Abstract
Fasting for 3 days leads to reduction in the expression of GLUT5 and SGLT1 genes in jejunum. Re-feeding a high-sucrose diet in fasted rats enhanced mRNA levels and histone H3 acetylation on transcribed region of GLUT5 gene within 24 h, but not in SGLT1. Responsiveness of jejunal GLUT5 gene is associated with changes in histone H3 acetylation on transcribed region.
Longer starvation reduces the functions of the small intestine by reduction of villus height along with reduced expression of proteins related to the nutrients digestion and absorption. It has been reported that enzyme activity of sucrase, which digests sucrose to glucose and fructose and contributes digestion of starch hydrolytic products, is gradually reduced until day 3 of starvation.Citation1) In addition, re-feeding a high-sucrose diet did not enhance sucrase activity until 24 h in starved rats,Citation2) although the activity under a non-fasted condition responded to high-sucrose diet within 3 h.Citation3) The dietary regulation of sucrase activity occurs at the mRNA transcription level.Citation3,4) These results indicate that fasting for 3 days in rats reduces responsiveness of jejunum sucrase–isomaltase gene to a high-sucrose diet. However, it is unclear whether hexose transporters in the small intestine maintain the responsiveness to dietary factors after 3 days of starvation. It has been reported that jejunal expression of glucose transporter 5 (GLUT5) and sodium/glucose cotransporter member 1 (SGLT1) genes in non-starved rats is rapidly induced by the force-feeding fructose or sucrose.Citation5) Recent studies from this laboratory have demonstrated that the induction of SGLT1 and GLUT5 genes by feeding a high-carbohydrate diet is associated with acetylation of histone H3 and H4 around these genes, in particular on transcribed region of the genes.Citation6,7) Histone acetylation, one of the epigenetic memories written on the chromatin, contributes to gene activation by modulating chromatin structure and recruiting coactivator proteins.Citation8) In particular, histone H3 acetylation is important for the transcriptional regulation because the acetylation of the histone H3 starts to transcription.Citation9) In this study, we examined whether the expressions of GLUT5 and SGLT1 genes are altered by re-feeding a high-sucrose diet following 3 days of starvation in rats, and whether the responsiveness of these genes depends on a change in histone acetylation around these genes.
Six-weeks-old male Sprague-Dawley rats (Japan SLC Inc., Hamamatsu, Japan) were used for the experiment in an air-conditioned room at a temperature of 23 ± 2 °C and a humidity of 55 ± 5% with a light–dark cycle (7:00–19:00). The rats had free access to a control diet containing 63% (w/w) corn starch, 20% casein, 7% corn oil, 5% cellulose, 3.5% minerals, 1% vitamins, 0.3% L-cystine, and 0.2% choline bitartrate (AIN-93G)Citation10), and water for 7 days, and they were fasted for 3 days. In re-fed groups, the animals were fasted for 3 days and then re-fed a high-sucrose diet containing 40% (w/w) sucrose, 23% corn starch, 20% casein, 7% corn oil, 5% cellulose, 3.5% minerals, 1% vitamins, 0.3% L-cystine, and 0.2% choline bitartrate for 12, 18, or 24 h. Control rats were given free access to the control diet. All rats had unrestricted access to water throughout the experimental period. Rats were killed by decapitation between 10:00 and 11:00. The experimental procedures used in the present study conformed to the guidelines of the Animal Usage Committee of the University of Shizuoka. Blood was collected from jugular vein. The entire small intestine was removed and the jejunoileum was divided into two segments of equal lengths. A 0.5-cm segment was excised from the middle region of the jejunal loop, and was immediately used for RNA extraction. The remaining part of the jejunal loop was used for the chromatin immunoprecipitation (ChIP) assay. Serum concentrations of glucose, triacylglycerols, and free fatty acids were determined by commercial kits (Wako Pure Chemical Industries, Osaka, Japan). Total RNA was extracted by the acidified guanidine thiocyanate methodCitation11) and converted to cDNA by reverse transcription using SuperscriptTM III reverse transcriptase (Life Technologie, CA, USA). To quantitatively estimate the mRNA levels of GLUT5 and SGLT1 genes, PCR amplification was performed on Light-Cycler instrument (Roche Molecular Biochemicals, Bavaria, Germany). PCR primers used were 5′-CGGACGTATATCCGGAGAAGGA-3′ and 5′-AGCAGGTGGGAGGTCATTAAGC-3′ for GLUT5, 5′-TGTACCCTGTGTGGCT-3′ and 5′-CGTGCCGCAGTGTTTC-3′ for SGLT1, and 5′-GGTGCATGGCCGTTCTTA-3′ and 5′-TCGTTCGTTATCGGAATTAACC-3′ for 18S rRNA. The cycle threshold (CT) values for each gene and 18S rRNA detected by real-time RT-PCR were converted to signal intensities by delta–delta method.Citation12) For ChIP assays, the mucosa collected from the jejunal loop was incubated with fixation solution, and following a wash in FACS solution, the samples were sonicated in SDS lysis buffer in a condition where the fragment size of DNA ranges 200–500 bp as previously described.Citation13) Chromatin immunoprecipitation was performed using 1 μg anti-acetyl-histone H3 antibody (Millipore, MA, USA) or 1 μg normal IgG. The precipitated DNA was subjected to real-time PCR with primers complementary to sites in the promoter/enhancer and transcribed region of GLUT5 gene (at −1900, −1100, −500, −100, +2000, and +5000 bp relative to the transcription start site) and SGLT1 gene (at −2000, −1000, −500, +1, +2000, and +5000 bp relative to the transcription start site). The CT values of ChIP and input signals detected by real-time PCR were converted to signal intensities by the delta–delta method.Citation12) All ChIP signals were normalized to the corresponding input signals.
