Abstract
Age-related skin thinning is correlated with a decrease in the content of collagen in the skin. Accumulating evidence suggests that collagen peptide (CP) and vitamin C (VC) transcriptionally upregulate type I collagen in vivo. However, the additive effects of CP and VC on age-related skin changes remain unclear. We herein demonstrate that CP and a VC derivative additively corrected age-related skin thinning via reduced oxidative damage in superoxide dismutase 1 (Sod1)-deficient mice. Co-treatment with these compounds significantly normalized the altered gene expression of Col1a1, Has2, and Ci1, a proton-coupled oligopeptide transporter, in Sod1−/− skin. The in vitro analyses further revealed that collagen oligopeptide, a digestive product of ingested CP, significantly promoted the bioactivity of the VC derivative with respect to the migration and proliferation of Sod1−/− fibroblasts. These findings suggest that combined treatment with CP and VC is effective in cases of age-related skin pathology.
Graphical Abstract
Co-treatment of collagen peptide and vitamin C additively improve aging-like skin atrophy in mice.
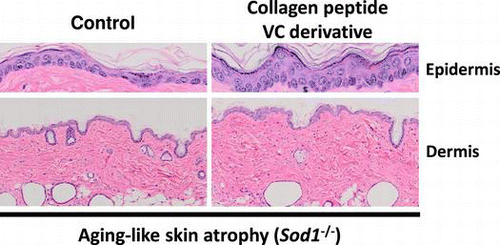
The skin is the largest organ in the body and it plays an essential role in the barrier function in protecting the body from environmental factors such as infection and UV radiation. Since the skin is abundantly composed of collagen fibers, collagen matrix homeostasis plays a central role in maintaining the skin function. In human studies, skin collagen components show age-dependent reductions in both male and female subjects, resulting in age-related skin thinning in older individuals.Citation1) Therefore, preventing age-related collagen declines helps to maintain skin integrity.
When heated, collagen is denatured and becomes water-soluble gelatin. Collagen peptide (CP) prepared via the enzymatic hydrolysis of gelatin is widely used as a food ingredient. Accumulating evidence indicates that the dietary supplementation of CP promotes extracellular matrix biosynthesis in the joints, nails, and hair.Citation2,3) Proksch et al. reported that CP intake increases skin elasticity in elderly females.Citation4) Oral treatment with CP and/or gelatin also increases the type I collagen expression in the bones and skin.Citation5–7) Notably, Sato and colleagues identified that CP ingestion increases collagen oligopeptides (COPs) composed of two or three amino acids, including Ala-Hyp-Gly, Pro-Hyp, Pro-Hyp-Gly, Ile-Hyp, Leu-Hyp, and Phe-Hyp, in human blood,Citation8,9) suggesting that ingested CP is at least, in part, digested and absorbed as COP. Furthermore, Shigemura et al. reported that COPs, such as Pro-Hyp and Hyp-Gly, exhibit bioactivity for skin fibroblasts.Citation10,11) In addition, vitamin C (VC) is a strong antioxidant that regulates the synthesis of collagen, as well as the post-translational modification of collagen by lysyl oxidase as a co-factor.Citation12,13) In fact, VC application upregulates the mRNA levels of collagen types I and III in human skin fibroblasts.Citation14,15) However, it is unclear whether this activity is augmented by simultaneous treatment, thereby modulating the skin function in vivo.
Using an antioxidant gene-targeting strategy to induce redox imbalance in vivo, we previously demonstrated that Sod1 deficiency causes skin thinning associated with collagen dysregulation.Citation16–18) Recently, we further established hairless Sod1−/− double mutant mice in order to avoid variations in skin morphology during the hair cycle (unpublished results). Therefore, double mutant mice constitute a valuable mouse model mimicking age-related skin thinning. In this context, we herein evaluated the hypothesis that treatment with both CP and VC delays skin aging by attenuating oxidative damage. In the present study, we investigated the additive effects of CP and VC on hairless Sod1-deficient skin phenotypes.
