Abstract
The laver (Porphyra tenera), red seaweed, has been reported to have anticancer activity, but little is known about its molecular mechanisms of action. The objective of this study was to determine the effects of laver extract on cancer cell proliferation, invasion, and metastasis in SK-Hep1 cells using migration and invasion assays. We also investigated the relationship of MMP-2/-9 and TIMP-1/-2 expression at both the protein and gene level in SK-Hep1 human hepatoma carcinoma cells after laver extract treatment. Laver extract inhibited cancer cell growth in a dose-dependent manner. In an invasion assay conducted in Transwell chambers, laver extract showed 19.6 and 27.2% inhibition of cancer cell at 200 and 400 μg/mL, respectively, compared to the control. The mRNA levels of both MMP-2 and MMP-9 were down-regulated by laver extract treatment in a dose-dependent manner. Laver extract, at 400 μg/mL, was inhibited by MMP-2 and MMP-9 expressions by 70.1 and 77.0%, respectively. An inverse relationship in the mRNA contents of MMP-2/-9 and TIMP-1/-2 expressions in SK-Hep1 cells was found by laver extract treatment. Our results demonstrate antimetastatic properties of laver extract in inhibiting the adhesion, invasion, and migration of SK-Hep1 human hepatoma cancer cells.
Graphical Abstract
Representative photographs show the invading cells laver extract-treated and untreated SK-Human hepatoma cells.
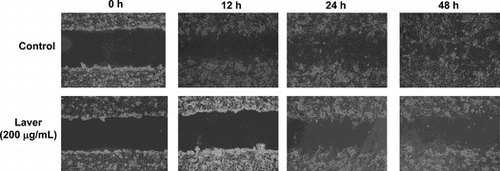
The laver (Porphyra tenera), red seaweed, has been cultivated long time ago in Asian countries, mainly in Korea, Japan, and China, and they are the major laver-producing countries in the world.Citation1) The genus Porphyra, traditionally known as kim in Korea, nori in Japan, and zicai in China, has increased in demand because of the increasing popularity of Oriental cuisine and macrobiotic diets in western countries in recent years.Citation2,3) From a nutritional point of view, lavers are characterized by high concentrations of fibers and minerals,Citation4,5) low fat content, and relatively high protein levels.Citation6,7) Laver is considered a rich source of antioxidants, such as polyphenols, flavonoids, chlorophyll, and carotenoids, that may have synergistic effects on antioxidant potential.Citation8−12) Laver may provide an excellent source of bioactive compounds such as antioxidants, dietary fiber, essential fatty acids, vitamins, and minerals.Citation6,7) Regular consumption of seaweeds, including laver, increases the intake of dietary fiber and lowers the occurrence of some chronic diseases such as diabetes, obesity, heart diseases, inflammation, and cancer.Citation13−15) Several studies have reported that seaweed extracts lower the risks of human breast, rectal, and mammary cancers with suppressive effects.Citation16−18)
Cancer metastasis consists of several sequential steps: cancer cell adhesion, invasion, proliferation, and migration.Citation19) Many studies have demonstrated that inhibition of these steps results in the prevention of metastasis. The matrix metalloproteinases (MMPs) are extracellular matrix-degrading enzymes and elevated levels of individual MMPs have been positively correlated with invasive and metastatic potential in various human tumor cell lines.Citation20) In particular, MMP-2 (gelatinase A, 72 kDa) and MMP-9 (gelatinase B, 92 kDa) degrade the type IV collagen, the major components of the basement membrane, and are strongly implicated in invasion, by metastasis of malignant tumors.Citation21,22) Therefore, inhibition of MMP activity is an important strategy for inhibiting cancer cell invasion and consequently prevention of the metastasis. MMP activities are regulated via suppression by tissue inhibitors of metalloproteinases (TIMPs). TIMPs form a complex with MMPs and inhibit any potential proteolytic activity in vitro, as well as in vivo.Citation20,23) Therefore, overexpression of TIMPs may inhibit cancer cell growth and metastasis in various cancers.Citation24)
In the present study, we determined the antimetastatic effect of laver extract in SK-Hep1 cells using migration and invasion assays. We also investigated the relationship of MMP-2/-9 and TIMP-1/-2 expression at both the protein and the gene level in SK-Hep1 human hepatoma carcinoma cells after laver extract treatment.
Materials and methods
Samples and chemicals
Dried laver (P. tenera) produced in Wando, Korea was purchased from a local market in Korea. Due to the hygroscopic nature of laver, it was stored in air-tight plastic bags until analysis. Folin-Ciocalteu’s phenol reagent, gallic acid, naringin, 2,2-diphenyl-1-picrylhydrazyl radical (DPPH), 2,2′-azino-bis-3-ethylbenzothiazoline-6-sulphonic acid (ABTS), fetal bovine serum (FBS), 3-(4,5-dimethylthiazolyl-2)-2,5-diphenyl tetrazolium bromide (MTT), and RNase A were obtained from Sigma Chemical Co. (St. Louis, MO, USA). Dulbecco’s modified Eagle’s medium (DMEM), trypsin-EDTA, and penicillin/streptomycin were from Gibco Life Technologies, Inc. (Paisley, UK). Cell culture supplies were purchased from Costar (Corning, Inc., Cypress, CA, USA). Organic solvents were purchased from Burdick & Jackson (Batavia, IL, USA). All reagents and chemicals used were of analytical grade.
