Abstract
In the present study, we evaluated the modifying behavior of simple phenolic compounds on the sulfhydryl groups of glutathione and proteins. The catechol-type polyphenols, including protocatechuic acid, but neither the monophenols nor O-methylated catechol, can modify the sulfhydryl groups in a phenol oxidase-dependent manner. The possible involvement of polyphenol bioactivation in the enhancement of skin inflammation was also suggested.
Tyrosinase (EC 1.14.18.1) is one of the most popular phenol oxidases widely distributed from the animal to fungal kingdoms, and catalyses two types of reactions on phenolic substrates at a binuclear copper center; (a) the ortho-hydroxylation of the monophenols into o-diphenols (monophenolase activity), and (b) the oxidation of o-diphenols to o-quinones (diphenolase activity).Citation1) The product of both reactions is the o-quinone of the corresponding monophenol/diphenol.Citation1) Since tyrosinase is believed to be one of the potential pharmacological targets against cutaneous hyperpigmentation and melanoma, tyrosinase substrates as well as tyrosinase inhibitors have been used as chemotherapeutic agents to treat these diseases.Citation2) For example, 4-hydroxyanisole is a substrate for tyrosinase and exhibits depigmentation and tumor shrinkage by its topical application.Citation3) However, 4-hydroxyanisole clinical trials were terminated because of serious side effects.Citation4) We also demonstrated that topical treatment with a high dose (>10 μmol) of protocatechuic acid (PCA), a catechol-type benzoic acid, significantly enhanced mouse skin inflammation in a tyrosinase-dependent manner.Citation5) Treatment with PCA alone for 3 h also reduced the glutathione (GSH) level and detoxification enzyme activities in mouse skin, which was counteracted by the tyrosinase inhibitor arbutin (ArB).Citation5) Therefore, the tyrosinase-dependent bioactivation of phenolic substances is a prerequisite not only for depigmentation and anti-melanoma, but also for toxicity in normal tissue under specific conditions.
Dietary polyphenols, such as flavonoids and phenylpropanoids, are the most common phytochemicals in plant foods.Citation6) A wealth of data suggests that the relevant mechanisms of disease prevention by polyphenols, such as in cancer, are not related to their antioxidant properties, but are rather due to their prooxidant action and direct interaction with the target molecules.Citation7) We recently found that several polyphenols form the covalent adducts with thiols in vitro.Citation8,9) ROS and electrophiles can cause modifications to sensitive proteins that lead to changes in protein function.Citation10) However, the relationship between the tyrosinase-dependent bioactivation of polyphenols and their modifying ability of thiols has not yet been well characterized.
In the present study, we evaluated the tyrosinase-dependent modifying effect of several simple phenolic compounds on GSH. We observed the in vitro chemical modifications in GSH and a model protein by the bioactivated catechol-type phenolic compound. We also examined the enhancing effects of the phenolic compounds on 12-O-tetradecanoylphorbol-13-acetate (TPA)-induced inflammation in mice to suggest the possible involvement of their bioactivation in the inflammation enhancement.
PCA and butylated hydroxytoluene (BHT) were purchased from Nacalai Tesque (Kyoto, Japan). Arbutin and laccase were obtained from Sigma-Aldrich (St. Louis, MO, USA). TPA was obtained from Research Biochemicals International (Natick, MA, USA). Glyceraldehydes-3-phosphate dehydrogenase (GAPDH) was purchased from GE Healthcare UK Ltd. (Buckinghamshire, UK). Iodoacetyl-LC-biotin (IAB) was purchased from Thermo Fisher Scientific (Rockford, IL, USA).
Measurement of the GSH level was spectrophotometrically performed using commercial kit GSH-400 (Oxis International Inc., Foster City, CA, USA).Citation8) GSH (100 μM) was incubated with 50 μM phenolic compounds in 100 mm sodium phosphate buffer (pH 7.4) at 25 °C for 30 min with or without tyrosinase or laccase (30 units). Detection of the GSH-PCA adduct was performed by nuclear magnetic resonance (NMR) spectrometry using a Bruker AMX400 (400 MHz; Bruker Daltonics, Karlsruhe, Germany) as previously reported.Citation8) For the detection of the free protein sulfhydryls, GAPDH (0.2 mg/mL) was incubated with PCA and/or laccase (30 units) for 1 h at 37 °C. The reaction was terminated by centrifugal filtration (Microcon 30, molecular weight cutoff of 30,000; Millipore Co., Billerica, MA, USA) to remove the low molecular weight reactants. The samples were treated with IAB according to the manufacturer’s protocol and then separated by 10% SDS-PAGE. The western blotting and band visualization were performed as previously reported.Citation8)
The modifying effect of the phenolic compounds on TPA-induced inflammation was determined by two biomarkers, i.e. edema formation and myeloperoxidease (MPO) activity, as previously reported.Citation5) Female ICR mice (7 weeks old) were obtained from Japan SLC, Shizuoka, Japan. All values were expressed as the mean ± SD. Statistical significance was assessed by Student’s paired two-tailed t-test. A P value of 0.05 was regarded to be statistically significant.
