Abstract
Bioluminescence is a chemical reaction process for light emission in vivo. An organic substance is normally oxidized in the protein to obtain the energy required for the light emission. Determination of the structure of the substance is one of the most important parts of bioluminescent research. Photoproteins of a flying squid and a mollusk contain chromophores that are formed by connecting an apo-protein and dehydrocoelenterazine. The chromophore has a chemical structure that can emit light in a photoprotein. The structural analysis of the chromophores in the photoproteins is described.
Graphical Abstract
Photoproteins of a flying squid and a mollusk contain chromophores that are formed by connecting an apo-protein and dehydrocoelenterazine (DCL).
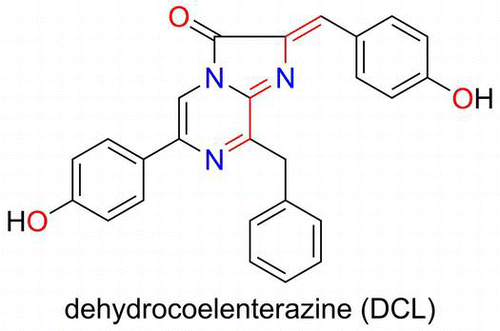
Bioluminescence is a chemical reaction process in vivo for light emission. The energy is normally obtained by the oxidation of a specific substance. The oxidation produces an intermediate (peroxide) which is decomposed to produce an excited singlet state of a light emitter. This reaction efficiently proceeds in a protein. Some bioluminescence requires biological cofactors, such as ATP, Ca2+, reactive oxygen species (ROS), etc.
There are two kinds of proteins that are involved in a bioluminescence reaction; i.e. a photoprotein and luciferase. The representative photoprotein is aequorin which was isolated from a bioluminescent jellyfish (Aequoria aequoria) by Shimomura.Citation1) This protein has a prosthetic group (chromophore) in the active site, and the chromophore is a peroxide of coelenterazine (CL) (Fig. ).Citation2–4) The chromophore decomposes to emit blue light when a calcium ion binds to the EF-hand of aequorin.Citation5,6) The light intensity is proportional to the molecular numbers of aequorin. Aequorin has been called a photoproteinCitation2) to discriminate it from luciferase of the firefly. Luciferase is an enzyme which catalyzes the oxidation of luciferin (the substrate of luciferase) for light emission. The resulting light intensity is proportional to the molecular numbers of luciferin.Citation7)
Fig. 2. 13C-NMR spectra of model chromophore.
Notes: 13C-labeled DCL reacted with DTT to form the model chromophore. The 13C-labeled sp2 carbon was observed at 135.3 ppm. The signal shifted to 45.4 and 45.2 ppm at the sp3 carbon when the chromophore was formed. Due to the chiral centers of DTT, the chromophore was observed as a mixture of diastereomers. The spectra were recorded in CD3OD.
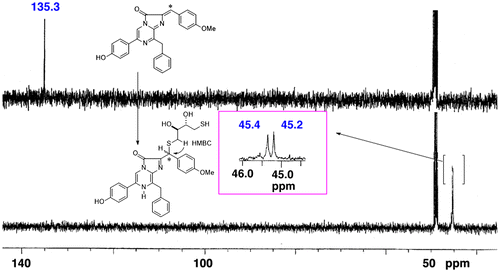
Fig. 3. Mass spectra of the peptides of the chromophore of symplectin.
Notes: The CGLK peptide bound to F-DCL formed the chromophore of symplectin. The signal was observed at m/z 422.78 (M + 2H)2+. The T43 peptide was produced by proteolytic digestion of symplectin with trypsin. The oxidized chromophore was observed at m/z 416.77 (M + 2H)2+ by losing carbon dioxide.
