preincubated with 30 μm FR180204 for 2 h. The cells were incubated with 0.1 mg/mL pectin for 1 h. Then, the medium was replaced with fresh medium and incubated for 6 h. Relative expression of HSulf-2 mRNA level was detected by real-time RT-PCR as described above.
Analysis of phosphorylated ERK1/2 with the Phos-tag SDS-PAGE system
Differentiated Caco-2 cells cultured in 12-well plates at a density of 4.0 × 104 cells/well were incubated with 0.1 mg/mL pectin for 0, 2, 5, 10, or 30 min. Moreover, the cells were incubated with 50 μm PD98059 for 90 min, and then 0.1 mg/mL pectin was administered for 5 min. The cells were incubated with ice-cold cell lysis buffer containing 25 mM Tris-HCl (pH 7.4), 150 mM NaCl, 1 mM EDTA, 1% Triton X-100, 5% glycerol, and Protease Inhibitor Cocktail for 5 min on ice. The cells were homogenized on ice and centrifuged (14000 × g, 10 min, 4 °C). Supernatant was collected and its protein concentration was determined using a BCA Protein Assay Kit. Samples were mixed with 4 × SDS sample buffer containing 250 mM Tris, pH 6.8, 8% SDS, 20% sucrose, 2.86 M 2-mercaptoethanol, and 0.01% bromophenol blue, and then boiled for 3 min. Phos-tag SDS-PAGE was conducted as described by Kinoshita and Kinoshita-Kikuta with slight modifications.Citation22) Briefly, the gel consisted of a separating gel (12% polyacrylamide and 357 mM bis-Tris-HCl, pH 6.8) and a stacking gel (4% polyacrylamide and 357 mM bis-Tris-HCl, pH 6.8). The acrylamide-pendant Phos-tag ligand (Wako) and two equivalents of Zn(NO3)2 were added to the separating gel before polymerization, and the final concentrations of Phos-tag ligand and Zn(NO3)2 were adjusted to 20 and 40 μm, respectively. The running buffer consisted of 0.10 M Tris, 0.10 M MOPS, 0.10% SDS, and 5.0 mM sodium bisulfite. Electrophoresis was performed at room temperature for 2 h at a constant flow of 30 mA. After electrophoresis, the gel was soaked in a solution containing 25 mM Tris, 192 mM glycine, 10% methanol, and 1.0 mM EDTA for 10 min to remove the Zn2+; this procedure was repeated three times, followed by incubation in a solution containing 25 mM Tris, 192 mM glycine, and 10% methanol for 10 min. Samples were transferred onto Immobilon-P transfer membranes. The transferred samples were immunoblotted with anti-ERK1/2 antibody (137F5; Cell Signaling Technology Inc., Danvers, MA) at 1:300 dilution in TBST containing 0.2% BSA, and then with ECL peroxidase-labeled anti-rabbit IgG secondary antibody at 1:3000 dilution in TBST containing 0.2% BSA. Bound antibodies were detected with enhanced chemiluminescence (Immobilon Western Chemiluminescent HRP Substrate; Merck Millipore) according to the manufacturer’s instructions. As a control, the expression of β-actin was detected by conventional Western blotting analysis with anti-actin antibody (sc-8432; Santa Cruz Biotechnology, Dallas, TX).
Statistical analyses
Differences were evaluated by Tukey’s test. The percentage data in Fig. were converted to arcsin values and then statistically tested. Results are expressed as means ± standard deviation (SD) and p < 0.05 was taken to indicate statistical significance.
Fig. 1. Alteration of disaccharide composition of HS at the surface of differentiated Caco-2 cells after pectin administration.
Notes: The disaccharide composition of HS on the cell surface of differentiated Caco-2 cells was compared between the cells with and without pectin treatment. The disaccharide mixtures of HS were analyzed by HPLC with a UV detection system. The values are shown as means ± SD of three independent experiments. After the percentage data were converted to arcsin values, statistical analyses were performed by Tukey’s test. *p < 0.05, **p < 0.01.
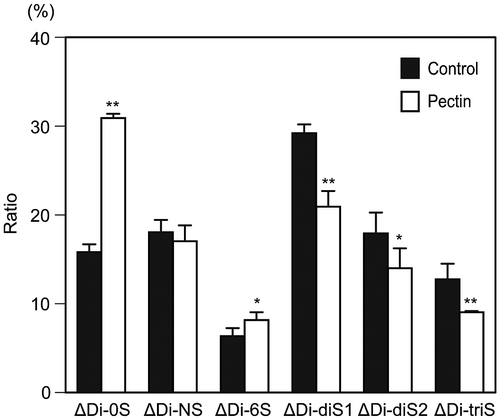
Results
Disaccharide composition of HS on the cell surface after pectin stimulation
AIS of prune, P. domestica L., was separated by anion-exchange chromatography and the main peak of uronic acid was obtained from the elution of 0.3 M NaHCO3 (data not shown). This fraction was used as pectin in this study. Caco-2 cells grown on polycarbonate membranes showed a gradual increase in TEER, with maximal TEER reached at about day 20 of culture (data not shown). The cells cultured for more than 20 d were used as differentiated Caco-2 cells.