Extraction of laver
We used food-grade solvents such as water and different percentages of aqueous ethanol due to safety concerns regarding the use of some organic solvent extracts in food. In a preliminary study, we found that 70% ethanol extraction at 37°C was good based on extraction yield and antioxidant activity. Thus, we used 70% ethanol as extraction solvents for further study. The dried laver was cut into small pieces, weighed (~20 g), and 20-fold water was added to the sample and extracted for 4 h at 37°C. The mixture was centrifuged at 5,000 × g for 20 min, and the upper layer was moved to another tube. The extraction was performed three times. Solvent fractions were combined and evaporated to dryness in a vacuum evaporator (EYELA 400 series, Tokyo, Japan). The extracts were freeze-dried in a freeze-drier (Ilshin, Seoul, Korea), finely ground with a cooled mortar and pestle to a particle size < 1.0 mm, and kept in air-tight plastic bags at –20°C until analysis. All determinations were performed at least in triplicate and data are reported on a dry weight basis as mean ± standard deviation (SD).
Determination of total phenolic and flavonoid contents
The laver extracts were dissolved in deionized water (1 mg/mL) and used to determine total polyphenol contents using the Folin–Denis methodCitation25) with some modifications. Briefly, 0.2 mL extract was mixed with 0.4 mL 10% 2 N Folin–Ciocalteu’s phenol reagent and allowed to react for 3 min at room temperature, after which 0.8 mL 10% Na2CO3 solution was added. The mixture was kept in the dark at room temperature for 1 h, and then absorbance was measured at 750 nm with a microplate reader (Infinite M200 Pro, Tecan Group Ltd, San Jose, CA). Gallic acid was used to calculate the standard curve (6.25–100 μg/mL). The results are expressed in milligrams of gallic acid equivalents (GAE)/gram dry weight.
Total flavonoid content was measured using the method of Woisky and SalatinoCitation26) with slight modifications. Ethanol extracts of laver were dissolved in water (1 mg/mL). An appropriately diluted 100 μL sample was mixed with 500 μL distilled water and 30 μL 5% NaNO2 and allowed to react for 6 min at room temperature. The mixture was added to 10% aluminium chloride (60 μL) and allowed to react for 6 min at room temperature. A 200 μL aliquot of 1 M NaOH and 110 μL distilled water was added. The mixture was kept at 25°C for 40 min, and then absorbance was measured at 415 nm with a microplate reader (Infinite M200 Pro). Total flavonoid content was calculated as catechin equivalents (CE) using calibration curves prepared with quercetin standard solutions over a concentration range of 6.25–100 μg/mL. Results are expressed in milligrams of CE/gram dry weight.
Determination of chlorophyll and carotenoid
The chlorophyll and carotenoid contents of the laver were measured using the method of Lichtenthaler and Buschmann.Citation27) Freeze-dried powdered samples (20 mg) were mixed with 5 mL of DMSO and incubated at 65°C for 6 h. After incubation the mixture was centrifuged at 15,000 g for 5 min. The supernatant was collected and the absorbance was read at 663 nm and 647 nm for chlorophylls and at 470 nm for carotenoid content. The concentration for chlorophyll and carotenoid were calculated by following equation.
Chlorophyll a = 12.25A663 – 2.79A647
Chlorophyll b = 21.50A647 – 5.10A663
Total chlorophyll = 20.29A647 + 8.02A663
Carotenoid = (1,000A470 – 1.82 Chlorophyll a − 95.15 Chlorophyll b)/225
Extraction and quantification of β-carotene
Dried laver powder (~500 mg) was weighed, 50 mL ethyl acetate was added to the mixture, followed by vortex mixing for 1 min. The mixture was centrifuged for 5 min, and the upper ethyl acetate layer was removed to another glass tube. The aqueous layer was extracted with another 50 mL of ethyl acetate. The extraction was performed 3–4 times, until laver extracts were colorless. Ethyl acetate fractions were combined and evaporated to dryness in a vacuum evaporator. The residue was redissolved in 0.25 mL of diethyl ether and 0.75 mL of HPLC mobile phase (methanol/acetonitrile/ tetrahydrofuran (THF), 50:45:5, v/v/v) to a total volume of 1.0 mL. The β-carotene was determined by isocratic HPLC (Waters 2695, Waters Co., Milford, MA) on C18 Novapak column (3.9 × 150 mm, 5 μm particle size, Waters Co., Milford, MA) with a mobile phase of methanol, acetonitrile, and THF (50:45:5, v/v/v) at 25°C, at a flow rate of 1 mL/min. The reconstituted samples were placed in autosampler vials, and a 10 μL volume was injected. The peaks were detected by a Waters 2487 Programmable Multiwavelength Detector (Waters, MA) at 450 nm.Citation28)
Extraction and quantification of polyphenol by using HPLC
Powdered samples were weighed to be ~500 mg. Then, 50 mL of 80% methanol containing solution was added, and the mixture was vortexed for 1 min. The mixture was then centrifuged for 5 min, and the upper fraction of the mixture was moved to another glass tube. The residue was extracted using another 50 mL of 80% methanol. The extraction was performed 3 ~ 4 times, until the extracts were colorless. Methanolic fractions were combined and evaporated until dryness in the rotary evaporator. The residue was redissolved in 80% methanol at an appropriate concentration for HPLC analysis, and a 10 μL volume was injected. Polyphenol levels were analyzed using HPLC (Waters, MA) on an Agilent XDB C18 column (4.6 × 150 mm, 5 μm, Waters Co., Milford, MA). The solvent system used was (A) water with 1.0% formic acid and (B) acetonitrile. The samples were separated according to the following gradient: A/B = 95/5 (0–39 min), 40/60 (40 min), 0/100 (45–50 min), and 95/5 (55–60 min) at a flow rate of 0.8 mL/min. The peaks were detected by a Waters 2487 Programmable Multiwavelength Detector at 280 nm (Waters, MA).