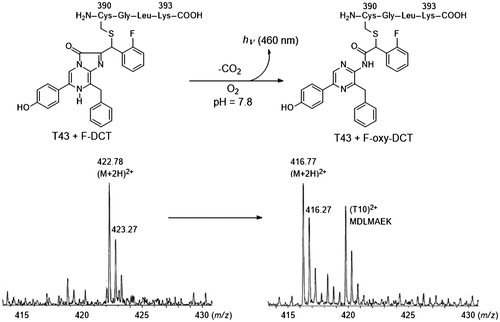
Fig. 4. Preparation of DCL–DTT from the chromophore of pholasin and the LC–MS spectra of extracts obtained from pholasin after being treated with DTT.
Notes: There is an equilibrium between the chromophore of pholasin and DCL. The chromophore was converted to the DCL–DTT adduct by the addition of an excess amount of DTT. The DCL–DTT adduct was extracted from a pholasin solution (upper scheme). (A)–(D) LC–MS spectra of extracts obtained from pholasin and (E)–(H) from the authentic DCL–DTT adduct. (A), (E) Chromatogram monitored by fluorescence (530 nm emission). (B), (F) Ion-chromatogram with m/z 576, which corresponded to the molecular weight of the DCL–DTT adduct. (C), (G) Mass spectra of the ion peak at around 40 min. (D), (H) Mass spectra of the same ion peak (around 40 min) when acetonitrile/D2O was used as the mobile phase for the HPLC.
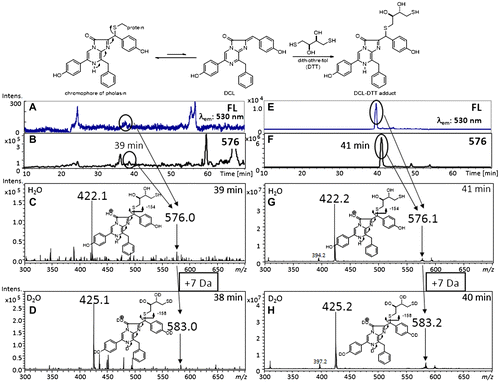
Light energy produced from aequorin is postulated to be absorbed on a green fluorescent protein (GFP) for light emission in Aequorea.Citation8) The GFP is a light emitting protein which was discovered from Aequorea aequorea by Shimomura.Citation9) The fluorescent chromophore of GFP is automatically formed in a living cell without any other cofactor.Citation10) Currently, three kinds of light-emitting proteins are known, i.e. a photoprotein, luciferase, and GFP. In this report, the fundamental research regarding the chromophores in photoproteins of the glowing mollusk and squid is discussed.
I. Connection between dehydrocoelenterazine and symplectin
Dehydrocoelenterazine (DCL) was isolated from the firefly squid (Watasenia scintillans) and its structure was elucidated as the oxidized form of CL by Inoue et al. in 1977 (Fig. ).Citation11) The function of DCL in the squid has been unknown for a long time. Takahashi and Isobe isolated a chemiluminescent compound from the flying squid (Symplectoteuthis oualaniensis) when trying to determine the organic substance responsible for its bioluminescence. The compound was deduced as an acetone adduct of DCL, which was an artifact during the extraction of organic compounds from the luminescent organ using acetone (Fig. ).Citation12)
The flying squid is around 30 cm long and has a light organ on its mantle. The organ contains thousands of small granules, in which a photoprotein exists as the active form. Tsuji et al. reported that the luminescence of the homogenate of the luminescent organ in 0.6 M KCl buffer required monovalent cations such as Na+ and K+.Citation13) The optimum pH for the luminescence was pH 7.8 and the peak wavelength of the luminescence was 456 nm.Citation13) Takahashi and Isobe later demonstrated that DCL is the required organic substance for the bioluminescence of the photoprotein (called symplectin) by the fact that an intense light was produced from the homogenate of the luminous organ only when DCL was mixed.Citation14) They reported that the acetone adduct was derived by forming a Shiff’s base produced from the amino residue of a protein and acetone.Citation14) By the fact that only cysteine formed an active luminescent compound by connecting to DCL, they proposed that a chromophore of symplectin must be formed by binding a sulfhydryl residue of cysteine with DCL at the active site of symplectin (Scheme ).Citation14)
Scheme 1. Bioluminescence mechanism of symplectin.
