Abstract
In previous reports, hesperidin, a flavonoid glucoside from citrus fruit, is hydrolyzed to hesperetin, an aglycone of hesperidin, and converted to the hesperetin glucuronides (H7-OG and H3′-OG) in vivo and depresses blood glucose levels. But there are no reports on the activity of hesperetin glucuronides. To determine the activity of hesperetin glucuronides, H7-OG and H3′-OG were synthesized and peroxisome proliferator-activated receptor-γ (PPARγ) agonist activity was observed at 250 μM. These glucuronides accelerated the differentiation of 3T3-L1 cells into adipocytes at 10 μM. Furthermore, H7-OG showed additive effects in reporter gene assays and caused noncompetitive reactions in time-resolved fluorescence resonance energy transfer assays with a thiazolidinedione derivative. Our results indicated that hesperetin glucuronides activated PPARγ, accelerated adipocyte differentiation.
Graphical Abstract
Hesperidin, a flavonoid glucoside is converted to its glucuronides in vivo. Synthesized hesperetin glucuronides activated PPARγ and accelerated the differentiation of 3T3-L1 cells into adipocytes.

Key words:
Hesperidin, a flavanone-type flavonoid abundant in citrus fruit, has various physiological activities, such as capillary fragility-reducing effects,Citation1) antioxidant properties,Citation2) antihypertensive effects,Citation3) cholesterol-lowering activity,Citation4) anticancer activity, neuroprotective effects, and peroxisome proliferator-activated receptor-γ (PPARγ) agonist activity. Several studies with animals have investigated the absorption, bioavailability, and pharmacokinetics of citrus flavanones, such as hesperidin and naringin.Citation5−8) Orally ingested hesperidin is assumed to be hydrolyzed by β-glucosidase derived from gut microflora into hesperetin (an aglycone of hesperidin) and absorbed into the blood as conjugated metabolites, such as hesperetin glucuronides and sulfoglucuronides, in humans and rats.Citation9–11) On the other hand, free aglycone is not detected in circulating blood.
Recently, two studies on the biological activities of hesperidin glucuronides have been reported. Yamamoto et al. showed that hesperetin-7-O-β-d-glucuronide (H7-OG) decreased blood pressure in anesthetized spontaneously hypertensive rats, enhanced endothelium-dependent vasodilation in response to acetylcholine in aortas isolated from spontaneously hypertensive rats, and decreased hydrogen peroxide-induced intracellular adhesion molecule-1 and monocyte chemoattractant protein-1 mRNA expression in rat aortic endothelial cells.Citation12) Brand et al. showed that H7G and H3′G demonstrated interaction with ATP-binding cassette transporters, especially with ATP-binding cassette sub-family G member 2 (ABCG2) and ATP-binding cassette sub-family C member 2. Furthermore, an interesting difference between H7-OG and H3′-OG was indicated from the respect of the interaction with ABCG2: H7-OG stimulated the ATPase activity, whereas H3′-OG did not stimulate ATPase activity.Citation13)
PPARs belong to a subfamily of the nuclear receptor superfamily and are ligand-activated transcription factors that heterodimerize with the retinoid X receptor and bind to the PPAR response element, which is localized in the promoter regions of target genes.Citation14) Three subtypes of PPAR have been identified: PPARα, PPARδ, and PPARγ. In particular, PPARγ is strongly expressed in adipocytes and is responsible for adipogenesis. Adipogenesis is the process by which undifferentiated precursor cells differentiated into fat cells. In this process, several transcriptional families have been implicated, including the CCAAT/enhancer binding proteins C/EBPα, C/EBPβ, and C/EBPδ; PPARγ; and the basic helix-loop-helix protein ADD1/SREBP1c. In the present model of the transcriptional cascade leading to adipogenesis, C/EBPβ and C/EBPδ induce low levels of PPARγ and C/EBPα, which are then able to induce each other’s expression and maintains the differentiated state.Citation15) The activation of PPARγ alters the transcription of genes that encode lipoprotein lipase, fatty acid transporter protein, adipocyte fatty acid-binding protein, fatty acyl-CoA synthase, malic enzyme, glucokinase, and glucose transporter 4, and induces the expression of adiponectin.Citation16) In addition, improvements in endothelial function decrease inflammatory conditions and lower blood pressure. Consequently, PPARγ activation improves insulin sensitivity and decreases inflammation, plasma levels of free fatty acids, and blood pressure. This leads to inhibition of atherogenesis, improvement of endothelial function, and reduction of cardiovascular events.Citation16) The thiazolidinedione group of PPARγ ligands, such as troglitazone, pioglitazone, and rosiglitazone, has beneficial effects on serum lipids in diabetic patients and has been shown to inhibit the progression of atherosclerosis in animal models.Citation17)
To understand the precise mechanisms underlying the biological activities of orally ingested hesperidin, it is important to prepare hesperidin metabolites and evaluate their biological activity in vitro.