We first examined the modification behavior of simple phenolic compounds with GSH, which is the most abundant intracellular sulfhydryl, in the presence or absence of tyrosinase. We used 12 simple phenolic compounds including naturally occurring PCA, luteolin, (+)-catechin, caffeic acid, (3,4-diOH-type), ferulic acid (3-OMe-4-OH type), p-coumaric acid (4-OH type), and gallic acid (3,4,5-triOH-type) as well as artificial pyrocatechol (o-diphenol), resorcinol (m-diphenol), hydroquinone (p-diphenol), its oxidized derivative (benzoquinone), and BHT (1-OH-4-Me). As shown in Fig. , the co-incubation of each o-diphenol-type phenolic compound (50 μM) with GSH (100 μM) for 30 min resulted in the significant loss of GSH in a tyrosinase-dependent manner, whereas neither the monophenols nor O-methylated catechol (p-coumaric acid, ferulic acid, and BHT) showed any effect. A triphenol gallic acid also showed the tyrosinase-dependent effect. An unsubstituted o-diphenol, pyrocatechol, but not resorcinol (m-diphenol) and hydroquinone (p-diphenol), decreased the GSH concentration in the presence of tyrosinase. Hydroquinone and benzoquinone consumed GSH independently of tyrosinase. Hydroquinone might be converted into benzoquinone possibly by auto-oxidation, which is supported by the previous report showing the electrophilicity of tert-butylhydroquinone.Citation11) These results suggested that substrates for the diphenolase activity, but not for the monophenolase activity, might participate in the tyrosinase-dependent GSH consumption under the tested conditions.
Fig. 1. GSH consumption by simple phenolic compounds.
Note: GSH (100 μM) was incubated with the test compounds (50 μM) for 130 min in the presence (filled bars) or absence (open bars) of tyrosinase. The amount of residual GSH was spectrophotometrically estimated using the commercial kit GSH-400.
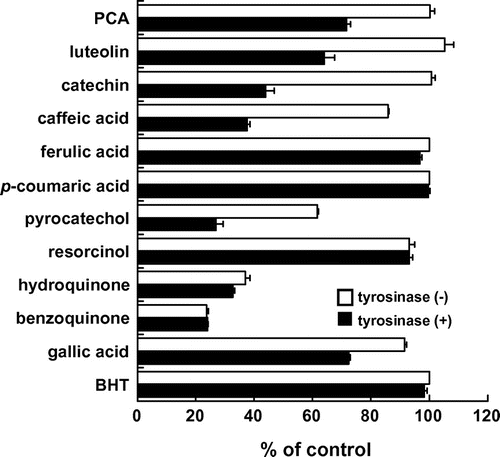
We used PCA as a model catechol compound to further investigate the modification mechanism, because PCA decreases the GSH level in vivo in a tyrosinase-dependent manner.Citation5) The incubation of 100 μM PCA with GSH in the presence of tyrosinase for 1 h resulted in an 80% loss of GSH (Fig. (A)). The α-tocopherol pretreatment significantly inhibited the tyrosinase-dependent modification of GSH, suggesting that, in addition to the electrophilic reaction, the oxidation of thiols might contribute to the reactivity of the bioactivated PCA. The products of PCA and GSH with tyrosinase are too complicated to identify by their NMR spectral data (data not shown). Therefore, we used another phenol oxidase, laccase (E.C. 1.10.3.2), since it has overlapping substrate ranges to tyrosinase and no monohydroxylase activity.Citation12) As shown in Fig. (A), incubation of PCA with GSH in the presence of laccase (30 unit) resulted in a 30% loss of GSH, and the co-treatment with α-tocopherol showed little effect. In addition, the GSH-PCA adduct formed by the diphenolase reaction was identified as 2-glutathionyl-PCA by its NMR spectral data (Fig. (B)). The electrophilic potential and position of S-glutathionylation in PCA is also supported by a previous report.Citation13) We examined the reactivity of the bioactivated PCA toward proteins using GAPDH containing four thiol groups per subunit. The GAPDH treated with both PCA and laccase decreased a positive band of approximately 36 kDa in a PCA concentration-dependent manner when assayed by a free sulfhydryl-positive IAB staining (Fig. (C)), suggesting that the bioactivated PCA can modify the free thiol groups in GAPDH.