Notes: DCL reacts with a cysteine in the active site to form the chromophore of symplectin. Oxidation of the chromophore affords the oxidized chromophore with light emission. The asterisk indicates the 13C-labeled carbon.
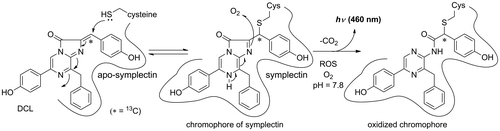
Symplectin was extracted from the light organs using 0.6 M KCl buffer at pH 6.0. Symplectin was a fluorescent protein and was composed of 500 amino acids (the molecular weight of symplectin was 60 kDa).Citation15) Symplectin has eleven cysteines on its amino acid sequence.Citation16) One of the residues of cysteines was therefore postulated to form the chromophore for the luminescence of symplectin. The structure of the chromophore was then investigated especially focusing on the carbon atom which was bound to the sulfhydryl residue at the active site of symplectin. A 13C-labeling strategy was employed because the sp2-carbon in DCL changed to a sp3-carbon when the chromophore was formed. The difference between the sp2-carbon and sp3-carbon could be easily monitored by 13C-NMR spectroscopy. A novel synthetic route was established to install the 13C-atom into DCL.Citation17,18) The formation of the chromophore was then investigated using dithiothreitol (DTT) as a model symplectin to establish a method for the isolation of the model chromophore. The compound was proved to be stable under acidic conditions and isolatable. The NMR spectra of the chromophore were obtained, and the structure of the chromophore demonstrated that the sulfhydryl group was certainly connected to the 13C-labled carbon atom of DCL (Fig. ).Citation17,18) The HMBC analysis of the chromophore also supported the covalent bond between the 13C-labeled carbon and sulfhydryl residue.Citation17,18) The model chromophore was an active luminescent compound and emitted blue light when it was treated with oxygen in alkaline DMSO. The signal of the oxidized chromophore was also observed in the NMR spectra.Citation17,18) Although this was a model study of the bioluminescent mechanism of symplectin, the proposed mechanism was partially demonstrated (Scheme ).
The binding site of the chromophore was then investigated. A serious problem arose during the identification of the binding site. The chromophore was stable as symplectin, but easily decomposed by releasing DCL when symplectin was digested by a protease to obtain a peptide containing the chromophore. The problem was caused by equilibrium between the chromophore and DCL. A fluorine atom was then installed to stabilize the chromophore. The fluorinated DCL (F-DCL) was proved to be an active substance for symplectin, and the chromophore was stable even under neutral conditions.Citation19) By using the F-DCL, peptides were obtained by proteolytic digestion of the reconstituted symplectin, which was prepared from apo-symplectin and F-DCL. The resulting peptides were then analyzed by LC–MS, and a molecular ion was observed at m/z 422.5 as a bivalent ion of the peptide which contained the chromophore. The peptide was formed from the DCL and CGLK-peptide of symplectin. After bioluminescence of the reconstituted symplectin, the molecular ion was shifted to m/z 417.5 by releasing carbon dioxide. Based on these results, cysteine-390 of symplectin was proved to be the binding site that forms the chromophore of symplectin (Fig. ).Citation15,16) During these analyses, the most active DCL analog (2,4-diF-DCL) was prepared. The 2,4-difluoro-DCL was then utilized to analyze the stereochemistry of the chromophore.Citation20)
II. Pholasin, the photoprotein of a bivalve mollusk
A bivalve mollusk (Pholas dactylus) lives off the coast of southwest England. This glowing mollusk digs a hole in a rock and spends its whole life in the hole. The mollusk has luminescent organs, presumably to catch food by glowing at night. A scientific research was initiated since the discovery of the luciferin–luciferase reaction by DuBois in 1887.Citation21) Seventy years later, the Michelson group extensively studied the bioluminescent system and then succeeded in the isolation of luciferin and luciferase.Citation22–24) The luciferin was called pholasin (photoprotein), which was a glycoprotein and had a chromophore in the active site. The luciferase was a copper-containing peroxidase and an glycoprotein with the molecular weight 310 kDa.Citation25,26) The luciferase oxidized luciferin (pholasin) in the presence of hydrogen peroxide and molecular oxygen in order to emit light. Their extensive studies revealed the components required for the chemiluminescence of pholasin without the luciferase.Citation24)