Table 1. Body weight, intestinal weight, and blood biochemical parameters in starved and re-fed rats.
Fig. 1. Effects of starvation and re-feeding a high-sucrose diet on the mRNA levels of GLUT5 and SGLT1 genes and histone H3 acetylation around their genes in rat jejunum.
Note: (A) mRNA. (B) Histone H3 acetylation. Each value is expressed as means ± standard error of the mean for 6–7 rats per group. The comparisons between control and fasted groups were analyzed by Student’s t-test, and comparisons among fasted and each re-fed group were analyzed by Dunnett’s based on one-way ANOVA.
*Indicate significant differences compared with control group. #Indicate significant differences compared with starved group. #p < 0.05, **, ##p < 0.01.
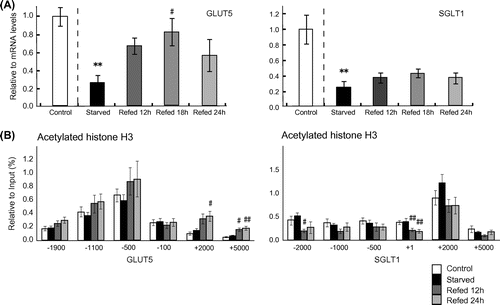
The jejunal and ileal weights were significantly lower in the rats fasted for 3 days than in control rats (Table ). The jejunal weight was recovered within 12 h after re-feeding to the level of non-fasted rats, however, the ileal weight was not fully recovered to the non-fasting level even at 24 h after re-feeding. This is consistent with a previous study, which showed that re-feeding for 24 h after 3 days of starvation led to a significant increase in protein content in the proximal segment, but not in middle and distal segments of jejunoileum of rats.Citation1) The mRNA levels of GLUT5 and SGLT1 genes in the jejunum were significantly reduced after 3 days of starvation. By re-feeding with a high-sucrose diet, the mRNA levels of GLUT5 gene, but not SGLT1 gene, were significantly elevated within 24 h (Fig. (A)). To the best of our knowledge, this is the first report that demonstrates that expression of jejunal GLUT5 gene is altered by re-feeding a high-sucrose diet in starved animals within 12 h. It should be pointed out that previous studies showed that dietary sucrose or fructose enhanced the expression of jejunal GLUT5 gene to a greater extent than SGLT1 and SI genes.Citation5,14) Thus, it is very likely that responsiveness of GLUT5 gene to dietary sucrose is maintained even after 3 days of fasting. In this study, we have demonstrated that re-feeding a high-sucrose diet enhances histone H3 acetylation on GLUT5 gene, but not on SGLT1 gene (Fig. (B)). Previously many studies have demonstrated that histone H3 acetylation is involved in transcription by recruiting the transcriptional complex on target genes.Citation15,16) Interestingly, we found that significant enhancement of histone H3 acetylation occurred not on promoter/enhancer region, but on transcribed region of the GLUT5 gene. Previous studies have reported that histone acetylation on transcribed region of genes enhances transcription elongation reaction by recruiting positive transcription elongation factor b (P-TEFb).Citation17) The results in this study suggest that responsiveness of GLUT5 gene in fasted rats is attributable to the readiness of transcription elongation reaction associated with induction of histone H3 acetylation on transcribed region of the gene. It should be noted that histone H3 acetylation around GLUT5 gene was not significantly reduced after 3 days of fasting. This result indicates that a reduction of GLUT5 mRNA levels in starved rats is not concerned with changes of histone H3 acetylation. A possible regulation of mRNA degradation process should be examined in further works. It should be noted that histone acetylation around the transcription initiation site and enhancer region of the SGLT1 gene are rather reduced by re-feeding a high-sucrose diet. This result suggests that acetylation levels of histone H3 are differently regulated between SGLT1 and GLUT5 genes in the animals re-fed a high-sucrose diet after starvation. It should be examined in further works whether recruitment of histone deacetylase is different between SGLT1 and GLUT5 genes. In conclusion, re-feeding a high-sucrose diet in fasted rats leads to induction of jejunal GLUT5 gene, but not SGLT1, which is associated with changes in histone H3 acetylation on the transcribed region of the gene.