Materials and methods
Materials
CP (fish-derived, MW 3000–5000. Nippi, Tokyo, Japan) was used. Two COPs, Hyp-Gly and Pro-Hyp, were purchased from Bachem (Bubendorf, Switzerland). Another six COPs, Ala-Hyp, Ser-Hyp, Leu-Hyp, Ala-Hyp-Gly, Phe-Hyp, and Pro-Hyp-Gly, were purchased from AnyGen (Gwangju, Korea). COP mixture was mixed with the above eight types of COP and used at a final concentration of 50 μM of Hyp-Gly, 5 μM of Ala-Hyp, 5 μM of Ser-Hyp, 100 μM of Pro-Hyp, 5 μM of Leu-Hyp, 5 μM of Ala-Hyp-Gly, 5 μM of Phe-Hyp, and 5 μM of Pro-Hyp-Gly. Casein peptide (Emulup, molecular weight 4600, lipid: 1.0%, protein: 89.0%, water: 4.0%, ash: 4.5%) was purchased from Morinaga Milk Industry Co, LTD. (Tokyo, Japan). The VC derivative (L-ascorbyl 2-phosphate 6-palmitate trisodium salt) was provided by Showa Denko K.K. (Tokyo, Japan). The VC derivative was prepared by additionally conjugating VC with a phosphate group and a long hydrophobic chain to enhance stability and liposolubility.Citation19)
Animals
Sod1−/− mice and Hos:HR-1hr/hr mice were purchased from the Jackson Laboratory (Bar Harbor, ME, USA) and Hoshino Laboratory Animals, Inc. (Ibaraki, Japan), respectively. The Sod1−/− mice were backcrossed with C57BL/6NCrSlc mice (Japan SLC, Shizuoka, Japan) for five or six generations. Hairless Sod1−/− double mutant mice were generated by crossbreeding Hos:HR-1hr/hr with Sod1−/− mice. Genotyping of the Sod1−/− allele was performed using genomic PCR with genomic DNA isolated from the tail tip, as previously reported.Citation20) The animals were housed under a 12-h light/dark cycle and fed ad libitum. The mice were maintained and studied according to protocols approved by the Animal Care Committee of the Tokyo Metropolitan Institute of Gerontology and Chiba University.
Administration of CP and the VC derivative
CP and the VC derivative were co-administered to hairless Sod1−/− and Sod1+/+ mice of both sexes at 17–20 weeks of age for eight weeks. Collagen or casein peptides were dissolved in MilliQ water at 2% (w/w) and orally administered via gavage (0.2 g/kg/day). Following the administration of CP or casein peptides, 0.1% (w/w) solution of the VC derivative (200 μL/mouse) or MilliQ water (200 μL/mouse) was applied onto the back skin once a day at 1:00 pm each weekday. Casein peptide and MilliQ water were used as placebo controls for CP and the VC derivative, respectively. We previously demonstrated that a low dose (0.1%) of the VC derivative, as well as 1.0 or 10% solution, significantly rescued skin atrophy in Sod1−/− mice against a C57BL/6 background.Citation16) The preliminary experiments, however, revealed that the administration of 0.1% of the VC derivative showed limited effects on skin thickness in the hairless Sod1−/− mice (data not shown). The limited effects of the VC derivative on skin thinning may be due to the differences in histological changes in the skin of the hairless Sod1−/− mice compared to that observed in the C57BL/6 Sod1−/− mice. In fact, we found that hairless Sod1−/− mice, but not C57BL/6 Sod1−/− mice, exhibit marked formation of dermal cysts (unpublished results). In this context, we herein applied 0.1% of the VC derivative in order to clarify the additive effects of treatment with CP and the VC derivative on age-related skin atrophy.
Histology
For the histological observation, skin specimens obtained from the backs of the mice were dissected, fixed in a 20% formalin neutral buffer solution (Wako, Osaka, Japan) overnight, embedded in paraffin and sectioned on a microtome at a thickness of 4 μm using standard techniques. Hematoxylin and eosin staining was performed as previously described.Citation21,22) The thickness of the skin tissue (epidermis and dermis) was measured using the Leica QWin V3 image software program (Leica, Germany).