Reichl’s group in 2000 reported that a mixture of peroxidase and hydrogen peroxide strongly activated the luminescence of pholasin.Citation27,28) In the same year, Campbell’s group reported the cloning and expression of pholasin.Citation29) The Knight Scientific Company farms the mollusk and supplies Pholasin® as an indicator for ROS.Citation30,31) Pholasin is now widely used as an ROS indicator in various fields of research.Citation32–37) Although the bioluminescent research of pholasin has a long history, the structure of the chromophore still remained unknown. Therefore, a cloned and expressed pholasin could not be activated due to the lacking of an appropriate substance for the formation of the chromophore.Citation29) Based on the fluorescent and UV/Vis spectra, Campbell’s group proposed that a flavin-type compound might be the chromophore of pholasin.Citation38)
The luminescence of the Symplectoteuthis photoproteins is strongly activated by the addition of a mixture of catalase and hydrogen peroxide.Citation39) A similar initiation of luminescence between symplectin and pholasin prompted us to form the hypothesis that the chromophore of pholasin also consisted of apo-pholasin and DCL. To prove this hypothesis, we initiated the determination of the chromophore of pholasin. Initially, the effect on the luminescence of pholasin by the addition of DCL was investigated. The simple addition of DCL to pholasin intensified the luminescence of pholasin. Based on this result, DCL was proved to be a substance needed for the luminescence of pholasin.Citation40) However, there remained the possibility that DCL worked as a mimic of the real substance of pholasin. We then tried to isolate DCL itself from pholasin. If the chromophore had a structure similar to the chromophore in symplectin, it would be impossible to extract DCL directly from the solution of pholasin. As was the case with symplectin, we first tried to convert the chromophore into the acetone adduct of DCL. Mixing pholasin with acetone did not afford any acetone adduct of DCL. We then tried to convert the chromophore into the DTT adduct of DCL by mixing pholasin with DTT in methanol (Fig. ). A fluorescent compound was successfully extracted from pholasin with methanol. The structure of the extract was definitely identified as the DCL–DTT adduct by LC–MS (Fig. ).Citation41) Isobe’s LC–MS and MS/MS analysis combined with counting the exchangeable protons was a powerful technique to identify the trace amounts of natural products using deuterium oxide as an eluent.Citation42–47)
Although the structure of the chromophore of pholasin has long been unknown, we finally concluded that DCL was the organic substance of pholasin, based on these results that DCL was active for the luminescence of pholasin and the DCL–DTT adduct was isolated from the native pholasin.
III. Conclusion
Bioluminescence is a chemical reaction process that occurs in living cells. This luminescence requires a protein and a substrate (or an organic substance). Some bioluminescence system requires cofactors such as ATP, Ca2+, and ROS. Oxygen is also typically required for the bioluminescence. This review described the fundamental research which focused on the structure of the chromophores in symplectin and pholasin of the glowing squid and mollusk. DCL binds to the residue of cysteine-390 at the active site of symplectin to form the chromophore. The active site of symplectin was demonstrated by using F-DCL and LC–MS. The chromophore of pholasin was converted to the DCL–DTT adduct, which was identified by LC–MS. Although the chromophore of pholasin had long been unknown, we finally demonstrated that DCL was the organic substance of pholasin.
Acknowledgment
I would express my gratitude to Emeritus Professor Minoru Isobe (Nagoya University), Toshio Nishikawa (Nagoya University), and Hirosato Takikawa (Kobe University) for their continuous encouragement and for the many fruitful discussions on this subject. The above-mentioned studies were supported by a Grant-in-Aid for Scientific Research from the Japan Society for the Promotion of Science (JSPS), and from the Ministry of Education, Culture, Sports, Science and Technology (MEXT) of Japan. I also thank the Japan Society for Bioscience, Biotechnology, and Agrochemistry for the Japan Society for Bioscience, Biotechnology, and Agrochemistry Society Award for the Encouragement of Young Scientists.