In this study, to investigate the effect of hesperetin glucuronides on lifestyle diseases, we evaluated the PPARγ agonist activity of H7-OG and hesperetin-3′-O-β-d-glucuronide (H3′-OG) and investigated the effects of these compounds on 3T3-L1 adipocyte differentiation. Furthermore, we examined the ability of H7-OG and H3′-OG as agonists to bind to the ligand-binding domain (LBD) of PPARγ.
Materials and methods
Chemicals
Dulbecco’s modified Eagle’s medium (DMEM) was purchased from Nissui Pharmaceutical Co. Ltd (Tokyo, Japan). Troglitazone was obtained from Cayman Chemicals (MI, USA). Fetal bovine serum (FBS) was obtained from PAA Laboratories (Pasching, Austria). A LanthaScreen™ TR-FRET PPARγ Competitive Binding Assay kit was purchased from Life Technologies (Tokyo, Japan). Other reagents were obtained from Nakalai Tesque (Kyoto, Japan).
Cell culture
COS-1 cells were obtained from RIKEN Bioresource Center (Ibaraki, Japan). 3T3-L1 cells were supplied by Health Science Research Resources Bank (Osaka, Japan). Each cell strain was maintained in DMEM supplemented with 10% FBS and 10% calf serum, respectively, at 37 °C in a 5% carbon dioxide—95% air incubator.
To differentiate 3T3-L1 cells into adipocytes, 4.2 × 104 cells were seeded into each well of a 24-well plate. After 2 d, the cells were treated with 0.5 mM isobutylmethylxanthine, 1 μM dexamethazone, and 1.7 or 2.8 × 10−3 μM insulin for 48 h. Then, the medium was changed to a fresh medium containing 1.7 or 2.8 × 10−3 μM insulin and changed thrice every 72 h. Troglitazone, H7-OG, and H3′-OG dissolved in dimethyl sulfoxide (DMSO) were added every time at the indicated concentrations. The concentration of DMSO in the culture medium was less than 0.1%.
Cell staining
Oil Red O staining was used to examine lipid accumulation in 3T3-L1 cells differentiated into adipocytes. In brief, the cells were fixed with 10% formaldehyde after being washed with phosphate-buffered saline and stained with a saturated solution of Oil Red O in 60% isopropanol solution for 15 min. After removal of the staining solution, the cultures were observed under a microscope. Spectrophotometric quantification of the stain was performed by extracting the stained oil droplets in the cell monolayers with isopropanol. Then, the absorbance was measured at 570 nm.
Synthesis of H7-OG
H7-OG was synthesized as reported previously.Citation18) A solution of hesperetin (0.3 mmol in 5 mL of anhydrous chloroform/pyridine) was added to acetobromo-α-d-glucuronic acid methyl ester (0.70 mmol), silver carbonate (0.70 mmol), and 4 Å molecular sieves (metal aluminosilicates having many pores that absorb water) at 0 °C. The mixture was stirred for 36 h in darkness at room temperature under an atmosphere of argon. After the mixture was filtered, the filtrate was dissolved in 30 mL of ethyl acetate (EtOAc). The EtOAc phase was washed with 10% citric acid and saturated sodium chloride, subsequently dehydrated with anhydrous sodium sulfate, and concentrated under vacuum. The concentrate was applied to a silica gel column, 150 × 30 mm i.d. 63–210 mm, neutral silica gel 60 N (Kanto Chemical Co., Inc., Tokyo, Japan), conditioned with chloroform, and eluted with chloroform–methanol (40:1). The crude product was dissolved in 5 mL of anhydrous methanol. The solution was stirred for 30 min at 0 °C after adding 28% sodium methoxide in methanol (150 mL). The solution was then stirred for 1 h at room temperature after adding 300 mL of distilled water. The solution was neutralized with Amberlite IR-120 (H+) resin (ICN Biochemicals Inc., USA) and concentrated under vacuum. The residue was applied to a silica gel column, and the eluent was applied to a high-performance liquid chromatography (HPLC) system (Gilson Inc., Madison, USA) equipped with a C18 reverse-phase column, 250 × 10 mm, Mightysil RP-18 (Kanto Chemical Co., Inc., Tokyo, Japan). The mobile phase consisted of two eluents: 0.1% aqueous trifluoroacetic acid (solvent A) and acetonitrile (solvent B). Isocratic elution at 30% B was performed for 30 min. A single peak separated by HPLC was collected. H7-OG was obtained at an overall yield of 5% as a yellow amorphous powder. 1H NMR (DMSO-d6, 400 MHz) δ: 2.73 (1H, dd, J = 17 and 3 Hz, H-3b), 3.23 (1H, m, H-2′′), 3.24 (1H, dd, J = 12 and 3 Hz, H-3a), 3.31–3.28 (2H, m, H-3′′ and H-4′′) 3.73 (3H, s, -OMe), 3.95 (1H, dd, J = 9.4 and 2.7 Hz, H-5′′), 5.13 (1H, d, J = 7.1 Hz, H-1′′), 5.46 (1H, dd, J = 12.8 and 2.7 Hz, H-2), 6.11 (1H, d, J = 2.2 Hz, H-6), 6.16 (1H, d, J = 2.1 Hz, H-8), 6.85 (1H, dd, J = 10.1 and 1.8 Hz, H-6′), 6.90 (1H, m, H-5′), 6.94 (1H, d, J = 2.2 Hz, H-2′).