Oxidative stress markers
The back skin tissues of male mice were homogenized with radioimmunoprecipitation assay buffer [150 m M NaCl, 10 m M MTris–HCl, pH7.4, 0.1% SDS, 1% triton X-100, 5 m M EDTA, 1% deoxycholic acid] containing protease inhibitor cocktail (Roche Diagnostics, Tokyo, Japan). The homogenate was centrifuged at 1600 × g for 10 min at 4 °C, and the total supernatant was used for the assay. The malondialdehyde (MDA) level was measured using the TBARS Assay Kit (Cayman Chemical Company, MI, USA) according to the manufacturer’s instructions. In order to measure the 8-isoprostene content, the back skin tissues were homogenized with 0.1 M phosphate buffer, pH 7.4, containing 1 m M of EDTA (Dojindo Laboratories, Kumamoto, Japan) and 50 μg/mL (w/w) of dibutylhydroxytoluene (Wako). The homogenate was centrifuged at 8000 × g for 10 min at 4 °C, and the total supernatant was used for the assay. The 8-isoprostane level was measured using the 8-isoprostane EIA Kit (Cayman Chemical Company) according to the manufacturer’s instructions. The supernatant was also assayed for the protein concentration using the DC Protein Assay Kit (BioRad, Hercules, CA, USA), and the MDA and 8-isoprostane levels were normalized to the protein level.
Quantitative PCR
Total RNA was extracted from back skin using Trizol reagent (Life Technologies Corporation, Carlsbad, CA, USA) according to the manufacturer’s instructions. cDNA was synthesized from 1 μg of total RNA using reverse transcriptase (ReverTra Ace qPCR RT Master MIX, TOYOBO, Osaka, Japan). Real-time PCR was performed on a Mini OpticonTM (Bio-Rad) with the SYBR GREEN PCR Master Mix (Bio-Rad) according to the manufacturer’s instructions. All data were normalized to the level of the housekeeping gene glyceraldehyde-3-phosphate dehydrogenase (Gapdh). The Gapdh data with large variation (± 5-fold change to mean value) were omitted as low RNA quality samples. The following primer sets were used: Gapdh forward, 5ʹ-ATGTGTCCGTCGTGGATCTGA-3ʹ and reverse, 5ʹ-TGCCTGCTTCACCACCTTCT-3ʹ; Col1a1 forward, 5ʹ-CATGTTCAGCTTTGTGGACCT-3ʹ and reverse, 5ʹ-GCAGCTGACTTCAGGGATGT-3ʹ; Mmp2 forward, 5ʹ-TAACCTGGATGCCGTCGT-3ʹ and reverse, 5ʹ-TTCAGGTAATAAGCACCCTTGAA-3ʹ; Has2 forward, 5ʹ-CGGTCGTCTCAAATTCATCTG-3ʹ and reverse, 5ʹ-ACAATGCATCTTGTTCAGCTC-3ʹ; Ki67 forward, 5ʹ-GCTGTCCTCAAGACAATCATCA-3ʹ and reverse, 5ʹ-GGCGTTATCCCAGGAGACT-3ʹ; Pept1 forward, 5ʹ-TCACAGACCACGACCACAAT-3ʹ and reverse, 5ʹ-CCCCGATGATAGCCAAATAA-3ʹ; Pept2 forward, 5ʹ-ATCCTGCAGTGCATTGTGAA-3ʹ and reverse, 5ʹ-CTGCTGCTGTAACCAGGACA-3ʹ; Pht1 forward, 5ʹ-CCGTGTTCTTGGCTCTGATT-3ʹ and reverse, 5ʹ-CCACCAGCTTGTCCTTCAGT-3ʹ; Ci1 forward, 5ʹ-GTTGCGGTGATCCTGATTCT-3ʹ and reverse, 5ʹ-AGCTGAGGCACTGTCTGGTT-3ʹ.