Notes
This review was written in response to the author’s receipt of JSBBA Award for Young Scientists in 2013.
References
- Shimomura O, Johnson FH, Saiga Y. Extraction, purification and properties of aequorin, a bioluminescent protein from the luminous hydromedusan, Aequoria. J. Cell Comp. Physiol. 1962;59:223–239.10.1002/(ISSN)1553-0809
- Shimomura O, Johnson FH. Bioluminescence in progress. In: Johnson FH, Haneda Y, editors. Princeton, NJ: Princeton University Press; 1966. p. 495–521.
- Shimomura O, Johnson FH, Morise H. Mechanism of the luminescent intramolecular reaction of aequorin. Biochemistry. 1974;13:3278–3286.10.1021/bi00713a016
- Inoue S, Sugiura S, Kakoi H, Hasizume K, Goto T, Iio H. Squid bioluminescence II. Isolation from Watasenia Scintillans and synthesis of 2-(p-hydroxybenzyl)-6-(p-hydroxyphenyl)-3,7-dihydroimidazo[1,2-a]-pyrazin-3-one. Chem. Lett. 1975;141–144.10.1246/cl.1975.141
- Head JF, Inouye S, Teranishi K, Shimomura O. The crystal structure of the photoprotein aequorin at 2.3A resolution. Nature. 2000;405:372–376.10.1038/35012659
- Jones K, Hibbert F, Keenan M. Glowing jellyfish, luminescence and a molecule called coelenterazine. Trends Biotechnol. 1999;17:477–481.10.1016/S0167-7799(99)01379-7
- Nakatsu T, Ichiyama S, Hiratake J, Saldanha A, Kobashi N, Sakata K, Kato H. Structural basis for the spectral difference in luciferase bioluminescence. Nature. 2006;440:372–376.10.1038/nature04542
- Morise H, Shimomura O, Johnson FH, Winant J. Intermolecular energy transfer in the bioluminescent system of Aequoria. Biochemistry. 1974;13:2656–2662.10.1021/bi00709a028
- Shimomura O. Discovery of green fluorescent protein (GFP) (Nobel Lecture). Angew. Chem. Int. Ed. 2009;48:5590–5602.10.1002/anie.v48:31
- Nishiuchi Y, Inui T, Nishio H, Bodi J, Kimura T, Tsuji FI, Sakakibara S. Chemical synthesis of the precursor molecule of the Aequorea green fluorescent protein, subsequent folding, and development of fluorescence. Proc. Nat. Acad. Sci. USA. 1998;95:13549–13554.10.1073/pnas.95.23.13549
- Inoue S, Taguchi H, Murata M, Kakoi H, Goto T. Squid bioluminescence IV. Isolation and structural elucidation of Watasenia dehydropreluciferin. Chem. Lett. 1977;6:259–262.10.1246/cl.1977.259
- Takahashi H, Isobe M. Symplectoteuthis bioluminescence. (1). Structure and binding form of chromophore in photoprotein of a luminous squid. Bioorg. Med. Chem. Lett. 1993;3:2647–2652.10.1016/S0960-894X(01)80734-4
- Tsuji FI, Leisman GB. K+/Na+ -triggered bioluminescence in the oceanic squid Symplectoteuthis oualaniensis. Proc. Nat. Acad. Sci. USA. 1981;78:6719–6723.10.1073/pnas.78.11.6719
- Takahashi H, Isobe M. Photoprotein of luminous squid, Symplectoteuthis oualaniensis and reconstruction of the luminous system. Chem. Lett. 1994;23:843–846.10.1246/cl.1994.843
- Fujii T, Ahn JY, Kuse M, Mori H, Matsuda T, Isobe M. A novel photoprotein from oceanic squid (Symplectoteuthis oualaniensis) with sequence similarity to mammalian carbon–nitrogen hydrolase domains. Biochem. Biophys. Res. Commun. 2002;293:874–879.10.1016/S0006-291X(02)00296-6