Synthesis of H3′-OG
H3′-OG was synthesized as reported previously.Citation19) Benzoyl chloride (2.15 mL, 18.5 mmol) was added dropwise to an ice-cold solution of hesperetin (1 equiv.) in pyridine (15 mL), and the reaction mixture was stirred overnight at room temperature. The mixture was extracted with EtOAc (300 mL) and then washed with water (200 mL), 2 M hydrochloride (3 × 100 mL), saturated aqueous sodium bicarbonate (100 mL), and saturated aqueous sodium chloride (100 mL). The combined organic extract was dried over sodium sulfate, filtered, and concentrated. The product was purified by precipitation from EtOAc/n-hexane to give 7-O-benzoylhesperetin with a yield of 60%. 7-O-Benzoylhesperetin (0.5 g, 1.3 mmol) was added to a solution of acetobromo-α-d-glucuronic acid methyl ester (0.45 g, 1 equiv.) in dry methylene chloride (15 mL) containing 4 Å molecular sieves. The reaction mixture was cooled in an ice water bath for 15 min under nitrogen atmosphere. Then, boron trifluoride diethyl etherate (170 μL, 1 equiv.) was added, and the mixture was diluted with EtOAc, filtered on Celite, concentrated, and purified by column chromatography (c-hexane/EtOAc, 6:4) to provide 7-O-benzoylhesperetin 3′-O-[methyl (2′′,3′′,4′′-tri-O-acetyl)-β-d-glucopyranosyl uronate with a yield of 73%. To a solution of 7-O-benzoylhesperetin 3′-O-[methyl (2′′,3′′,4′′-tri-O-acetyl)-β-d-glucopyranosyl uronate (0.85 mmol) in methanol (30 mL) under nitrogen atmosphere at 0 °C was slowly added a 0.5 M aqueous solution of sodium carbonate (13 mL). The mixture was stirred at room temperature for 5 h. Then, Dowex 50WX4-50 ion-exchange resin (H+ form) was added under stirring to lower the pH to approximately 6. The resin was filtered off, and the filtrate was concentrated at 50 °C under vacuum. Purification was performed by semiseparative reversed-phase HPLC with a mobile phase of 30% acteonitrile/water containing 0.1% trifluoroacetic acid on a 250 × 10 mm Mightysil RP-18 column. H3′-OG was obtained at an overall yield of 34% as a yellow amorphous powder. 1H NMR (CDOD3, 400 MHz) δ: 2.72 (1H, dd, J = 17.0 and 3.7 Hz, H-3b), 3.09 (1H, dd, J = 17.0 and 12.4 Hz, H-3a), 3.45–3.62 (3H, m, H-2′′, 3′′ and 4′′), 3.86 (3H, s, -OMe), 3.93 (1H, dd, J = 9.6 and 2.7 Hz, H-5′′), 4.97 (1H, d, J = 6.4 Hz, H-1′′), 5.36 (1H, m, H-2), 5.86 (1H, m, H-6), 5.89 (1H, m, H-8), 7.02 (1H, d, J = 8.3 Hz, H-5′), 7.14 (1H, d, J = 8.2 Hz, H-6′) 7.27 (1H, d, J = 1.8 Hz, H-2′)
Authentic compounds, H7-OG and H3′-OG, were gifted from Dr. Hikaru Matsumoto, National Institute of Fruit Tree Science. Synthesized compounds were identified using HPLC with authentic compounds.