Cell culture
The skin specimens of Sod1−/− neonates obtained at 4–6 days after birth were sterilized with 70% ethanol and dissected into small pieces. The primary dermal fibroblasts were isolated via dissociation in 0.2% collagenase type 2 (Worthington Biochemical Corporation, Lakewood, NJ, USA) at 37 °C for 60 min. The cells were maintained in α-MEM (Life Technologies Corporation) supplemented with 20% FBS (Life Technologies Corporation), 100 units/ml of penicillin (Sigma-Aldrich, MO, USA), and 0.1 mg/mL of streptomycin (Sigma-Aldrich) at 37 °C in a humidified incubator with 5% CO2 and 1% O2 to prevent damage by O2 and to maintain viability of Sod1−/− fibroblasts. During the subsequent experiments, the cells were cultured to investigate cellular phenotypes under a general condition (20% O2).
Outgrowth assay
The back skin was sterilized with 70% ethanol, rinsed with PBS (Takara Bio Inc., Shiga, Japan) and punched out into disks measuring 5 mm in diameter using Dermalpunch (Nipro, Tokyo, Japan). The punched skin disks were placed into a 24-well culture plate (Falcon BD, Franklin Lakes, NJ) and cultured with or without COP or the VC derivative in α-MEM containing 20% FBS, 100 units/ml of penicillin, and 0.1 mg/mL of streptomycin at 37 °C in a humidified incubator with 5% CO2 and 20% O2. The number of outgrowth fibroblasts originating from the mouse skin disks was directly counted at 96 h after culture.
In vitro scratch wound assay
Fibroblasts were preincubated with or without COP or the VC derivative in α-MEM containing 20% FBS, 100 units/mL of penicillin and 0.1 mg/mL of streptomycin at 37 °C in a humidified incubator for 24 h with 5% CO2 and 1% O2 to prevent damage by O2. The fibroblast monolayer was artificially injured by scratching across the surface of the plate with a pipette tip. After washing to remove detached cells and debris, the wells were further cultured in medium with or without COP or the VC derivative at 37 °C in a humidified incubator with 5% CO2 and 20% O2. Following incubation, the fibroblasts were incubated with a calcein-AM fluorescent probe (1 μg/mL, Dojindo) for 30 min. The degree of migration of the fibroblasts was determined relative to the average gap at 12 h after the creation of the scratch wounds using fluorescent microscopy.
Statistics
The statistical analyses were performed using Student’s t-test for comparisons between two groups and Tukey’s test for comparisons between three groups. Differences in the data were considered to be significant for p values less than 0.05. All data are expressed as the mean ± SD.
Results
CP and the VC derivative additively increased the skin thickness of the hairless Sod1−/− mice
In the present study, in order to elucidate the beneficial effects of CP and VC on skin thinning, we performed co-treatment with oral CP and the transdermal VC derivative in the hairless Sod1−/− mice for eight weeks. As shown in Fig. , the co-treatment significantly increased the epidermal and dermal thickness in the Sod1−/− mice compared to that observed in the hairless Sod1−/− mice administered the casein peptide only. On the other hand, treatment with the casein peptide plus the VC derivative, the casein peptide alone or CP alone failed to change the skin thickness of the hairless Sod1−/− mice (Fig. (A)–(C)). In order to exclude the adverse effects of the administration of CP and the VC derivative on the skin tissues, we next investigated histological changes in the epidermis and dermis in wild-type mice (Fig. (D) and (E)). The co-administration of these compounds showed no effects on the skin thickness of the hairless wild-type mice (Fig. (D) and (E)). Similarly, the other three treatments also showed no adverse effects on skin morphology in the hairless wild-type mice (Fig. (D) and (E)). These findings demonstrate that combination treatment with CP and the VC derivative significantly improved skin atrophy in the hairless Sod1−/− mice.
Fig. 1. Co-treatment with CP and the VC derivative additively thickened the epidermal and dermal layers in the hairless Sod1−/− mice.