- Isobe M, Kuse M, Tani N, Fujii T, Matsuda T. Cysteine-390 is the binding site of luminous substance with symplectin, a photoprotein from Okinawan squid, Symplectoteuthis oualaniensis. Proc. Jpn Acad. Ser. B. 2008;84:386–392.10.2183/pjab.84.386
- Isobe M, Kuse M, Yasuda Y, Takahashi H. Synthesis of 13C-dehydroeoelenterazine and model studies on Symplectoteuthis squid bioluminescence. Bioorg. Med. Chem. Lett. 1998;8:2919–2924.10.1016/S0960-894X(98)00525-3
- Kuse M, Isobe M. Synthesis of 13C-dehydrocoelenterazine and NMR studies on the bioluminescence of a Symplectoteuthis model. Tetrahedron. 2000;56:2629–2639.10.1016/S0040-4020(00)00163-0
- Isobe M, Fujii T, Kuse M, Miyamoto K, Koga K. 19F-dehydrocoelenterazine as probe to investigate the active site of symplectin. Tetrahedron. 2002;58:2117–2126.10.1016/S0040-4020(02)00100-X
- Kongjinda V, Nakashima Y, Tani N, Kuse M, Nishikawa T, Yu CH, Harada N, Isobe M. Dynamic chirality determines critical roles for bioluminescence in symplectin–dehydrocoelenterazine system. Chem. Asian J. 2011;6:2080–2091.10.1002/asia.201100089
- Dubois R. Fonction photogenique chez le Pholas dactylus. Compt. Rend. Soc. Biol. 1887;39:564–566.
- Henry JP, Michelson AM. Studies in bioluminescence. VIII. Chemically induced luminescence of Pholas dactylus luciferin. Biochimie. 1973;55:75–81.10.1016/S0300-9084(73)80239-1
- Henry JP, Isambert MF, Michelson AM. Studies in bioluminescence. IX. Mechanism of the Pholas dactylus system. Biochimie. 1973;55:83–93.10.1016/S0300-9084(73)80240-8
- Michelson AM. Purification and properties of Pholas dactylus luciferin and luciferase. Methods Enzymol. 1978;57:385–406.10.1016/0076-6879(78)57037-7
- Henry JP, Monny C, Michelson AM. Characterization and properties of Pholas luciferase as metalloglycoprotein. Biochemistry. 1975;14:3458–3466.10.1021/bi00686a026
- Henry JP, Monny C. Protein-protein interaction in the Pholas dactylus system of bioluminescence. Biochemistry. 1977;16:2517–2525.10.1021/bi00630a031
- Reichl S, Arnhold J, Knight J, Schiller J, Arnold K. Reactions of pholasin with peroxidases and hypochlorous acid. Free Radical Biol. Med. 2000;28:1555–1563.10.1016/S0891-5849(00)00268-9
- Reichl S, Vocks A, Petković M, Schiller J, Arnhold J. The photoprotein pholasin as a luminescence substrate for detection of superoxide anion radicals and myeloperoxidase activity in stimulated neutrophils. Free Radical Res. 2001;35:723–733.10.1080/10715760100301231
- Dunstan SL, Sala-Newby GB, Fajardo AB, Taylor KM, Campbell AK. Cloning and expression of the bioluminescent photoprotein pholasin from the bivalve mollusc Pholas dactylus. J. Biol. Chem. 2000;275:9403–9409.10.1074/jbc.275.13.9403
- Roberts PA, Knight J, Campbell AK. Pholasin - a bioluminescent indicator for detecting activation of single neutrophils. Anal. Biochem. 1987;160:139–148.10.1016/0003-2697(87)90624-5
- Knight Scientific Limited. Abel® antioxidant test kit. Available from: http://www.knightscientific.com/kits
- Müller T, Davies EV, Campbell AK. Pholasin chemiluminescence detects mostly superoxide anion released from activated human neutrophils. J. Biolumin. Chemilumin. 1989;105–113.