Luciferase reporter assay
An expression plasmid of human PPARγ2 full-length (pPPARγ2) and the luciferase reporter plasmid containing PPAR-binding cis-element (pPPARE) were kindly donated by Dr S. Kato (University of Tokyo, Tokyo, Japan). We used Effectene transfection reagent to transiently transfect COS-1 cells (5 × 105 cells) with pPPARγ2 (1 μg), pPPARE (1 μg), and pSEAP control vector (Clontech, CA, USA). Transfections were performed in 60 mm culture dishes according to the manufacturer’s instructions. After 16 h, the transfected cells were recovered and seeded into 96-well white multiwell plates, the indicated concentrations of the test compounds were added, and the plates were cultured for an additional 24 h at 37 °C in a 5% carbon dioxide incubator. The concentration of DMSO in the culture medium was 1 or 2%. A Steady-Glo® Luciferase Assay System (Promega, Madison, WI) and a Great EscAPe SEAP Reporter System3 (Clontech) were used according to the manufacturer’s instructions to measure luciferase activities and secreted alkaline phosphatase (SEAP) activities in each well. The SEAP activity level was used to correct the luciferase activity in each well. For all data, each value represents the mean ± standard error of six experiments.
Reverse transcription polymerase chain reaction (PCR) analysis
An RNeasy Protect Mini Kit (Qiagen) was used to extract total RNA (1 μg). Random hexamers and Moloney murine leukemia virus reverse transcriptase (Life Technologies, NY, USA) were used according to the manufacturer′s protocols to generate first-strand cDNA from total RNA. PCR was performed using Taq DNA polymerase (Life technologies). The primers used for PCR were PPARγ: forward, 5′-GAG CTG ACC CAA TGG TTG CTG-3′ and reverse, 5′-CCA AAC CTG ATG GCA TTG TGA GAC-3′; mouse glyceraldehyde dehydrogenase: forward, 5′-ACC ACA GTC CAT GCC ATC AC-3′ and reverse, 5′-TCC ACC ACC CTG TTG CTG TA-3′; CCAAT/enhancer-binding protein (C/EBP)α: forward, 5′-AGC CAA GAA GTC GGT GGA CAA G-3′ and reverse, 5′-TAG AGA TCC AGC GAC CCG AAA C-3′. Thermal cycling conditions for PCR were 95 °C for 1 min, followed by 28 cycles of 94 °C for 40 s, 57 °C for 40 s, and 72 °C for 60 s. A transilluminator was used to observe mRNA expression levels after 2.5% agarose gel electrophoresis and staining with ethidium bromide. The data were photographed using digital camera, and quantitative analysis was performed using Image J ver. 1.47 software (National Institutes of Health, USA).
LanthaScreen™ TR-FRET PPARγ competitive binding assay
A Time-resolved fluorescence resonance energy transfer (TR-FRET) assay was performed in vitro using the LanthaScreen™ TR-FRET PPARγ competitive binding assay kit according to the manufacturer’s instructions (Life Technologies). Measurements were performed on an Infinite® M1000 microplate reader (Tecan, Männedorf, Switzerland), with instrument settings as described in the manufacturer’s instructions for LanthaScreen assays.
Results
Hesperetin glucuronides activate PPARγ
To investigate the PPARγ agonist activities of hesperetin glucuronides of hesperidin and hesperetin metabolites (Fig. ), we synthesized H7-OG and H3′-OG according to the methods of Matsumoto et al. and Khan et al., respectively.Citation18,19) The PPARγ agonist activities of H3′-OG and H7-OG were assessed in a luciferase ligand assay using an expression plasmid of human PPARγ2 with COS-1 cells. At 25 μM, hesperetin and hesperidin showed 88 and 31%, respectively, of luciferase activity of 10 μM troglitazone, a known PPARγ agonist, (Fig. ), whereas at 250 μM, H3′-OG and H7-OG showed 67 and 76%, respectively, of luciferase activity of 10 μM troglitazone. Hesperetin and hesperidin showed cytotoxicity at >25 μM, but H3′-OG and H7-OG showed no cytotoxicity at 250 μM (data not shown). The concentration of DMSO in the culture medium was 1%.
Fig. 2. PPARγ agonist activity of hesperidin, hesperetin, and hesperetin glucuronides.