Cell culture
SK-Hep1 human hepatoma carcinoma cells were obtained originally from the Korean Cell Line Bank (Seoul, Korea) and maintained at 37°C with 5% CO2 in DMEM supplemented with 10% FBS, 0.37% NaHCO3, 100 U/mL of penicillin, and 100 μg/mL of streptomycin.
Cell proliferation assay
Proliferation was determined by MTT assay. To evaluate the effect of laver extract on cell viability, cells were seeded at a density of 5 × 103 cells per well containing 200 μL of culture medium in 96-well plates. After cultivation for 24 h, the medium was changed to 200 μL of fresh medium supplemented with different concentration of laver extract (0–500 μg/mL). After cultivation for 24–48 h, entire medium was removed and cell viability was evaluated as follows: MTT was dissolved in phosphate-buffered saline (PBS) at 5 mg/mL and filtered to sterilize and remove small amounts of insoluble residue. A mixture of 10 μL of the MTT stock solution and 90 μL of phenol red and serum-free medium was added to all the wells of the 96-well plate culture. After the plates were incubated for 4 h at 37°C, DMSO was added to the wells and mixed by pipetting to dissolve the dark-blue formazan product. The absorbance of the dissolved formazan in each well was measured with a microplate spectrophotometer (Infinite M200 Pro) at 570 nm.
Cell adhesion assay
The 24-well tissue culture plates were coated with 25 μg/well of Matrigel and left to air-dry for 40 min. Cells (5 × 104) suspended in DMEM that contained 0.5% BSA, in the presence and the absence of different concentrations of laver extract, were dispensed into each well of the 24-well culture plate, incubated in 5% CO2 at 37°C for 2 h, and gently washed three times with PBS to remove the unattached cells. The cells that remained attached to the bottom of the plate were stained with hematoxylin and eosin, and counted under a microscope (Olympus I×70; Olympus, Okaya, Japan).
Cell invasion assay
A Transwell system that incorporated a polycarbonate filter membrane with a diameter of 6.5 mm and pore size of 8 μm (Corning, Corning, NY) was used to assess the rate of cell invasion. Matrigel (12.5 μg in 50 μL of PBS) was added to the filter to form a thin gel layer, dried in a laminar hood overnight, and reconstituted in 100 μL of PBS at 37°C for 2 h. Cells at 90% confluency were harvested using a cell dissociation solution. The cells (1 × 105) were suspended in 100 μL of FBS-free DMEM in the presence and the absence of different concentrations of laver extract, and added to the upper chamber of the Transwell insert. The lower chamber was filled with 500 μL of the same medium as the upper chamber. After 18 h of incubation at 37°C, the cells on the upper surface of the filter were removed using a cotton swab. Cells that penetrated to the lower surface of the filter were stained with hematoxylin and counted under an Olympus Ix70 microscope in 13 randomized fields at 400× magnification.
Wound migration assay
Cells were cultured up to 100% confluency in a 6-well plate at different concentrations of laver extract for 18 h. The cells were pretreated with mitomycin C (25 μg/mL) for 30 min before the injury line was made by applying a plastic pipette tip to the cells across the center of the well, producing a clean 1-mm wide wound area. After rinsing with PBS, the cells were allowed to migrate in the medium. A computer-based microscopy imaging system was used to determine wound healing at 0 h with a microscope at 200× magnification. At the indicated time-points, migration was assessed by counting the number of cells across the wound. This number was then compared to the same frame at 0 h. Several wound areas (at least five per plate) were quantified for cell migration. The migration distances between the leading edge of the migrating cells and the edge of the wound were compared. Migration rate = (migration distances of sample-treated cells/migration distances of untreated cells) × 100%.