To investigate the effects of pectin on the sulfated structure of cell-surface HS, we incubated the differentiated Caco-2 cells with or without pectin for 24 h and compared the disaccharide composition of HS (Fig. ). The amount of HS at 24 h after pectin administration was unaffected (data not shown). Disaccharides of the HS collected from cells without pectin administration (control) were composed of non-sulfated disaccharides (∆Di-0S, 16%), N-sulfated disaccharides (∆Di-NS, 18%), N- and 6-O-disulfated disaccharides (∆Di-diS1, 29%), N- and 2-O-disulfated disaccharides (∆Di-diS2, 18%), and N-, 6-, and 2-O-trisulfated disaccharides (∆Di-triS, 13%). On the other hand, disaccharide compositions of HS collected from cells with pectin administration were markedly changed: the ratio of ∆Di-0S was increased to 31%, that of ∆Di-diS1 was decreased to 21%, that of ∆Di-diS2 was decreased to 14%, and that of ∆Di-triS was decreased to 9%. This result indicated a change in sulfated structure of cell-surface HS in response to pectin.
Expression of HSulf-1/2 mRNA in differentiated Caco-2 cells
The pectin-induced percentage reduction of ∆Di-diS1 and ∆Di-triS suggested the participation of HSulf-1/2, which removes 6-O-sulfate from the UA2S-GlcNS6S trisulfated disaccharide unit and UA-GlcNS6S disulfated disaccharide unit.Citation9,10) Therefore, the mRNA expression of HSulf-1/2 in differentiated Caco-2 cells was investigated by RT-PCR. We found that both HSulf-1 and HSulf-2 were expressed in the cells (Fig. (A)). Furthermore, the level of HSulf-1 mRNA expression was markedly downregulated, whereas that of HSulf-2 was increased at 3 h after pectin treatment (Fig. (A)). Quantitative RT-PCR confirmed that HSulf-2 mRNA level was upregulated by pectin administration (Fig. (B)).
Fig. 2. Effects of pectin on the mRNA expression of HSulf-1 and HSulf-2 in the differentiated Caco-2 cells.
Notes: (A) Differentiated Caco-2 cells were incubated with 0.1 mg/mL pectin for 1 h, and then incubated with normal medium for 3, 9, 18, and 24 h. Total RNA was collected from the differentiated Caco-2 cells and the mRNA expression levels of HSulf-1 and HSulf-2 were analyzed by RT-PCR. The mRNA expression of the cells without pectin administration was shown as 0 h. (B) HSulf-2 mRNA expression of differentiated Caco-2 cells was quantitatively determined by real-time PCR and normalized relative to GAPDH expression. The values are shown as means ± SD of at least three independent experiments. Statistical analyses were performed by Tukey’s test. **p < 0.01.
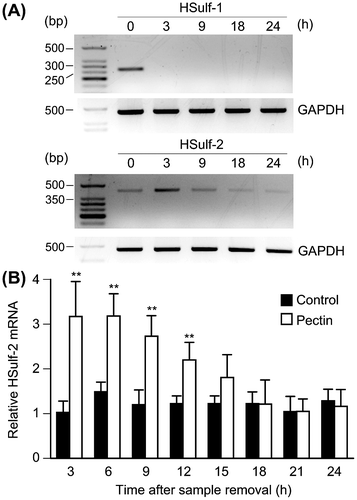
Time course of changes in 6-OSTs and 2-OST-1 mRNA levels after pectin administration
The effects of pectin treatment on mRNA expression of 6-OSTs and 2-OST-1 were investigated because Lamanna et al. reported that knockout of Sulfs affected the expression of 6-OSTs and 2-OST-1Citation9) and we found HSulf-1 expression was markedly inhibited by pectin administration. Differentiated Caco-2 cells were incubated with 0.1 mg/mL pectin for 1 h followed by incubation with normal medium for several hours. The relative expression of each enzyme was investigated by quantitative RT-PCR. Although the expression of 6-OST-2 was unaffected, that of 6-OST-1 was increased and that of 2-OST-1 was decreased by pectin treatment (Fig. ). Among the isoforms of 6-OSTs, 6-OST-3 was not expressed in differentiated Caco-2 cells (data not shown). Real-time RT-PCR analysis could not be carried out for the N-deacetylase/N-sulfotransferases, because these enzymes are regulated by internal ribosomal entry sites and not at the transcriptional level.Citation23) These data suggested that cell-surface HS changed the sulfated structure by regulating the expression of HS-modifying enzymes in response to pectin.