Notes: COS-1 cells were co-transfected with the luciferase reporter plasmid, p17m2G, which contained a GAL4 upstream-activating sequence in the promoter region, an expression vector for the human PPARγ LBD fused to the GAL4 DNA-binding domain, pPPARγ-GAL4, and SEAP control vector, pSEAP. The results are relative to the luciferase expression level and were normalized to the SEAP activity. Hesperidin derivatives and troglitazone, a positive control PPARγ agonist, were dissolved in DMSO and added to the medium of the transfected cells. Each value is presented as the mean ± standard error of three experiments. *p < 0.05, significantly different from cells adding DMSO.

H7-OG and H3′-OG accelerate 3T3-L1 adipocyte differentiation
To evaluate the effects of H7-OG and H3′-OG on adipocyte differentiation, 3T3-L1 cells were differentiated into adipocytes with or without H7-OG and H3′-OG at 10 μM in the presence of a low concentration of insulin (2.8 × 10−3 μM). Lipid accumulation was examined by Oil Red O staining, and the cultures were observed under a microscope (Fig. (A)). In the cells differentiated with H7-OG and H3′-OG in the presence of a low concentration of insulin, lipid accumulation increased significantly as compared with that in the cells without hesperetin glucuronides. The ratio of its amount was 29% for H3′-OG and 12% for H7-OG as compared with that in fully differentiated cells (Fig. (B)). The concentration of DMSO in the culture medium was less than 0.1%.
Fig. 3. Adipocyte differentiation accelerative activity of hesperetin glucuronides on 3T3-L1 cells.
Notes: To differentiate 3T3-L1 cells into adipocytes, 4.2 × 104 cells were seeded into each well of a 24-well plate. After 2 d, the cells were treated with 0.5 mM isobutylmethylxanthine, 1 μM dexamethazone, and 1.7 or 2.8 × 10−3 μM insulin for 48 h. The medium was then changed to a fresh medium containing 1.7 or 2.8 × 10−3 μM insulin, with or without hesperidin glucuronides, and was changed thrice every 72 h. Oil Red O staining was used to examine lipid accumulation in the 3T3-L1 cells differentiated into adipocytes. In brief, the cells were fixed with 10% formaldehyde after being washed with phosphate-buffered saline and stained with a saturated solution of Oil Red O in 60% isopropanol solution for 15 min. After removal of the staining solution, the cultures were observed under a microscope (A). (a), (b), (c), and (d) show, respectively, the cells with additions of 2.8 × 10−3 μm insulin, 1.7 μm insulin, 1.7 nm insulin with 10 μm H3′-OG, and 1.7 nm insulin with 10 μm H7-OG. Spectrophotometric quantification of the stain was performed by extracting the stained oil droplets in the cell monolayers with isopropanol. Then, the absorbance was measured at 570 nm (B). This experiment was performed thrice and same results were acquired.
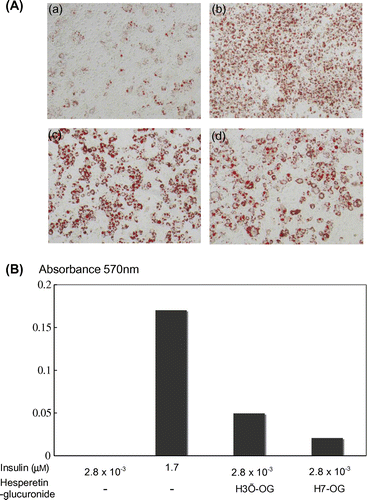
H7-OG and H3′-OG induce expression of not only PPAR target genes but also the PPARγ gene in 3T3-L1 cells
To determine whether H7-OG and H3′-OG actually regulate the endogenous PPAR response elements in intact cells, the expression level of the PPARγ target genes, adiponectin and C/EBPα, were measured in 3T3-L1 cells in the presence of H7-OG or H3′-OG. As shown in Fig. (A) and (B), adiponectin and C/EBPα expression was induced by the addition of 10 μM H7-OG or H3′-OG, and the induced expression was approximately equal to that in the fully differentiated cells in the presence of 1.7 μM insulin. Furthermore, the addition of H7-OG or H3′-OG also slightly increased PPARγ gene expression.
Fig. 4. Upregulation of mRNA expression of adiponectin, C/EBPα, and PPARγ genes in 3T3-L1 cells by hesperetin glucuronides.
Notes: 3T3-L1 cells were differentiated by the addition of 1.7 or 2.8 × 10−3 μm insulin containing 10 μm hesperetin glucuronides. After total RNA was prepared, the adiponectin, C/EBPα, and PPARγ genes were amplified after cDNA synthesis, and the expression levels were observed under a transilluminator after agarose gel electrophoresis and staining with ethidium bromide. The data were photographed using digital camera, and quantitative analysis was performed using Image J ver. 1.47 software (National Institutes of Health, USA).