Gelatin zymography
The MMP activities were determined using previously described methods.Citation29) The proteins were separated on a non-reducing 10% SDS-PAGE containing 1 mg/mL of gelatin. After electrophoresis, the gel was washed two times with 2.5% Triton X-100 for 30 min to remove SDS, and was subsequently incubated in buffer containing 50 mM Tris-HCl (pH 7.6), 200 mM NaCl, and 5 mM CaCl2 for 18 h at 37°C. The gel was stained with 0.5% Coomassie Blue for 1 h and then destained in water containing 10% glacial acetic acid and 30% methanol until clear bands appeared against a blue background. The intensity of the clear bands was quantitated using ImageJ software (version 1.47) (NIH, Bethesda, MD).
Isolation of total RNA and semiquantitative RT-PCR
Total RNA from the SK-Hep1 cells was isolated using RNeasy mini kit (Qiagen Inc., Valencia, CA) with manufacturer’s instruction. The concentration and purity of total RNA were calculated on the basis of the absorbance at 260 and 280 nm. First-strand cDNA was synthesized with 1 μg of total RNAs and 1 μM oligo (dT15) primer using Omniscript Reverse Transcriptase (Qiagen). Using the Taq PCR Master Mix kit (Qiagen), subsequent PCR as performed with 0.5 μL of first-strand cDNA and 20 pmol of primers. PCR consisted of an initial denaturation at 94°C for 3 min; 30 three-step cycles at 94°C for 1 min, 60°C for 1 min, and 72°C for 1 min; and a final extension at 72°C for 10 min. The amplified PCR products were loaded onto a 1.0% agarose gel. After ethidium bromide staining, the gel was illuminated on a UV transilluminator and quantified DNA using molecular imager (BioRad Inc., Waltham, MA, USA). Primers were synthesized at the Bioneer Inc. (Daejeon, Korea) and primer sequences used are listed in Table .
Table 1. Primer sequences used in this study.
Statistical analysis
All the results are presented as mean ± SD values. Statistical analysis was performed using the statistical analysis system (SPSS software package, Version 17.0). Data were compared using one-way analysis of variance, and p < 0.05 was considered significant.
Results
Determination of total phenolic, flavonoid, chorophyll, and carotenoid contents
The total phenolic, flavonoid, chlrophyll, and carotenoid contents of the laver samples are presented in Table . The polyphenolic and flavonoid compounds in 70% ethanol extract were 28.72 mg GAE/g extract and 1.25 mg CE/g extract, respectively. Laver contained 23.84 mg of total chlorophylls and the concentration of chlorophyll a was higher than chlorophyll b; 17.48 mg and 4.12 mg/g extract for chlorophyll a and b, respectively. The concentration of total carotenoid was 5.76 mg in g extract.
Table 2. Concentration of total phenolic, flavonoid, chlorophyll, and carotenoid contents.
Extraction and quantification of β-carotene and polyphenol by using HPLC
The concentration of β-carotene in laver was determined by HPLC with retention time of 10.9 min. As shown in Table , the average concentration of β-carotene in laver was 64.02 μg/g dry weight. We detected four phenolic compounds: catechin hydrate, chlorogenic acid, vanillic acid, and quercetin, which had retention times of 7.9, 8.3, 8.9, and 30.3 min, respectively, in laver extract by HPLC analysis. Chlorogenic acid was one of the major phenolic compound with 66.96 μg/g dry weight of laver. The average concentration of vanillic acid, quercetin, and catechin hydrate in laver extract (in g dry weight) were 30.30, 24.42, and 12.66 μg, respectively.
Table 3. Concentration of β-Carotene and Polyphenol Contents (μg/g) in laver extract measured by HPLC.
Cell proliferation
Fig. illustrates the dose-dependent effect of 0–400 μg/mL of laver extract on the growth of SK-Hep1 human hepatoma cancer cells after 24 or 48 h incubation. After 24 h incubation, laver extract inhibited the proliferation of SK-Hep1 cells in a dose-dependent manner. The numbers of cells were significantly reduced by 5.2–88% at laver extract concentrations of 0–400 μg/mL as compared to the control. Similarly, after 48 h of incubation, 200 μg/mL of laver extract reduced the cell numbers by 61 and 71%, respectively, and laver extract in dose-dependent manner inhibited the SK-Hep1 cell proliferation.
Cell adhesion
To determine the effects of laver extract on the adhesion of SK-Hep1 cells, adhesive cells were quantitated using an ELISA reader. Incubation of SK-Hep1 cells with 0–400 μg/mL of laver extract for 2 h significantly inhibited cell adhesion to the Matrigel-coated substrate in a concentration-dependent manner (Fig. ). Approximately 12.5 and 23.2% reduction was seen under the 200 and 400 μg/mL laver extract treatments, respectively.