Fig. 2. Modification behavior of PCA to GSH and GAPDH.
Note: (A) Phenol oxidase-dependent GSH consumption by PCA. GSH (100 μM) was incubated with or without PCA (100 μM) and α-tocopherol (1 mm) for 1 h in the presence of tyrosinase or laccase. The amount of residual GSH was spectrophotometrically estimated using the commercial kit GSH-400. (B) 1H NMR analysis of GSH-PCA adduct. A mixture of PCA and PCA-GSH produced by laccase was dissolved in D2O and then analyzed by NMR spectrometry. (C) Detection of free sulfhydryls of GAPDH treated with PCA and laccase. GAPDH (0.2 mg/mL) was incubated with PCA and laccase for 1 h. The free sulfhydryl groups of GAPDH were detected by SDS-PAGE/blotting with IAB staining.
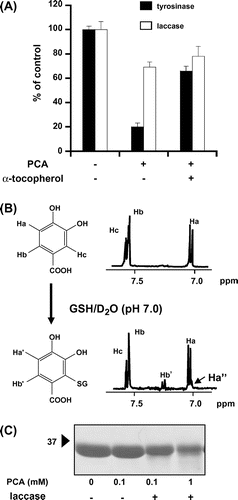
Because PCA is capable of reducing the GSH level and thus enhancing the TPA-induced inflammation in mouse skin,Citation5) we next examined the effects of (+)-catechin and BHT, the former of which tyrosinase-dependently consumed GSH the latter was inactive, on two TPA-induced inflammatory biomarkers, including edema formation and MPO activity. As shown in Table , the application of 20 μmol PCA 3 h before the TPA treatment showed a significant enhancement of edema formation and MPO activity compared to the TPA alone-treated control (163% of control and 238% of control, respectively). Similarly, the application of not only (+)-catechin but also tyrosinase reaction-negative BHT showed the enhancing effects on two inflammatory biomarkers (edema, 141%, p < 0.05 and 136%, p = 0.07, respectively; MPO, 179%, p = 0.07 and 160%, p < 0.05, respectively). We next assessed whether the enzyme-dependent bioactivation is involved in the inverse effects using a tyrosinase inhibitor ArB and cytochrome P450 (CYP) inhibitor SKF252a. The tyrosinase inhibitor significantly counteracted the PCA-enhanced inflammation as previously reported.Citation5) Although ArB showed the tendency to decrease the (+)-catechin-induced enhancement of edema formation (124% vs. 141%, p = 0.11), it showed no effect on the BHT-enhanced inflammation. On the other hand, SKF252a did not show any effect on the PCA- and (+)-catechin-induced enhancement, whereas it showed tendency to inhibit the BHT-enhanced edema formation (111% vs. 136%, p = 0.08). These results suggested that higher amounts of the catechol-type polyphenols, including (+)-catechin, have the potential to enhance skin inflammation through a tyrosinase-dependent bioactivation, even though the catechol-type catechins have a lower antioxidant activity and higher stability compared to the gallate-type catechins, such as (-)-epigallocatechin-3-gallate.Citation14) In addition, the toxic effects of BHT, possibly through CYP, led us to the idea that much attention should be paid to the administered dose of monophenols not only in the skin, but also in the liver and kidney, which have significant CYP activities and the potential target tissues for toxic effects of PCA and some tyrosinase substrates.Citation15,16)
Table 1. Modifying effects of PCA, (+)-Catechin, and BHT on TPA-Induced inflammatory parameters in ICR mouse skin.
In the present study, we have indicated that the catechol-type polyphenols can modify sulfhydryl groups in a phenol oxidase-dependent manner. The possible involvement of polyphenol bioactivation in the enhancement of TPA-induced skin inflammation was also suggested. Several phenolic toxicants, such as urushiols (poison ivy), cause type IV hypersensitivity through antigen formation via the hapten–protein adduct formation.Citation17) The occurrence of hapten–protein adduct formation in mouse skin treated with (+)-catechin or BHT and the relationship to the immunomodulatory effects remains to be elucidated. In addition, since the prooxidant or electrophilic action of polyphenols is quite important for their health-promoting property,Citation10) future studies will be needed to determine the optimal doses of polyphenols to control their beneficial/harmful balance.
Acknowledgments
We thank K. Torikai and H. Ohigashi (Prof. Emer.) at Kyoto University for their technical support. This study was mainly supported by Grant-in-Aid 25292073 (Y.N.) for scientific research from the Ministry of Education, Science, Culture, and Sports of Japan, and in part by Grant-in-Aid for research fellowships from the Japan Society for the Promotion of Science for Young Scientists (N.A.).