H7-OG exerts an additive effect with troglitazone on PPARγ agonist activity
To investigate the synergism between the hesperetin glucuronides and troglitazone, a thiazolidinedione compound, on PPARγ agonist activity, the additive effects of H7-OG and H3′-OG combined with troglitazone at 10 μM, which exerted the highest agonist activity, were evaluated (Fig. ). At the concentration of 10 μM of troglitazone, luciferase activity increased to 4.1-fold against the activity of DMSO, and troglitazone with 250 μM of H7-OG increased luciferase activity to 5.5-fold against the activity of DMSO, but troglitazone with 250 μM of H3′-OG had no additive activity. The concentration of DMSO in the culture medium was 2%.
The interaction of H7-OG and H3′-OG with the PPARγ receptor
The TR-FRET assay was used to investigate the ability of H7-OG and H3′-OG as agonists to bind to LBD of PPARγ. The assay is based on the interaction of the PPARγ LBD indirectly labeled with teribium by the addition of the co-activator peptide C33 with fluorescein.Citation20) We used pioglitazone as a known PPARγ agonist. Pioglitazone was able to induce FRET in a concentration-dependent manner with an EC50 value of 2.3 μM (Fig. ). H7-OG and H3′-OG did not show similar PPARγ LBD binding responses.
Fig. 6. Hesperetin glucuronides show no competitive activity with a thiazolidinedione derivative against PPARγ
Notes: The LanthaScreen TR-FRET PPARγ assay was performed according to the manufacturer’s instructions. The data for pioglitazone, H7-OG, and H3′-OG are indicated by closed circles, closed triangles, and closed squares, respectively.
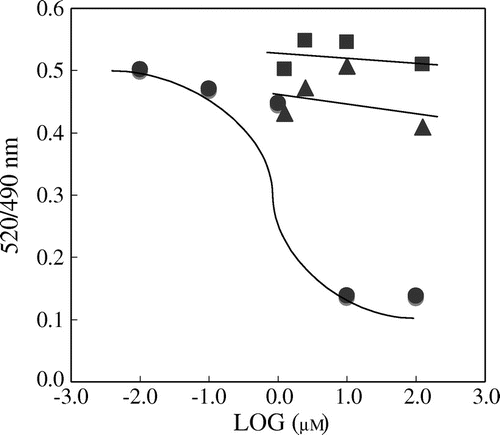
Discussion
Hesperidin, a well-known flavonoid glycoside, is present at high concentrations almost exclusively in citrus fruits and has various physiological activities. PPARγ agonist activity in vitro and its suppressive effect on blood glucose levels in vivo have been reported,Citation21) findings which suggest the potential therapeutic value against various metabolic diseases. However, orally investigated hesperidin is hydrolyzed to hesperetin in the gastrointestinal tract and converted to hesperetin glucuronides (H7-OG and H3′-OG) during absorption. Hesperetin glucuronides are the main circulating metabolites in human and rat plasma, and hesperidin is present only at very low levels. On this basis, we presumed that hesperetin glucuronides are the active compounds exhibiting suppressive effects on blood glucose levels and that the effect is caused by PPARγ agonist activity.
Initially, we synthesized H7-OG and H3′-OG, and evaluated the PPARγ agonist activity of these compounds. H7-OG and H3′-OG showed PPARγ agonist activity, although only at a high concentration. On the basis of this result, we presumed that oral ingestion of hesperidin was effective for the suppression of blood glucose levels. On the other hand, the maximum concentrations of hesperetin metabolites determined in human plasma 5–7 h after the intake of orange juice are 1.3–2.2 μM for the intake of 130–220 mg. Furthermore, it has been reported that H7-OG and H3′-OG were detected in rat plasma as the predominant metabolites at 0.2 μM after oral administration of hesperidin (50 mg/kg).Citation21) Taking these data into account, the concentrations of H7-OG and H3′-OG that showed PPARγ agonist activity were very high; thus, we believed that their contributions to the suppression of blood glucose levels were small.
Preadipocyte 3T3-L1 cells differentiate into adipocytes following the uptake of glucose and stimulating lipids caused by the addition of insulin to the medium. PPARγ agonists accelerate adipocyte differentiation.Citation22) H7-OG and H3′-OG at 10 μM accelerated adipocyte differentiation in the presence of a low concentration of insulin. This concentration was approximately 25 times lower than that of PPARγ agonists showing similar activity. These results suggested that H7-OG and H3′-OG targeted other compounds in addition to PPARγ.