Cell invasion
We further evaluated the antimetastatic activity of laver extract using the Transwell assay. We tested the ability of SK-Hep1 cells to invade through a reconstituted basement membrane barrier (Matrigel) with and without laver extract. Similar to its effects in the adhesion assay, laver extract in the range of 0–400 μg/mL inhibited the invasion of SK-Hep1 cells in a dose-dependent manner. When SK-Hep1 cells were grown on Matrigel, treatment with 100–400 μg/mL laver extracts for 18 h produced a significant reduction in the number of invasive cells compared to the control (fresh medium alone). The level of invasion was reduced to 5.9% of the control level at 100 μg/mL laver extract (Fig. ). Laver extract showed significant 19.6% and 27.2% inhibition of cancer cell invasion at 200 and 400 μg/mL, respectively, compared to the control.
Cell migration
To evaluate the antimetastatic activity of laver extract, we assessed the effect of laver extract on the migration of SK-Hep1 cells on wound migration assay. The migration distance between the leading edge and the wound line was compared between laver extract-treated cells and untreated cells. As shown in Fig. , cellular motility was controlled by 200 μg/mL of laver extract in a time-dependent manner, with up to 87 and 72% motility at 24 and 48 h of incubation, respectively (p < 0.001).
MMP and TIMP expression
We determined the inhibitory effects of laver extract on MMP production by zymography in SK-Hep1 cells. The SK-Hep1 cells constitutively secreted high levels of MMP-9 and low levels of MMP-2. Gelatin zymography of conditioned medium demonstrated that the SK-Hep1 cells secreted a 62-kD protein with gelatinolytic activity, corresponding to the activated MMP-2. As shown in Fig. , gelatinolytic activity in the zymogram was inhibited by laver extract in a dose-dependent manner. Laver extract at 200 μg/mL and higher concentrations clearly suppressed MMP-2 activity, and MMP-9 activity was inhibited in a dose-dependent manner by 0–400 μg/mL laver extract. Thus, in the SK-Hep1 cell system, both MMP-2 and MMP-9 were down-regulated by laver extract treatment.
Fig. 6. Expression of MMP-2/-9 mRNA in SK-Hep1 cells after treatment with laver extract for 24 h, as measured by RT-PCR.
Note: Values marked * and ** indicate significant differences from control (p < 0.05).
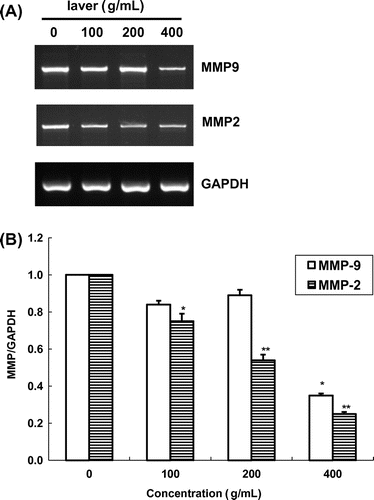
We also quantified MMP-2/-9 mRNA expression in SK-Hep1 cells by RT-PCR with an internal standard (GAPDH) (Fig. ). In the presence of laver extract, MMP-9 and MMP-2 activities were reduced in a dose-dependent manner. MMP-9 expression was inhibited by 24.7 and 62.1% at 100 and 200 μg/mL of laver extract, respectively, as compared to the control. MMP-2 mRNA expression was also inhibited at all doses of laver extract used in this study, with 22.3 and 55.2% inhibition after a 24 h exposure to 100 and 200 μg/mL of laver extract, respectively. Laver extract at 400 μg/mL was inhibited MMP-2 and MMP-9 express by 70.1 and 77.0%, respectively.
Fig. 7. Expression of TIMP-1/-2 mRNA in SK-Hep1 cells after treatment with laver extract for 24 h, as measured by RT-PCR.
Note: Values marked * and ** indicate significant differences from control (p < 0.05).
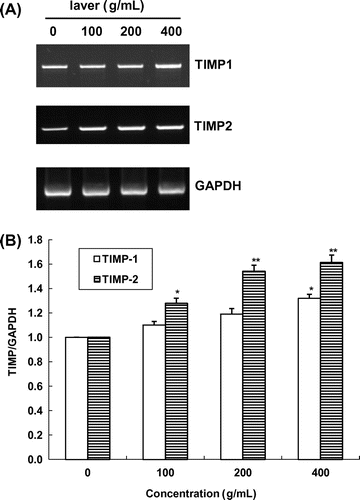
The effects of 24 h exposure to laver extract on TIMP-1/-2 activities in SK-Hep1 cells are shown in Fig. . In the presence of laver extract, TIMP-1 and TIMP-2 activities were increased in a dose-dependent manner. TIMP-1 expression was increased by 10.1 and 19.4% at 100 and 200 μg/mL laver extract, respectively, as compared to the control. TIMP-2 mRNA expression was also increased at all doses of laver extract used in this study, with 44.7 and 61.8% increases after a 24 h exposure to 100 and 200 μg/mL of laver extract, respectively. Laver extract at 400 μg/mL increased TIMP-1 and TIMP-2 expression by 41.8% and 83.7%, respectively. We found that there was an inverse relation in mRNA contents between MMP-2/-9 and TIMP-1/-2 expression in SK-Hep1 cells by laver extract treatment.