Notes
Abbreviations: PCA, protocatechuic acid; GSH, glutathione; ArB, arbutin; ROS, reactive oxygen species; TPA, 12-O-tetradecanoylphorbol-13-acetate; BHT, butylated hydroxytoluene; GAPDH, glyceraldehydes-3-phosphate dehydrogenase; IAB, Iodoacetyl-LC-biotin; NMR, nuclear magnetic resonance.
References
- García-Molina F, Muñoz JL, Varón R, Rodríguez-López JN, García-Cánovas F, Tudela J. A review on spectrophotometric methods for measuring the monophenolase and diphenolase activities of tyrosinase. J. Agric. Food Chem. 2007;55:9739–9749.10.1021/jf0712301
- Muñoz-Muñoz JL, Garcia-Molina F, Varon R, Garcia-Ruíz PA, Tudela J, Garcia-Cánovas F, Rodríguez-López JN. Suicide inactivation of the diphenolase and monophenolase activities of tyrosinase. IUBMB Life. 2010;62:539–547.
- Riley PA. Hydroxyanisole depigmentation: in-vitro studies. J. Pathol. 1969;97:193–206.10.1002/(ISSN)1096-9896
- Rustin GJ, Stratford MR, Lamont A, Bleehen N, Philip PS, Howells N, Watfa RR, Slack JA. Phase I study of intravenous 4-hydroxyanisole. Eur. J. Cancer. 1992;28A:1362–1364.10.1016/0959-8049(92)90520-C
- Nakamura Y, Torikai K, Ohto Y, Murakami A, Tanaka T, Ohigashi H. A simple phenolic antioxidant protocatechuic acid enhances tumor promotion and oxidative stress in female ICR mouse skin: dose- and timing-dependent enhancement and involvement of bioactivation by tyrosinase. Carcinogenesis. 2000;21:1899–1907.10.1093/carcin/21.10.1899
- Bravo L. Polyphenols: chemistry, dietary sources, metabolism, and nutritional significance. Nutr. Rev. 1998;56:317–333.
- Galati G, O’Brien PJ. Potential toxicity of flavonoids and other dietary phenolics: significance for their chemopreventive and anticancer properties. Free Radical Biol. Med. 2003;37:287–303.
- Ishii T, Ishikawa M, Miyoshi N, Yasunaga M, Akagawa M, Uchida K, Nakamura Y. Catechol type polyphenol is a potential modifier of protein sulfhydryls: development and application of a new probe for understanding the dietary polyphenol actions. Chem. Res. Toxicol. 2009;22:1689–1698.10.1021/tx900148k
- Ishii T, Mori T, Tanaka T, Mizuno D, Yamaji R, Kumazawa S, Nakayama T, Akagawa M. Covalent modification of proteins by green tea polyphenol (-)-epigallocatechin-3-gallate through autoxidation. Free Radical Biol. Med. 2008;45:1384–1394.10.1016/j.freeradbiomed.2008.07.023
- Nakamura Y, Miyoshi N. Electrophiles in foods: the current status of isothiocyanates and their chemical biology. Biosci. Biotechnol. Biochem. 2010;74:242–255.10.1271/bbb.90731
- Nakamura Y, Kumagai T, Yoshida C, Naito Y, Miyamoto M, Ohigashi H, Osawa T, Uchida K. Pivotal role of electrophilicity in glutathione S-transferase induction by tert-butylhydroquinone. Biochemistry. 2003;42:4300–4309.10.1021/bi0340090
- Thurston CF. The structure and function of fungal laccases. Microbiology. 1994;140:19–26.10.1099/13500872-140-1-19
- Saito S, Kawabata J. Synergistic effects of thiols and amines on antiradical efficiency of protocatechuic acid. J. Agric. Food Chem. 2004;52:8163–8168.10.1021/jf048970j
- Mori T, Ishii T, Akagawa M, Nakamura Y, Nakayama T. Covalent binding of tea catechins to protein thiols: the relationship between stability and electrophilic reactivity. Biosci. Biotechnol. Biochem. 2010;74:2451–2456.10.1271/bbb.100509
- Nakamura Y, Torikai K, Ohigashi H. Toxic dose of a simple phenolic antioxidant, protocatechuic acid, attenuates the glutathione level in ICR mouse liver and kidney. J. Agric. Food Chem. 2001;49:5674–5678.10.1021/jf0106594
- Moridani MY, Cheon SS, Khan S, O’Brien PJ. Metabolic activation of 4-hydroxyanisole by isolated rat hepatocytes. Drug Metab. Dispos. 2002;30:1063–1069.10.1124/dmd.30.10.1063
- Park BK, Pirmohamed M, Kitteringham NR. Role of drug disposition in drug hypersensitivity: A chemical, molecular, and clinical perspective. Chem. Res. Toxicol. 1998;11:970–988.