In previous studies, it has been reported that the activation of C/EBPβ and C/EBPδ induces low levels expression of PPARγ and C/EBPα, which are then able to induce each other’s expression and maintains the differentiated state.Citation15) From these results, it is suggested that hesperetin glucuronides activate C/EBPβ, C/EBPδ, and/or those upstream factors, increase the expression of PPARγ, and then also the expression of adiponectin is induced.
The relative activity between troglitazone and hesperetin glucuronides shown in Fig. is different from the data obtained in Fig. . We think that one of the reasons is the concentration of DMSO added to culture medium because our reporter gene assay system is very sensitive to it.
In recent years, some flavonoids have been identified that are PPAR ligands.Citation23) From analysis of the crystal structure of the PPARγ LBD, luteolin (5,7,3′,4′-tetrahydroxyflavone) in these compounds occupies a buried ligand-binding pocket of PPARγ but binds an inactive PPARγ LBD conformer and occupies a space near the β-sheet region far from the activation helix.Citation24) On the basis of our results on the additive effects and noncompetitive reactions with a thiazolidinedione derivative, we presumed that H7-OG and H3′-OG did not bind to PPARγ in the same manner as thiazolidinedione derivatives.
In conclusion, it is suggested that hesperetin glucuronides activate PPARγ, improve insulin resistance, accelerate adipocyte differentiation, and elevate glucose uptake activity following binding to an unknown factor related to adipocyte differentiation. On the basis of these results, we estimate that intake of hesperidin depresses blood glucose levels and is useful of the improvement of insulin resistance.
Supplemental material
The supplemental material for this paper is available at http://dx.doi.org/10.1080/09168451.2014.910097.
Supplemental Fig. 1 caption
Download MS Word (19 KB)Supplemental Fig. 1
Download MS Power Point (42 KB)Acknowledgment
This work was supported by a matched fund subsidy for private universities from the Ministry of Education, Culture, Sports, Science and Technology (MEXT).
Notes
Abbreviations: ABC, ATP-binding cassette; C/EBP, CCAAT/enhancer-binding protein; DMEM, Dulbecco’s modified Eagle’s medium; EtOAc, ethyl acetate; FBS, fetal bovine serum; H3′-OG, hesperetin-3′-O-β-d-glucuronide; H7-OG, hesperetin-7-O-β-d-glucuronide; HPLC, high-performance liquid chromatography; LBD, ligand-binding domain; PCR, polymerase chain reaction; PPARs, peroxisome proliferator-activated receptors; SEAP, secreted alkaline phosphatase; TR-FRET, time-resolved fluorescence resonance energy transfer.
References
- Garg A, Garg S, Zaneveld LJ, Singla AK. Chemistry and pharmacology of the Citrus bioflavonoid hesperidin. Phytother. Res. 2001;15:655–669.10.1002/(ISSN)1099-1573
- Wilmsen PK, Spada DS, Salvador M. Antioxidant activity of the flavonoid hesperidin in chemical and biological systems. J. Agric. Food Chem. 2005;53:4757–4761.10.1021/jf0502000
- Galati EM, Trovato A, Kirjavainen S, Forestieri AM, Rossitto A, Monforte MT. Biological effects of hesperidin, a Citrus flavonoid. (Note III): antihypertensive and diuretic activity in rat. Farmaco. 1996;51:219–221.
- Bok SH, Lee SH, Park YB, Bae KH, Son KH, Jeong TS, Choi MS. Plasma and hepatic cholesterol and hepatic activities of 3-hydroxy-3-methyl-glutaryl-CoA reductase and acyl CoA: cholesterol transferase are lower in rats fed citrus peel extract or a mixture of citrus bioflavonoids. J. Nutr. 1999;129:1182–1185.
- Booth AN, Jones FT, DeEds F. Metabolic fate of hesperidin, eriodictyol, homoeridictyol, and diosmin. J. Biol. Chem. 1958;230:661–668.
- Hackett AM, Marsh I, Barrow A. The bilary excretion of flavanones in the rat. Xenobiotica. 1979;9:491–501.10.3109/00498257909087263
- Choudhury R, Chowrimootoo G, Srai K, Debnam E, Rice-Evans CA. Interactions of the flavonoid naringenin in the gastrointestinal tract and the influence of glycosylation. Biochem. Biophys. Res. Commun. 1999;265:410–415.10.1006/bbrc.1999.1695
- Felgines C, Texier O, Morand C, Manach C, Scalbert A, Regerat F, Remesy C. Bioavailability of the flavanone naringenin and its glycosides in rats. Am. J. Physiol. Gastrointest. Liver Physiol. 2000;279:G1148–G1154.