Discussion
Laver is considered a rich source of antioxidants, such as several phenolic compounds and these may contribute on anticancer activity. We determined total phenolic and flavonoid contents, as well as several individual phenolic compounds: catechin hydrate, chlorogenic acid, vanillic acid, and quercetin. The reported phenolic contents vary among seaweed species and extraction solvents. Cho et al.Citation30) reported that the total phenolic contents of a crude extract of green seaweed, Enteromorpha prolifera, ranged from 46.2 to 80.4 mg GAE/g, which were considerably higher than our results (28.72 mg GAE/g extract). The red alga P. urceolata contains 73.7 GE/g phenolic content in the ethyl acetate fraction.Citation31) The brown seaweed Papenfussiella kuromo contains only 0.18 mg CAE/g in ethanol extractsCitation32) but another brown seaweed Sargassum ringgoldianum showed 383.0 mg CE/g dry weight in 50% ethanol extracts.Citation33) Devi et al.Citation34) reported that the in vitro antioxidant activities of several seaweeds and the total phenol concentration of methanol and ethyl ether extracts of T. conoides were 1.23 and 1.19 mg GAE/g, respectively. Relatively less flavonoid contents were obtained in laver extract and there was not much comparable data regarding the flavonoid contents in seaweeds.
Syad et al.Citation35) determined chlrophyll contents in red alga, Gelidiella acerosa and Sargassum wightii and reveals that about 1.58–6.56 μg/g fresh weight of chlorophyll a and 1.90–3.76 μg/g FW of chlorophyll b. Chlorophyll possesses excellent antioxidant activity and the possible mechanism of action behind its antioxidant activity might be either the protection of linoleic acid against oxidation or prevention of the decomposition of hydroperoxides.Citation36)
Several studies reported that seaweed extracts or the specific component have been reported to show anticancer activity,Citation12−14), Citation37) but the mechanisms of cancer inhibition have not been clearly elucidated. In our present study, we demonstrated that laver extract at a rate more than 100 μg/mL inhibits cancer cell proliferation, adhesion, invasion, and migration in SK-Hep1 human hepatoma cancer cells. It also provided evidence that the mechanism underlying the above effects was related to the inhibition of MMP-2/-9 activities and increased TIMP-1/-2 expression. Several in vitro and in vivo studies have shown that seaweed extract inhibits the growth of human cancer cells. Namvar et al.Citation38) showed that edible red seaweed Eucheuma cottonii inhibited the growth of both estrogen-dependent MCF-7 and estrogen-independent MB-MDA-231 human breast cancer cells. Also they found that E. cottonii extract (0–300 mg/kg) inhibited tumor incidence (87.5% in control vs. 12.5% in 300 mg/kg in E. cottonii extract) and tumor volume (10.7 cm3 in control and 0.9 cm3 in 300 mg/kg E. cottonii extract) in the cancer-induced rats. Athukorala et al.Citation39) reported that enzyme (4-α-D-glucosidase) hydrolyzed extract of Ecklonia cava (EC) showed strong inhibition of several cell proliferation such as CT-26 murine colon cancer cells, THP-1 and U-937 human leukemia cells, and B-16 mouse melanoma cells. Especially, crude polyphenolic fractions showed 5.1 μg/mL of IC50 value on CT-26 murine colon cancer cells.
Our results indicate that laver extract decreased the metastasis of SK-Hep1 cells, as determined by adhesion, invasion, and migration assays. To define the inhibitory effects of laver extract on metastasis, we focused on MMP expression, because degradation of the ECM is required for tumor metastasis. We evaluated MMP-2/-9 expression because the main component of the basement membrane, type IV collagen, is degraded by collagenase, and several reports have indicated a correlation between decreased MMP-2/-9 expression and metastatic potential in various cancer cells. As found in the current study, laver extract suppressed the secretion of MMP-2/-9 into the culture medium as well as the expression of MMP-2/-9 mRNA and protein.
Several studies have reported that seaweed extract inhibits cancer cell invasion. Kim et al.Citation40) reported that methanolic extract of brown seaweed, Sragassum thunbergii, led to the reduction of the expression levels of MMP-2/-9 in zymography and confirmed in both mRNA and protein levels in HT1080 cells. Kim et al.Citation41) showed that 95% ethanol extract of EC (10–50 μg/mL) inhibited both MMP-2 and MMP-9 activities. Treatment of 10 μg/mL of EC extract inhibited MMP-2 and MMP-9 activities by 80% and 85%, respectively, in fluorometric analysis. In addition, artificially induced activities of MMP-2 and MMP-9 in human dermal fibroblasts and HT1080 cells were inhibited by EC extracts in gelatin zymography. The EC extract showed greater inhibition of MMP-2 activity than that of MMP-9 activity and complete inhibition of MMP-2 activity was observed at 500 μg/mL.
In conclusion, laver extract inhibits cell proliferation, adhesion, invasion, and migration in SK-Hep1 human hepatoma cancer cells. The antimetastatic action of laver extract was related to the inhibition of MMP-2/-9 activities and increased TIMP-1/-2 expression.