Fig. 3. Effects of pectin on 6-OSTs and 2-OST-1 mRNA expression level in the differentiated Caco-2 Cells.
Notes: Differentiated Caco-2 cells were incubated with 0.1 mg/mL pectin for 1 h, and then incubated with normal medium for the indicated times. The time courses of changes in 6-OST-1, 6-OST-2, and 2-OST-1 mRNA expression were quantitatively determined by real-time RT-PCR and normalized relative to GAPDH expression. The values are shown as means ± SD of at least three independent experiments. Each value was compared with each control and statistical analyses were performed by Tukey’s test. *p < 0.05, **p < 0.01.
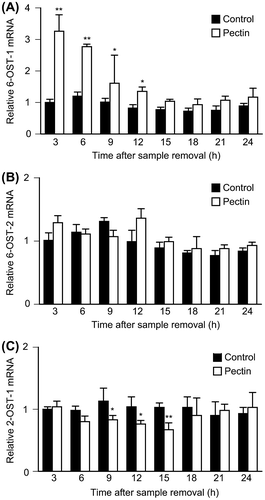
Elucidation of the mechanism by which pectin stimulates HSulf-2 mRNA expression
The sulfated structure of cell-surface HS and the mRNA expression of HS-modifying enzymes were changed by pectin administration, suggesting that the differentiated Caco-2 cells recognized pectin. Although receptors for pectins were not identified, Nagel et al. have shown that enzymatically modified pectins interacted with fibronectin,Citation24) which mediates a wide variety of cellular interactions in the extracellular matrix and plays a central role in cell adhesion, migration, and differentiation.Citation25) We have obtained preliminary results indicating that the C-terminal fragment containing two thirds of the III1 domain of fibronectin (FNIII1C) was bound to pectin of P. domestica L. (unpublished results). Furthermore, Nagel et al. reported that α5β1 and/or αvβ3 integrins, which are receptors for fibronectin,Citation26) play essential roles in fibronectin-dependent cell spreading of fibroblast cells.Citation24) Therefore, we hypothesized that pectin stimulated HSulf-2 expression by binding to fibronectin on α5β1 integrin in the differentiated Caco-2 cells. To verify the involvement of fibronectin, the effect of FNIII1C on the pectin-induced upregulation of HSulf-2 mRNA expression was investigated. As a result, premixing pectin with FNIII1C inhibited the upregulation of HSulf-2 mRNA level at 6 h after pectin stimulation (Fig. (A)). This result suggested that pectin stimulated HSulf-2 expression by binding to fibronectin. Furthermore, to verify whether α5β1 integrins participate in the pathway of pectin stimulation, the effect of RGD peptide on pectin-induced upregulation of HSulf-2 mRNA expression was investigated. As shown in Fig. (B), RGD peptide suppressed the increase in HSulf-2 expression by pectin. These results indicated that HSulf-2 mRNA expression was stimulated by pectin, which bound to fibronectin on α5β1 integrin in differentiated Caco-2 cells.
Fig. 4. Effects of FNIII1C and RGD peptides on HSulf-2 mRNA expression level in the differentiated Caco-2 cells.
Notes: (A) Differentiated Caco-2 cells were stimulated by 0.1 mg/mL pectin with or without FNIII1C peptide for 1 h. After the medium was exchanged, cells were incubated for 6 h. Relative mRNA expression of HSulf-2 was measured by real-time RT-PCR. (B) Differentiated Caco-2 cells were stimulated by 0.1 mg/mL pectin with or without RGD peptide for 1 h. After the medium was exchanged, cells were incubated for 6 h. Relative mRNA expression of HSulf-2 was measured by real time RT-PCR. The values are shown as means ± SD of three independent experiments. Statistical analyses were performed by Tukey’s test. **p < 0.01. (C) The expression of α5 integrin in differentiated Caco-2 cells was detected by Western blotting analysis.
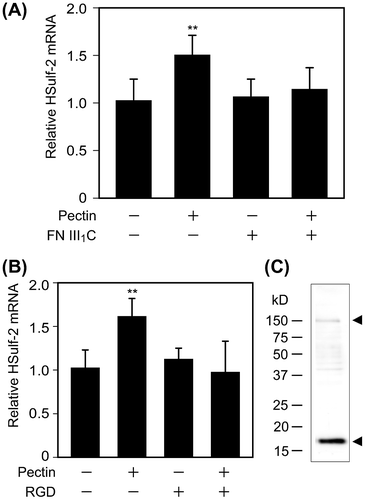
Investigation of α5 integrin in differentiated Caco-2 cells
As the expression of α5 integrin subunits in the Caco-2 cells differ among reports,Citation27–30) we investigated whether α5 integrin was expressed in differentiated Caco-2 cells by Western blotting. Whole lysates of differentiated Caco-2 cells were immunoblotted with α5 integrin antibody. Observed bands at 150 kDa and 19 kDa suggested that differentiated Caco-2 cells possess α5 integrin (Fig. (C)). As the expression of β1 integrin subunit in Caco-2 cells was reported previously,Citation31) we concluded that α5β1 integrins are expressed in the differentiated Caco-2 cells used in this study.