- Flowers HM. Synthesis of two phosphate-containing “heptasaccharide” fragments of the capsular polysaccharides of Streptococcus pneumoniae types 6A and 6B. Carbohydr. Res. 1971;18:211–218.10.1016/S0008-6215(00)80344-4
- Koenigs W, Knorr E. Over some derivatives of grape sugar and the galactose. Ber. Deu. Chem. Ges. 1901;34:957–981.10.1002/(ISSN)1099-0682
- Nielsen SE, Breinholt V, Justesen U, Cornett C, Dragsted LO. In vitro biotransformation of flavonoids by rat liver microsomes. Xenobiotica. 1998;28:389–401.10.1080/004982598239498
- Yamamoto M, Jokura H, Hashizume K, Ominami H, Shibuya Y, Suzuki A, Hase T, Shimotoyodome A. Hesperidin metabolite hesperetin-7-O-glucuronide, but not hesperetin-3′-O-glucuronide, exerts hypotensive, vasodilatory, and anti-inflammatory activities. Food Funct. 2013;4:1346–1351.10.1039/c3fo60030k
- Brand W, Oosterhuis B, Krajcsi P, Barron D, Dionisi F, Bladeren PJ, Rietjens IM, Williamson G. Interaction of hesperetin glucuronide conjugates with human BCRP, MRP2 and MRP3 as detected in membrane vesicles of overexpressing baculovirus-infected Sf9 cells. Biopharm. Drug Dispos. 2011;32:530–535.10.1002/bdd.780
- Chinetti G, Fruchart JC, Staels B. Peroxisome proliferator-activated receptors (PPARs): nuclear receptors at the crossroads between lipid metabolism and inflammation. Inflamm. Res. 2000;49:497–505.10.1007/s000110050622
- Rosen ED, Hsu CH, Wang X, Sakai S, Freeman MW, Gonzalez FJ, Spiegelman BM. C/EBPalpha induces adipogenesis through PPARgamma: a unified pathway. Genes Dev. 2002;16:22–26.10.1101/gad.948702
- Francis GA, Annicotte JS, Auwerx J. PPAR agonists in the treatment of atherosclerosis. Curr. Opin. Pharmacol. 2003;3:186–191.10.1016/S1471-4892(03)00014-6
- Gurnell M, Savage DB, Chatterjee VK, O’Rahilly S. The metabolic syndrome: peroxisome proliferator-activated receptor gamma and its therapeutic modulation. J. Clin. Endocrinol. Metab. 2003;88:2412–2421.10.1210/jc.2003-030435
- Matsumoto H, Ikoma Y, Sugiura M, Yano M, Hasegawa Y. Identification and quantification of the conjugated metabolites derived from orally administered hesperidin in rat plasma. J. Agric. Food Chem. 2004;52:6653–6659.10.1021/jf0491411
- Khan MK, Rakotomanomana N, Loonis M, Dangles O. Chemical synthesis of citrus flavanone glucuronides. J. Agric. Food. Chem. 2010;58:8437–8443.
- Stafslien DK, Vedvik KL, De Rosier T, Ozers MS. Analysis of ligand-dependent recruitment of coactivator peptides to RXRbeta in a time-resolved fluorescence resonance energy transfer assay. Mol. Cell. Endocrinol. 2007;264:82–89.
- Yoshida H, Takamura N, Shuto T, Ogata K, Tokunaga J, Kawai K, Kai H. The citrus flavonoids hesperetin and naringenin block the lipolytic actions of TNF-alpha in mouse adipocytes. Biochem. Biophys. Res. Commun. 2010;394:728–732.
- Kletzien RF, Clarke SD, Ulrich RG. Enhancement of adipocyte differentiation by an insulin-sensitizing agent. Mol. Pharmacol. 1992;41:393–398.
- Huang TH, Kota BP, Razmovski V, Roufogalis BD. Herbal or natural medicines as modulators of peroxisome proliferator-activated receptors and related nuclear receptors for therapy of metabolic syndrome. Basic Clin. Pharmacol. Toxicol. 2005;96:3–14.
- Puhl AC, Bernardes A, Silveira RL, Yuan J, Campos JL, Saidemberg DM, Palma MS, Cvoro A, Ayers SD, Webb P, Reinach PS, Skaf MS, Polikarpov I. Mode of peroxisome proliferator-activated receptor γ activation by luteolin. Mol. Pharmacol. 2012;81:788–799.