Supplemental material
The supplemental material for this paper is available at http://dx.doi.org/10.1080/09168451.2014.912116.
Supplemental Fig. 1 caption
Download MS Word (13.3 KB)Supplemental Fig. 1
Download MS Power Point (2.8 MB)References
- Nisizawa K, Noda H, Kikuchi R, Watanabe T. The main seaweeds in Japan. Hydrobiologia. 1987;151–152:5–29.10.1007/BF00046102
- Sahoo D, Tang X, Yarish C. Porphyra-the economic seaweed as a new experimental system. Curr. Sci. 2002;83:1313–1316.
- FAO. Year book of fishery statistics 2005. Vol. 100-1/2. Rome: Food and Agricultural Organization; 2007.
- Burtin P. Nutritional value of seaweeds. Elect. J. Environ. Agric. Food Chem. 2003;2:498–503.
- Bocanegra A, Bastida S, Benedí J, Ródenas S, Sánchez-Muniz FJ. Characteristics and nutritional and cardiovascular-Health properties of seaweeds. J. Med. Food. 2009;12:236–258.10.1089/jmf.2008.0151
- Galland-Irmouli AV, Fleurence J, Lamghari R, Luçon M, Rouxel C, Barbaroux O, Bronowicki JP, Villaume C, Guéant JL. Nutritional value of proteins from edible seaweed Palmaria palmata (Dulse). J. Nutr. Biochem. 1999;10:353–359.10.1016/S0955-2863(99)00014-5
- Sánchez-Machado DI, López-Cervantes J, López-Hernández J, Paseiro-Losada P. Fatty acids, total lipid, protein and ash contents of processed edible seaweeds. Food Chem. 2004;85:439–444.10.1016/j.foodchem.2003.08.001
- Ito K, Hori K. Seaweed: Chemical composition and potential uses. Food Rev. Int. 1989;5:101–144.10.1080/87559128909540845
- Wong KH, Cheung PCK. Nutritional evaluation of some subtropical red and green seaweeds. Part I-proximate composition, amino acid profiles and some physico-chemical properties. Food Chem. 2000;71:475–482.10.1016/S0308-8146(00)00175-8
- Li K, Li XM, Ji NY, Wang BG. Bromophenols from the marine red alga Polysiphonia urceolata with DPPH radical scavenging activity. J. Nat. Prod. 2008;71:28–30.10.1021/np070281p
- Duan XJ, Li XM, Wang BG. Chemical constituents of the red alga Symphyocladia latiuscula. J. Nat. Prod. 2007;70:1210–1213.10.1021/np070061b
- Cho S, Kang S, Cho J, Kim A, Park S, Hong Y, Ahn D. The antioxidant properties of brown seaweed (Sargassum siliquastrum) extracts. J. Med. Food. 2007;10:479–485.10.1089/jmf.2006.099
- Dawczynski C, Schubert R, Jahreis G. Amino acids, fatty acids, and dietary fiber in edible seaweed products. Food Chem. 2007;103:891–899.10.1016/j.foodchem.2006.09.041
- Kazłowska K, Hsu T, Hou C, Yang W, Tsai G. Anti-inflammatory properties of phenolic compounds and crude extract from Porphyra dentata. J. Ethnopharmacol. 2010;128:123–130.10.1016/j.jep.2009.12.037
- Kim YC, An RB, Yoon NY, Nam TJ, Choi JS. Hepatoprotective constituents of the edible brown alga Ecklonia stolonifera on tacrine-induced cytotoxicity in HepG2 cells. Arch. Pharm. Res. 2005;28:1376–1380.10.1007/BF02977904
- Kato I, Tominaga S, Matsuura A, Yoshii Y, Shirai M, Kobayashi S. A comparative case-control study of colorectal cancer and adenoma. Cancer Sci. 1990;81:1101–1108.10.1111/cas.1990.81.issue-11
- Eskin BA, Grotkowski CE, Connolly CP. Different tissue responses for iodine and iodide in rat thyroid and mammary glands. Biol. Trace Elem. Res. 1995;49:9–19.10.1007/BF02788999
- Funahashi H, Imai T, Mase T, Sekiya M, Yokoi K, Hayashi H, Shibata A, Hayashi T, Nishikawa M, Suda N, Hibi Y, Mizuno Y, Tsukamura K, Hayakawa A, Tanuma S. Seaweed prevents breast cancer? Cancer Sci. 2001;92:483–487.10.1111/cas.2001.92.issue-5
- Yilmaz M, Christofori G, Lehembre F. Distinct mechanisms of tumor invasion and metastasis. Trends Mol. Med. 2007;13:535–541.10.1016/j.molmed.2007.10.004
- Deryugina EI, Quigley JP. Matrix metalloproteinases and tumor metastasis. Cancer Metast. Rev. 2006;25:9–34.10.1007/s10555-006-7886-9
- John A, Tuszynski G. The role of matrix metalloproteinases in tumor angiogenesis and tumor metastasis. Pathol. Oncol. Res. 2001;7:14–23.10.1007/BF03032599
- Klein G, Vellenga E, Fraaije MW, Kamps WA, de Bont ES. The possible role of matrix metalloproteinase (MMP)-2 and MMP-9 in cancer. Crit. Rev. Oncol./Hematol. 2004;50:87–100.10.1016/j.critrevonc.2003.09.001
- Chakraborti S, Mandal M, Das S, Mandal A, Chakraborti T. Regulation of matrix metalloproteinases: an overview. Mol. Cell. Biochem. 2003;253:269–285.10.1023/A:1026028303196
- Suminoe A, Matsuzaki A, Hattori H, Koga Y, Ishii E, Hara T. Expression of matrix metalloproteinase (MMP) and tissue inhibitor of MMP (TIMP) genes in blasts of infant acute lymphoblastic leukemia with organ involvement. Leukemia Res. 2007;31:1437–1440.10.1016/j.leukres.2007.01.015
- Zhou K, Su L, Yu L. Phytochemicals and antioxidant properties in wheat bran. J. Agric. Food Chem. 2004;52:6108–6114.10.1021/jf049214g
- Woisky R, Salatino A. Analysis of propolis: some parameters and procedures for chemical quality control. J. Apicul. Res. 1998;37:99–105.