Identification of signal transduction pathways activating HSulf-2 expression
Integrins mediate cell adhesion to extracellular matrix proteins and regulate a number of intracellular signaling events, such as stimulation of the mitogen-activated protein kinase (MAPK) ERK1/2.Citation32) A previous study showed that α5β1 integrin mediates fibronectin-dependent cell proliferation of Caco-2 cells through ERK1/2 activation.Citation28) Therefore, we investigated whether pectin activates ERK1/2. Differentiated Caco-2 cells on 12-well plates were incubated with 0.1 mg/mL pectin for 0, 2, 5, 10, or 30 min. Phos-tag Western blotting analysis confirmed that ERK1/2 was phosphorylated by pectin treatment (Fig. (A)). The level of active ERK1/2 peaked at 5 min and then decreased over 30 min. Furthermore, pretreatment with 50 μm PD98059, an inhibitor of MEK activation, inhibited the pectin-dependent phosphorylation of ERK1/2 (Fig. (B)), suggesting that pectin activated ERK1/2 in differentiated Caco-2 cells. The participation of ERK1/2 in pectin-induced upregulation of HSulf-2 was then investigated. Differentiated Caco-2 cells on 12-well plates were preincubated with or without 30 μm FR180204, an inhibitor of ERK1/2, for 2 h, and then 0.1 mg/mL pectin was administered for 1 h. The pectin-induced upregulation of HSulf-2 mRNA expression was inhibited by FR180204 (Fig. (C)), indicating that pectin stimulated the transcription of HSulf-2 via ERK1/2 in differentiated Caco-2 cells.
Fig. 5. Effects of pectin on the activation of ERK1/2 and ERK1/2 inhibitor on HSulf-2 mRNA expression level in the differentiated Caco-2 cells.
Notes: (A) Differentiated Caco-2 cells were incubated with 0.1 mg/mL pectin for 0, 2, 5, 10, or 30 min. The time course of changes in pectin-induced phosphorylation levels of ERK1/2 were analyzed by Phos-tag Western blotting analysis. (B) Differentiated Caco-2 cells were preincubated with 50 μm PD98059 and then incubated with 0.1 mg/mL pectin for 0 or 5 min. Phosphorylation levels of ERK1/2 were analyzed by Phos-tag Western blotting analysis. (C) Differentiated Caco-2 cells were pretreated with FR180204 for 2 h followed by incubation with 0.1 mg/mL pectin for 1 h. After the medium was exchanged, the cells were incubated for 6 h. Relative mRNA levels of HSulf-2 were measured. The values are shown as means ± SD of three independent experiments. Statistical analyses were performed by Tukey’s test. **p < 0.01.
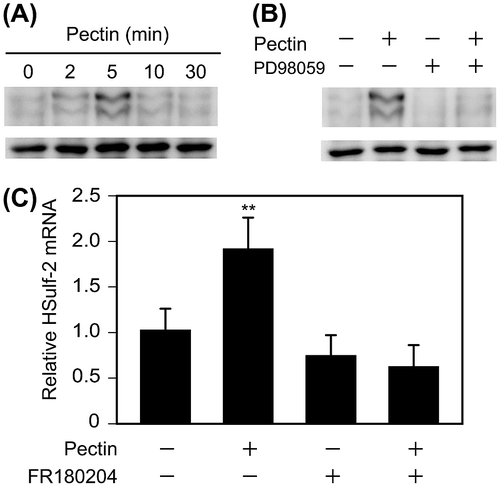
Discussion
We first investigated the effect of pectin on the disaccharide composition of cell-surface HS. In normal differentiated Caco-2 cells, approximately 85% of total disaccharide was sulfated in this study. This was greater than the extent reported previously, which showed that about 66% of disaccharide was sulfated in differentiated Caco-2 cells.Citation33,34) This was probably due to differences in passage number of Caco-2 cells. Incubating differentiated Caco-2 cells with pectin markedly increased the ratio of ∆Di-0S and decreased the ratio of ∆Di-diS1 and ∆Di-triS. As various growth factors and morphogens interact with HS by distinguishing the certain monosaccharide sequence and sulfation patterns of HS,Citation35) structural changes in the sulfation pattern of HS chains could have an effect on the physiological functions of HS. The pectin-induced structural alteration of HS also suggested that pectin directly stimulated differentiated Caco-2 cells.