- Cotton B, Allshire A, Cobbold PH, Müller T, Campbell AK. Pholasin: a novel bioluminescent probe for monitoring oxidative stress in single cardiomyocytes. Biochem. Soc. Trans. 1989;17:705–706.
- Swindle EJ, Hunt JA, Coleman JW. A comparison of reactive oxygen species generation by rat peritoneal macrophages and mast cells using the highly sensitive real-time chemiluminescent probe pholasin: inhibition of antigen-induced mast cell degranulation by macrophage-derived hydrogen peroxide. J. Immunol. 2002;169:5866–5873.10.4049/jimmunol.169.10.5866
- Glebska J, Koppenol WH. Chemiluminescence of pholasin caused by peroxynitrite. Free Radical Biol. Med. 2005;38:1014–1022.10.1016/j.freeradbiomed.2004.12.028
- Jaffar NZ, Dan Z, Christopher S, John BD, Jan K, John H. The use of Pholasin® as a probe for the determination of plasma total antioxidant capacity. Clin. Biochem. 2006;39:55–61.10.1016/j.clinbiochem.2005.09.011
- Sild E, Hõrak P. Assessment of oxidative burst in avian whole blood samples: validation and application of a chemiluminescence method based on pholasin. Behav. Ecol. Sociobiol. 2010;64:2065–2076.10.1007/s00265-010-1076-z
- Müller T, Campbell AK. The chromophore of pholasin: a highly luminescent protein. J. Biolumin. Chemilumin. 1990;5:25–30.
- Shimomura O. Bioluminescence, chemical principal and methods, revised edition. In: Shimomura O, editor. Toh Tuck Link: World Scientific Publishing Co. Pte. Ltd; 2012. p. 219–224.
- Kuse M, Tanaka E, Nishikawa T. Pholasin luminescence is enhanced by addition of dehydrocoelenterazine. Bioorg. Med. Chem. Lett. 2008;18:5657–5659.10.1016/j.bmcl.2008.08.113
- Tanaka E, Kuse M, Nishikawa T. Dehydrocoelenterazine is the organic substance constituting the prosthetic group of pholasin. ChemBioChem. 2009;10:2725–2729.10.1002/cbic.v10:17
- Kuse M, Franz T, Koga K, Suwan S, Isobe M, Agata N, Ohta M. High incorporation of L-amino acids to cereulide, an emetic toxin from Bacillus cereus. Bioorg. Med. Chem. Lett. 2000;10:735–739.10.1016/S0960-894X(00)00092-5
- Kuse M, Doi I, Kondo N, Kageyama Y, Isobe M. Synthesis of azide-fluoro-dehydrocoelenterazine analog as a photoaffinity-labeling probe and photolysis of azide-fluoro-coelenterazine. Tetrahedron. 2005;61:5754–5762.10.1016/j.tet.2005.04.025
- Sydnes MO, Doi I, Ohishi A, Kuse M, Isobe M. Determination of solvent-trapped products obtained by photolysis of aryl azides in 2,2,2-trifluoroethanol. Chem. Asian J. 2008;3:102–112.10.1002/(ISSN)1861-471X
- Doi I, Kuse M, Nakashima Y, Tani N, Isobe M. Dfferentiation of coelenteramide sulfates from phosphates by hydrogen/deuterium exchange coupled with ion trap mass spectrometry. J. Mass Spectrom. Soc. Jpn. 2009;57:89–95.10.5702/massspec.57.89
- Doi I, Kuse M, Nishikawa T, Isobe M. Selective protein modification by the hydroperoxide intermediate in a photoprotein, aequorin. Bioorg. Med. Chem. 2009;17:3399–3404.10.1016/j.bmc.2009.03.033
- Kuse M, Yanagi M, Tanaka E, Tani N, Nishikawa T. Identification of a fluorescent compound in the cuticle of the train millipede Parafontaria laminata armigera. Biosci. Biotechnol. Biochem. 2010;74:2307–2309.10.1271/bbb.100171