Acknowledgments
This work was supported by a Grant-in-Aid for Scientific Research (23300276) and for challenging Exploratory Research (24650458) from the Ministry of Education, Culture, Sports, Science and Technology of Japan.
References
- Yamada K, Goda T, Bustamante S, Koldovsky O. Different effect of starvation on activity of sucrase and lactase in rat jejunoileum. Am. J. Physiol. 1983;244:G449–G455.
- Ulshen MH, Grand RJ. Site of substrate stimulation of jejunal sucrase in the rat. J. Clin. Invest. 1979;64:1097–1102.10.1172/JCI109548
- Kishi K, Takase S, Goda T. Enhancement of sucrase-isomaltase gene expression induced by luminally administered fructose in rat jejunum. J. Nutr. Biochem. 1999;10:8–12.10.1016/S0955-2863(98)00071-0
- Goda T, Yasutake H, Tanaka T, Takase S. Lactase-phlorizin hydrolase and sucrase-isomaltase genes are expressed differently along the villus-crypt axis of rat jejunum. J. Nutr. 1999;129:1107–1113.
- Kishi K, Tanaka T, Igawa M, Takase S, Goda T. Sucrase-isomaltase and hexose transporter gene expressions are coordinately enhanced by dietary fructose in rat jejunum. J. Nutr. 1999;129:953–956.
- Inoue S, Mochizuki K, Goda T. Jejunal induction of SI and SGLT1 genes in rats by high-starch/low-fat diet is associated with histone acetylation and binding of GCN5 on the genes. J. Nutr. Sci. Vitaminol. 2011;57:162–169.10.3177/jnsv.57.162
- Honma K, Mochizuki K, Goda T. Inductions of histone H3 acetylation at lysine 9 on SGLT1 gene and its expression by feeding mice a high carbohydrate/fat ratio diet. Nutrition. 2009;25:40–44.10.1016/j.nut.2008.07.006
- Roh TY, Cuddapah S, Zhao K. Active chromatin domains are defined by acetylation islands revealed by genome-wide mapping. Genes Dev. 2005;19:542–552.10.1101/gad.1272505
- Yan C, Boyd DD. Histone H3 acetylation and H3 K4 methylation define distinct chromatin regions permissive for transgene expression. Mol. Cell. Biol. 2006;26:6357–6371.10.1128/MCB.00311-06
- Reeves PG, Nielsen FH, Fahey GC Jr. AIN-93 purified diets for laboratory rodents: final report of the American Institute of Nutrition ad hoc writing committee on the reformulation of the AIN-76A rodent diet. J. Nutr. 1993;123:1939–1951.
- Chomczynski P, Sacchi N. Single-step method of RNA isolation by acid guanidinium thiocyanate-phenol-chloroform extraction. Anal. Biochem. 1987;162:156–159.10.1016/0003-2697(87)90021-2
- Livak kJ, Schmittgen TD. Analysis of relative gene expression data using real-time quantitative PCR and the 2(-Delta Delta C(T)) Method. Methods. 2001;25:402–408.10.1006/meth.2001.1262
- Honma K, Mochizuki K, Goda T. Carbohydrate/fat ratio in the diet alters histone acetylation on the sucrase-isomaltase gene and its expression in mouse small intestine. Biochem. Biophys. Res. Commun. 2007;357:1124–1129.10.1016/j.bbrc.2007.04.070
- Honma K, Mochizuki K, Goda T. Induction by fructose force-feeding of histone H3 and H4 acetylation at their lysine residues around the Slc2a5 gene and its expression in mice. Biosci. Biotechnol. Biochem. 2013;77:2188–2191.10.1271/bbb.130300
- Soutoglou E, Talianidis I. Coordination of PIC assembly and chromatin remodeling during differentiation-induced gene activation. Science. 2002;295:1901–1904.10.1126/science.1068356
- Wang H, Cao R, Xia L, Erdjument-Bromage H, Borchers C, Tempst P, Zhang Y. Purification and functional characterization of a histone H3-lysine 4-specific methyltransferase. Mol. Cell. 2001;8:1207–1217.10.1016/S1097-2765(01)00405-1
- Jang MK, Mochizuki K, Zhou M, Jeong HS, Brady JN, Ozato K. The bromodomain protein Brd4 is a positive regulatory component of P-TEFb and stimulates RNA polymerase II-dependent transcription. Mol. Cell. 2005;19:523–534.10.1016/j.molcel.2005.06.027