Pectin treatment of differentiated Caco-2 cells significantly decreased the ratio of ∆Di-diS1 and ∆Di-triS. As HSulfs remove a 6-O-sulfate from the UA2S-GlcNS6S trisulfated disaccharide unit and UA-GlcNS6S disulfated disaccharide unit,Citation9,10) the involvement of HSulfs in the pectin-dependent structural alteration of HS was investigated. As Nagamine et al. demonstrated Sulf-1 and Sulf-2 mRNA expression in the small intestine of wild-type mice,Citation36) we found that both HSulf-1 and HSulf-2 were expressed in differentiated Caco-2 cells. HSulf-1 mRNA expression was markedly decreased by pectin treatment until 24 h after removal of pectin from the medium. On the other hand, HSulf-2 mRNA expression was upregulated by pectin administration. Taken together, differentiated Caco-2 cells may inhibit the transcription of HSulf-1 to emphasize the increased HSulf-2 expression in response to pectin stimulation. These findings suggest that decreased ratio of ∆Di-diS1 and ∆Di-triS was caused by the upregulated HSulf-2 expression.
Lamanna et al. reported that knockout of Sulfs affected the mRNA expression of 6-OSTs and 2-OST-1.Citation9) As we found that HSulf-1 expression was markedly inhibited by pectin administration, the effects of pectin on 6-OSTs and 2-OST-1 mRNA expression were investigated in differentiated Caco-2 cells. HS-modifying enzymes have multiple isoforms and their expression patterns exhibit tissue- and developmental stage-specific variation.Citation37) After pectin stimulation, 6-OST-1 mRNA was increased by about threefold and then gradually decreased to the normal level. On the other hand, no obvious increases were observed in 6-OST-2 mRNA expression. Increased expression of 6-OST-1 probably produced the increased ratio of ∆Di-di6S. 6-OST-3 was not expressed in differentiated Caco-2 cells (data not shown). Relative 2-OST-1 mRNA expression was decreased and the ratio of ∆Di-NS was unaffected by pectin treatment, suggesting that the decreased ratio of ∆Di-diS2 was caused by downregulated 2-OST-1 expression.
Fibronectin usually exists as a dimer of two closely identical subunits linked at the C-terminal and plays important roles in cell adhesion, migration, growth, and differentiation.Citation38) Fibronectin contains three types of repeating unit: 12 type I repeats, 2 type II repeats, and 15–17 type III repeats. Among them, the type III10 domain contains the RGD region, which binds to α5β1 integrin.Citation38) In this study, premixing pectin with FNIII1C suppressed the pectin-induced upregulation of HSulf-2. Due to covering of the fibronectin-binding domain in pectin with FNIII1C in advance, the pectin would not bind to fibronectin of differentiated Caco-2 cells.
Nagel et al. suggested that the enzymatically modified apple pectin interacted with Swiss 3T3 fibroblast cells and their interactions were mediated by α5β1 integrin.Citation24) As Swiss 3T3 fibroblast cells express fibronectins in the extracellular matrix,Citation25) we hypothesized that the effect of pectin on the upregulated HSulf-2 mRNA expression was mediated by fibronectin and α5β1 integrin in this study. Thus, to verify the involvement of α5β1 integrin, differentiated Caco-2 cells were pretreated with or without RGD peptides, and then incubated with pectins. The RGD peptides suppressed pectin-induced upregulation of HSulf-2 mRNA expression. This was because the RGD peptides covered α5β1 integrin on the surface of differentiated Caco-2 cells. Pectin-bound fibronectin could not interact with α5β1 integrin at the surface of differentiated Caco-2 cells. The galacturonan fragment of pectin from the aerial part of Comarum palustre L. was assumed to interact with β1/β2 chain of integrins,Citation39) suggesting that β1/β2 integrins on the Caco-2 cells may support the binding of pectin to fibronectin. These results support the suggestion that pectin from prune stimulated the expression of HSulf-2 via fibronectin and α5β1 integrin in differentiated Caco-2 cells. Pectin-induced downregulation of 6-OST-1 and upregulation of 2-OST-1 may also be controlled by fibronectin-bound α5β1 integrin to change the sulfation pattern of HS, which is suitable for interaction with bioactive proteins.