Notes: (A) Hematoxylin and eosin staining of the back skin of hairless Sod1−/− and Sod1+/+ mice treated with the casein peptide (casein), CP (collagen), casein peptide plus the VC derivative (VC) and CP plus the VC derivative. Casein peptide or CP was orally administered, while the VC derivative was transdermally applied on the skin once a day for eight weeks. E, epidermis; D, dermis. The scale bar represents 50 μm (top) and 200 μm (bottom). The thickness of the epidermis (B) and dermis (C) of the back skin of the hairless Sod1−/− mice. The thickness of the epidermis (D) and dermis (E) of the back skin of the hairless Sod1+/+ mice. The data indicate the mean ± SD; *p < 0.05.
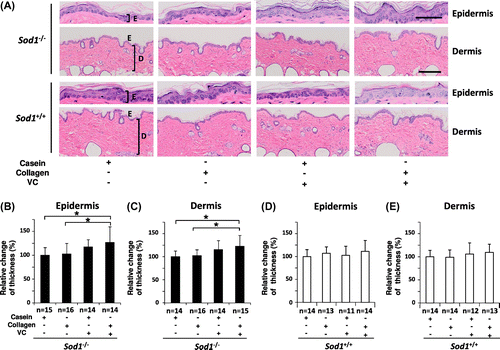
CP and the VC derivative significantly decreased oxidative damage in the skin of the hairless Sod1−/− mice
We previously demonstrated that Sod1 loss induces oxidative damage in several organs, including the skin and bones.Citation17,23) In order to evaluate oxidative damage in the skin, we measured the lipid peroxidation levels in the skin tissues (Fig. ). Regarding the MDA content, co-treatment with the above compounds significantly reduced the MDA levels in the skin tissues of the mutant mice (Fig. (A)). In contrast, the other three treatments, including the administration of casein peptide only, CP only and casein peptide plus the VC derivative, did not decrease the MDA levels in the skin tissues (Fig. (A)). Regarding the 8-isoprostane levels, co-treatment with CP and the VC derivative also significantly decreased the 8-isoprostane content in the skin extracts (Fig. (B)). Interestingly, treatment with CP only and casein peptide plus the VC derivative, but not casein peptide only, significantly downregulated the 8-isoprostane levels in the skin lysates (Fig. (B)). These data indicate that the combined treatment mitigated oxidative damage in the skin of the hairless Sod1−/− mice.
Fig. 2. Treatment with CP and the VC derivative significantly decreased lipid peroxidation in the hairless Sod1−/− skin.
Notes: The (A) MDA and (B) 8-isoprostane content in skin lysates obtained from hairless Sod1−/− male mice treated with the casein peptide only, CP only, casein peptide plus the VC derivative and CP plus the VC derivative. The data indicate the mean ± SD; *p < 0.05, **p < 0.01.
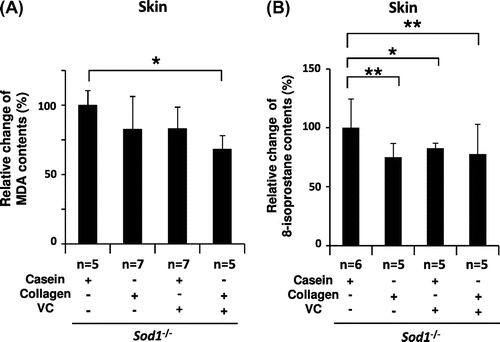
CP and the VC derivative partially normalized the transcriptional profiles of skin-related genes in the hairless Sod1−/− skin
In order to investigate the molecular mechanisms underlying the rescue effects of co-treatment with CP plus the VC derivative on skin thinning in hairless Sod1−/− mice, we analyzed the expression patterns of extracellular matrix-related genes in the skin. In the hairless Sod1−/− mice, the skin mRNA levels of the type I collagen gene (Col1a1) were significantly downregulated, while those of the matrix metalloproteinase 2 gene (Mmp2) were significantly upregulated compared to that observed in the hairless Sod1+/+ mice, indicating reduced collagen biosynthesis (Fig. (A) and (B)). Moreover, the mRNA levels of the hyaluronan synthase 2 gene (Has2) were also significantly downregulated in the mutant skin (Fig. (C)). In contrast, the mRNA levels of Ki67, a marker of the proliferation of skin cells, showed no alterations in their expression between the hairless Sod1+/+ and Sod1−/− mice (Fig. (D)). These results suggest that Sod1 deficiency causes skin thinning due to dysregulation of the extracellular matrix. Among the altered genes, the combined treatment significantly corrected the mRNA levels of Col1a1 and Has2, but not Mmp2, (Fig. (A)–(C)), suggesting that the co-treatment increased components of the extracellular matrix, such as collagen and hyaluronic acid, thereby normalizing the skin thickness.