- Lichtenthaler HK, Buschmann C. Current protocols in food analytical chemistry (CPFA), (Supplement 1). Wiley, New York (2001).
- Hwang ES, Stacewicz-Sapuntzakis M, Bowen PE. Effects of heat treatment on the carotenoid and tocopherol composition of tomato. J. Food Sci. 2012;77:C1109–C1114.10.1111/jfds.2012.77.issue-10
- Hwang E, Lee HJ. Benzyl isothiocyanate inhibits metalloproteinase-2/-9 expression by suppressing the mitogen-activated protein kinase in SK-Hep1 human hepatoma cells. Food Chem. Toxicol. 2008;46:2358–2364.10.1016/j.fct.2008.03.016
- Cho M, Lee H, Kang I, Won M, You S. Antioxidant properties of extract and fractions from Enteromorpha prolifera, a type of green seaweed. Food Chem. 2011;127:999–1006.10.1016/j.foodchem.2011.01.072
- Duan XJ, Zhang WW, Li XM, Wang BG. Evaluation of antioxidant property of extract and fractions obtained from a red alga, Polysiphonia urceolata. Food Chem. 2006;95:37–43.10.1016/j.foodchem.2004.12.015
- Kuda T, Tsunekawa M, Goto H, Araki Y. Antioxidant properties of four edible algae harvested in the Noto Peninsular. J. Food Compos. Anal. 2005;18:625–633.10.1016/j.jfca.2004.06.015
- Nakai M, Kageyama N, Nakahara K, Miki W. Phlorotannins as radical scavengers from the extract of Sargassum ringgoldianum. Mar. Biotechnol. 2006;8:409–414.10.1007/s10126-005-6168-9
- Devi GK, Manivannan K, Thirumaran G, Rajathi FAA, Anantharaman P. In vitro antioxidant activities of selected seaweeds from southeast coast of India. Asia-Pac. J.Trop. Med. 2011;26:205–211.
- Syad AN, Shunmugiah KP, Kasi PD. Seaweeds as nutritional supplements: Analysis of nutritional profile, physicochemical properties and proximate composition of G. acerosa and S. wightii. Biomed. Prev. Nutr. 2013;3:139–144.10.1016/j.bionut.2012.12.002
- Shetty K. Role of proline-linked pentose phosphate pathway in biosynthesis of plant phenolics for functional food and environmental applications: a review. Process Biochem. 2004;39:789–803.10.1016/S0032-9592(03)00088-8
- Yuan YV, Walsh NA. Antioxidant and antiproliferative activities of extracts from a variety of edible seaweeds. Food Chem. Toxicol. 2006;44:1144–1150.10.1016/j.fct.2006.02.002
- Namvar F, Mohamed S, Fard SG, Behravan J, Mustapha NM, Alitheen NBM, Othman F. Polyphenol-rich seaweed (Eucheuma cottonii) extract suppresses breast tumor via hormone modulation and apoptosis induction. Food Chem. 2012;130:376–382.10.1016/j.foodchem.2011.07.054
- Athukorala Y, Kim KN, Jeon YJ. Antiproliferative and antioxidant properties of an enzymatic hydrolysate from brown alga, Ecklonia cava. Food Chem. Toxicol. 2006;44:1065–1074.10.1016/j.fct.2006.01.011
- Kim JA, Kong CS, Seo YW, Kim SK. Sargassum thunbergii extract inhibits MMP-2 and -9 expressions related with ROS scavenging in HT1080 cells. Food Chem. 2010;120:418–425.10.1016/j.foodchem.2009.10.022
- Kim M-M, Ta QV, Mendis E, Rajapakse N, Jung W-K, Byun H-G, Jeon Y-J, Kim S-K. Phlorotannins in Ecklonia cava extract inhibit matrix metalloproteinase activity. Life Sci. 2006;79:1436–1443.10.1016/j.lfs.2006.04.022