Integrins mediate cell adhesion to extracellular matrix proteins and regulate a number of intracellular signal transduction pathways. Integrins activate the extracellular signal regulated kinase (ERK) 1/2 by at least two different signaling pathways. Activated α5β1 integrin activates ERK1/2 via Src with tyrosine-phosphorylated focal adhesion kinase and subsequent activation of the MAPK pathway.Citation40–42) On the other hand, association of α5β1 integrin with the membrane protein caveolin activates ERK1/2 and subsequently tyrosine kinase Fyn phosphorylates the tyrosine of the adaptor protein Shc.Citation40,43,44) In Caco-2 cells, α5β1 integrin mediates fibronectin-induced epidermal growth factor receptor (EGFR) activation, which leads to EGFR-mediated activation of ERK1/2 and cell proliferation.Citation29,30) In this study, pectin-induced ERK1/2 activation was confirmed by Phos-tag Western blotting, suggesting that α5β1 integrin on the surface of differentiated Caco-2 cells mediated the interaction of pectin-bound fibronectin. Furthermore, we found that pretreatment with ERK1/2 inhibitor suppressed the upregulation of HSulf-2. These results suggest that pectin from prune stimulated the expression of HSulf-2 via ERK1/2 in differentiated Caco-2 cells. As Ai et al. suggested that QSulf-1 removes 6-O-sulfates from HS chains on the surface of HS proteoglycans to regulate binding of Wnts,Citation45) pectin-induced HSulf-2 expression may affect the affinity for Wnt proteins in this study. Further studies are required to investigate this point.
In conclusion, our findings showed that pectin extracted from prune stimulated the expression of HSulf-2 via fibronectin, α5β1 integrin, and ERK1/2, and changed the sulfated structure of cell-surface HS on the differentiated Caco-2 cells. These results will provide insight into the understanding of molecular mechanisms, in which pectin affected the morphology of the small intestine. Further studies are needed to understand the functional significance of cell-surface HS on pectin-induced morphological changes in the small intestine.
Acknowledgments
We thank Miki Corporation (Hyogo, Japan) for providing the concentrated prune juice, and we thank the members of the Division of Genomics Research, Life Science Research Center, Gifu University, for their support in conducting the experiments.
Notes
Abbreviations: ERK, extracellular related kinase; FNIII1C, C-terminal fragment containing two-thirds of III1 domain of fibronectin; HS, heparan sulfate; HSulf-2, human heparan sulfate 6-O-endosulfatase-2; 2-OST-1, heparan sulfate 2-O-sulfotransferase-1; 6-OST, heparan sulfate 6-O-sulfotransferase; 3-OST, heparan sulfate 3-O-sulfotransferase; NDST, heparan sulfate N-deacetylase/N-sulfotransferase.
References
- Lunn J, Buttriss JL. Carbohydrates and dietary fiber. Nutr. Bull. 2007;32:21–64.10.1111/nbu.2007.32.issue-1
- Leclere L, Cutsem PV, Michiels C. Anti-cancer activities of pH- or heat-modified pectin. Front. Pharmacol. 2013;4:128.
- Terpstra AH, Lapre JA, de Vries HT, Beynen AC. Dietary pectin with high viscosity lowers plasma and liver cholesterol concentration and plasma cholesteryl ester transfer protein activity in hamsters. J. Nutr. 1998;128:1944–1949.
- Tazawa K, Yatuzuka K, Yatuzuka M, Koike J, Ohkami H, Saito T, Ohnishi Y, Saito M. Dietary pectin with high viscosity lowers plasma and liver cholesterol concentration and plasma cholesteryl ester transfer protein activity in hamsters. Hum. Cell. 1999;12:189–196.
- Lee JC, Pak SC, Lee SH, Na CS, Lim SC, Song CH, Bai YH, Jang CH. Asian pear pectin administration during presensitization inhibits allergic response to ovalbumin in BALB/c mice. J. Altern. Complement. Med. 2004;10:527–534.10.1089/1075553041323867
- Olano-Martin E, Gibson GR, Rastall RA. Comparison of the in vitro bifidogenic properties of pectins and pectic-oligosaccharides. J. Appl. Microbiol. 2002;93:505–511.10.1046/j.1365-2672.2002.01719.x
- Ohkami H, Tazawa K, Yamashita I, Shimizu T, Murai K, Kobashi K, Fujimaki M. Effects of apple pectin on fecal bacterial enzymes in azoxymethane-induced rat colon carcinogenesis. Jpn. J. Cancer Res. 1995;86:523–529.10.1111/cas.1995.86.issue-6
- Habuchi O. Diversity and functions of glycosaminoglycan sulfotransferases. Biochim. Biophys. Acta. 2000;1474:115–127.10.1016/S0304-4165(00)00016-7
- Lamanna WC, Frese MA, Balleininger M, Dierks T. Sulf loss influences N-, 2-O-, and 6-O-sulfation of multiple heparan sulfate proteoglycans and modulates fibroblast growth factor signaling. J. Biol. Chem. 2008;283:27724–27735.10.1074/jbc.M802130200
- Frese MA, Milz F, Dick M, Lamanna WC, Dierks T. Characterization of the human sulfatase Sulf1 and its high affinity heparin/heparan sulfate interaction domain. J. Biol. Chem. 2009;284:28033–28044.10.1074/jbc.M109.035808
- Bishop JR, Schuksz M, Esko JD. Heparan sulphate proteoglycans fine-tune mammalian physiology. Nature. 2007;446:1030–1037.10.1038/nature05817
- Habuchi H, Habuchi O, Kimata K. Sulfation pattern in glycosaminoglycan: does it have a code? Glycoconjugate J. 2004;21:47–52.10.1023/B:GLYC.0000043747.87325.5e
- Brown L, Rosner B, Willett WW, Sacks FM. Cholesterol-lowering effects of dietary fiber: a meta-analysis. Am. J. Clin. Nutr. 1999;69:30–42.