Fig. 3. Treatment with CP and the VC Derivative partially corrected the transcriptional profiles of skin-related genes in the skin tissues of the hairless Sod1−/− mice.
Notes: The relative mRNA expression levels of (A) Col1a1, (B) Mmp2, (C) Has2 and (D) Ki67 were determined using qRT-PCR. The data indicate the mean ± SD; *p < 0.05.
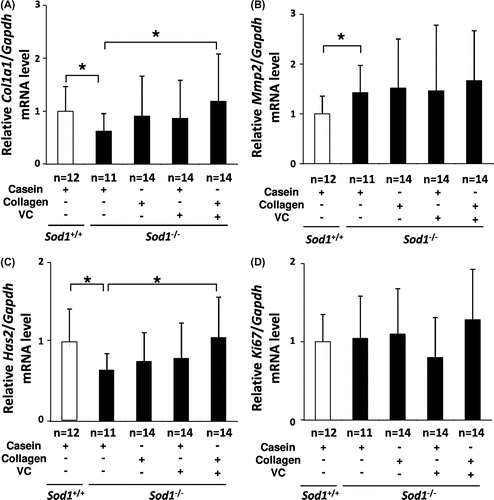
Proton-coupled oligopeptide transporter (POT) regulates the transport of oligopeptides into cells.Citation24) Since ingested CP is digested and absorbed partly as an oligopeptide, we next analyzed the expression profiles of POT genes, including Pept1, Pept2, Pht1, and Ci1 (PHT2 in humans), in the skin tissues. As shown in Fig. , the mRNA levels of Pept1, Pept2, and Pht1 were constitutively expressed in the skin in both the hairless Sod1+/+ and Sod1−/− mice. Furthermore, these three genes were not altered by any of the treatments (Fig. (A)–(C)). In contrast, the Ci1 gene expression was significantly upregulated in the skin of the hairless Sod1−/− mice compared to that observed in the hairless Sod1+/+ mice (Fig. (D)). Interestingly, co-administration selectively normalized the Ci1 expression in the hairless Sod1−/− skin (Fig. (D)).
Fig. 4. Treatment with CP and the VC derivative partially normalized the transcriptional profiles of POT family genes in the skin tissues of the hairless Sod1−/− mice.
Notes: The relative mRNA expression levels of (A) Pept1, (B) Pept2, (C) Pht1, and (D) Ci1 were determined using qRT-PCR. The data indicate the mean ± SD; *p < 0.05.