- Tasman-Jones C, Owen RL, Jones AL. Semipurified dietary fiber and small-bowel morphology in rats. Digest. Dis. Sci. 1982;27:519–524.10.1007/BF01296731
- McCullough JS, Ratcliffe B, Mandir N, Carr KE, Goodlad RA. Dietary fibre and intestinal microflora: effects on intestinal morphometry and crypt branching. Gut. 1998;42:799–806.10.1136/gut.42.6.799
- Langhout DJ, Schutte JB, Van Leeuwen P, Wiebenga J, Tamminga S. Effect of dietary high- and low-methylated citrus pectin on the activity of the ileal microflora and morphology of the small intestinal wall of broiler chicks. Br. Poult. Sci. 1999;40:340–347.10.1080/00071669987421
- Perreault N, Katz JP, Sackett SD, Kaestner KH. Foxl1 controls the Wnt/beta-catenin pathway by modulating the expression of proteoglycans in the gut. J. Biol. Chem. 2001;276:43328–43333.10.1074/jbc.M104366200
- Yamamoto S, Nakase H, Matsuura M, Honzawa Y, Matsumura K, Uza N, Yamaguchi Y, Mizoguchi E, Chiba T. Heparan sulfate on intestinal epithelial cells plays a critical role in intestinal crypt homeostasis via Wnt/β-catenin signaling. Am. J. Physiol. Gastrointest. Liver Physiol. 2013;305:G241–G249.10.1152/ajpgi.00480.2012
- Galambos JT. The reaction of carbazole with carbohydrates. I. Effect of borate and sulfamate on the carbazole color of sugars. Anal. Biochem. 1967;19:119–132.10.1016/0003-2697(67)90141-8
- Shworak NW, Fritze LM, Liu J, Butler LD, Rosenberg RD. Cell-free synthesis of anticoagulant heparan sulfate reveals a limiting converting activity that modifies an excess precursor pool. J. Biol. Chem. 1996;271:27063–27071.
- Yoshida K, Miyauchi S, Kikuchi H, Tawada A, Tokuyasu K. Analysis of unsaturated disaccharides from glycosaminoglycuronan by high-performance liquid chromatography. Anal. Biochem. 1989;177:327–332.10.1016/0003-2697(89)90061-4
- Kinoshita E, Kinoshita-Kikuta E. Improved Phos-tag SDS-PAGE under neutral pH conditions for advanced protein phosphorylation profiling. Proteomics. 2011;11:319–323.10.1002/pmic.v11.2
- Grobe K, Esko JD. Regulated translation of heparan sulfate N-acetylglucosamine N-deacetylase/n-sulfotransferase isozymes by structured 5′-untranslated regions and internal ribosome entry sites. J. Biol. Chem. 2002;277:30699–30706.10.1074/jbc.M111904200
- Nagel MD, Verhoef R,Schols H, Morra M, Knox JP, Ceccone G, Della Volpe C,Vigneron P, Bussy C, Gallet M, Velzenberger E, Vayssade M, Cascardo G, Cassinelli C, Haeger A, Gilliland D, Liakos I, Rodriguez-Valverde M, Siboni S. Enzymatically-tailored pectins differentially influence the morphology, adhesion, cell cycle progression and survival of fibroblasts. Biochim. Biophys. Acta 2008;1780:995–1003.10.1016/j.bbagen.2008.04.002
- Verderio E, Nicholas B, Gross S, Griffin M. Regulated expression of tissue transglutaminase in Swiss 3T3 fibroblasts: effects on the processing of fibronectin, cell attachment, and cell death. Exp. Cell Res. 1998;239:119–138.10.1006/excr.1997.3874
- Danen EH, Aota S, van Kraats AA, Yamada KM, Ruiter DJ, van Muijen GN. Requirement for the synergy site for cell adhesion to fibronectin depends on the activation state of integrin alpha 5 beta 1. J. Biol. Chem. 1995;270:21612–21618.
- Zhang J, Li W, Sanders MA, Sumpio BE, Panja A, Basson MD. Regulation of the intestinal epithelial response to cyclic strain by extracellular matrix proteins. FASEB J. 2003;17:926–928.
- Sanders MA, Basson MD. Collagen IV-dependent ERK activation in human Caco-2 intestinal epithelial cells requires focal adhesion kinase. J. Biol. Chem. 2000;275:38040–38047.10.1074/jbc.M003871200
- Kuwada SK, Li X. Integrin alpha5/beta1 mediates fibronectin-dependent epithelial cell proliferation through epidermal growth factor receptor activation. Mol. Biol. Cell. 2000;11:2485–2496.10.1091/mbc.11.7.2485
- Kuwada SK, Kuang J, Li X. Integrin alpha5/beta1 expression mediates HER-2 down-regulation in colon cancer cells. J. Biol. Chem. 2005;280:19027–19035.10.1074/jbc.M410540200
- Lévy P, Robin H, Bertrand F, Kornprobst M, Capeau J. Butyrate-treated colonic Caco-2 cells exhibit defective integrin-mediated signaling together with increased apoptosis and differentiation. J. Cell Physiol. 2003;197:336–347.10.1002/(ISSN)1097-4652
- Aliaga JC, Deschênes C, Beaulieu JF, Calvo EL, Rivard N. Requirement of the MAP kinase cascade for cell cycle progression and differentiation of human intestinal cells. Am. J. Physiol. 1999;277:G631–G641.
- Molist A, Romarís M, Lindahl U, Villena J, Touab M, Bassols A. Changes in glycosaminoglycan structure and composition of the main heparan sulphate proteoglycan from human colon carcinoma cells (perlecan) during cell differentiation. Eur. J. Biochem. 1998;254:371–377.10.1046/j.1432-1327.1998.2540371.x
- Salmivirta M, Safaiyan F, Prydz K, Andresen MS, Aryan M, Kolset SO. Differentiation-associated modulation of heparan sulfate structure and function in CaCo-2 colon carcinoma cells. Glycobiology. 1998;8:1029–1036.10.1093/glycob/8.10.1029
- Ashikari-Hada S, Habuchi H, Kariya Y, Itoh N, Reddi AH, Kimata K. Characterization of growth factor-binding structures in heparin/heparan sulfate using an octasaccharide library. J. Biol. Chem. 2004;279:12346–12354.
- Nagamine S, Tamba M, Ishimine H, Araki K, Shiomi K, Okada T, Ohto T, Kunita S, Takahashi S, Wismans RG, van Kuppevelt TH, Masu M, Keino-Masu K. Organ-specific sulfation patterns of heparan sulfate generated by extracellular sulfatases Sulf1 and Sulf2 in mice. J. Biol. Chem. 2012;287:9579–9590.10.1074/jbc.M111.290262
- Nogami K, Suzuki H, Habuchi H, Ishiguro N, Iwata H, Kimata K. Distinctive expression patterns of heparan sulfate O-sulfotransferases and regional differences in heparan sulfate structure in chick limb buds. J. Biol. Chem. 2004;279:8219–8229.10.1074/jbc.M307304200
- Pankov R, Yamada KM. Fibronectin at a glance. J. Cell Sci. 2002;115:3861–3863.10.1242/jcs.00059
- Popov SV, Ovodova RG, Popova GY, Nikitina IR, Ovodov YS. Adhesion of human neutrophils to fibronectin is inhibited by comaruman, pectin of marsh cinquefoil Comarum palustre L., and by its fragments. Biochemistry (Mosc). 2005;70:108–112.10.1007/s10541-005-0058-7
- Gilcrease MZ. Integrin signaling in epithelial cells. Cancer Lett. 2007;247:1–25.10.1016/j.canlet.2006.03.031
- Schlaepfer DD, Hunter T. Focal adhesion kinase overexpression enhances ras-dependent integrin signaling to ERK2/mitogen-activated protein kinase through interactions with and activation of c-Src. J. Biol. Chem. 1997;272:13189–13195.10.1074/jbc.272.20.13189
- Schlaepfer DD, Jones KC, Hunter T. Multiple Grb2-mediated integrin-stimulated signaling pathways to ERK2/mitogen-activated protein kinase: summation of both c-Src- and focal adhesion kinase-initiated tyrosine phosphorylation events. Mol. Cell Biol. 1998;18:2571–2585.
- Wary KK, Mainiero F, Isakoff SJ, Marcantonio EE, Giancotti FG. The adaptor protein Shc couples a class of integrins to the control of cell cycle progression. Cell. 1996;87:733–743.10.1016/S0092-8674(00)81392-6
- Wary KK, Mariotti A, Zurzolo C, Giancotti FG. A requirement for caveolin-1 and associated kinase Fyn in integrin signaling and anchorage-dependent cell growth. Cell. 1998;94:625–634.10.1016/S0092-8674(00)81604-9
- Ai X, Do AT, Lozynska O, Kusche-Gullberg M, Lindahl U, Emerson CP Jr. QSulf1 remodels the 6-O sulfation states of cell surface heparan sulfate proteoglycans to promote Wnt signaling. J. Cell Biol. 2003;162:341–351.10.1083/jcb.200212083