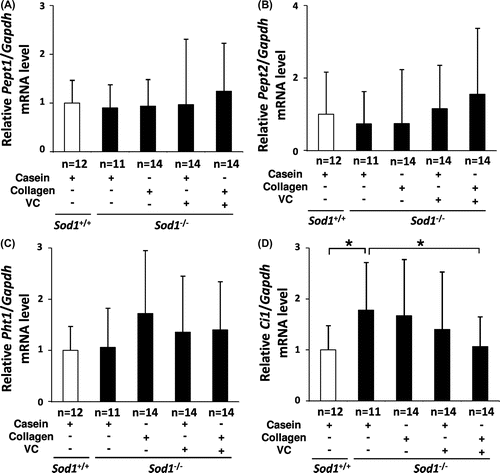
COP mixture and the VC derivative cooperatively attenuated the impairment of cellular phenotypes in the Sod1−/− dermal fibroblasts
We determined plasma levels of the eight types of COP in human blood at 1 hr after ingestion of 25 g CP (unpublished results). Based on these data, the eight types of COP were mixed to prepare a COP mixture at the concentration described in Materials and Methods. Shigemura et al. reported that Pro-Hyp and Hyp-Gly promote cellular proliferation in dermal fibroblasts.Citation10,11) On the other hand, we previously reported that treatment with VC and/or its derivatives significantly promotes the proliferation of Sod1−/− fibroblasts.Citation17,25) In this context, we investigated whether the COP mixture interacts with the effects of the VC derivative in promoting the proliferation of Sod1−/− fibroblasts in vitro. The organ culture experiments using skin disks revealed that the Sod1−/− fibroblasts demonstrated marked suppression of their outgrowth capacity compared to that observed in the Sod1+/+ mice, indicating impairment of the migration and proliferation of Sod1−/− fibroblasts (Fig. (A) and (B)). In order to analyze the combined effects of the COP mixture and the VC derivative in vitro, we added the COP mixture and/or the VC derivative into the organ culture of Sod1−/− skin disks (Fig. (C)). Treatment with the VC derivative only, not the COP mixture only, significantly promoted fibroblast outgrowth from the skin disks. Interestingly, the combined treatment cooperatively enhanced the outgrowth activity (Fig. (C)). In the scratch wound assay, co-treatment with the COP mixture and the VC derivative also significantly facilitated the healing activity of the Sod1−/− fibroblasts (Fig. (D)). These findings collectively suggest that the COP mixture promoted the bioactivity of the VC derivative with respect to the migration and proliferation of Sod1−/− fibroblasts in vitro.
Fig. 5. COP mixture and the VC derivative cooperatively attenuated the impairment of the migration and proliferation of Sod1−/− fibroblasts in vitro.
Notes: (A and B) Number of outgrowth fibroblasts of Sod1+/+ and Sod1−/− mice in the organ culture. (C) Treatment with the COP mixture plus the VC derivative cooperatively promoted the outgrowth activity of the skin fibroblasts. (D) The scratch wound assay allowed for the direct evaluation of migration in vitro. (D) Fibroblasts of Sod1−/− mice were scratched with a pipet tip and cultured for 12 h. (D) Following incubation, the hearing area was quantified by detecting calcein-labeled fibroblasts in the scratch area. 1x and 2x COP indicate one- or twofold concentrations of the COP mixture, respectively. The data indicate the mean ± SD; *p < 0.05, **p < 0.01, ***p < 0.001. The scale bars represent 100 μm.
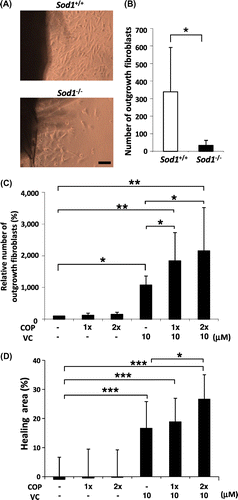
Discussion
In the present study, we demonstrated that co-treatment with CP and the VC derivative prevented skin thinning via upregulation of the Col1a1 and Has2 expression in Sod1−/− skin (Figs. and ). Furthermore, we confirmed that treatment with either CP or VC only failed to attenuate the skin pathology of the hairless Sod1−/− mice (Fig. ). On the other hand, several reports have shown that treatment with CP ingestion only significantly improves epidermal thickening and impairment of skin elasticity in UVB-irradiated hairless mice.Citation26–28) These data indicate that treatment with CP only discriminates rescue effects between hairless Sod1−/− and UVB-irradiated hairless wild-type mice. In fact, Sod1−/− fibroblasts exhibit marked loss of cell viability and a significant increase in apoptotic cell death associated with a 10-fold increase in intracellular reactive oxygen species (ROS) generation.Citation25) In contrast, UV irradiation results in a 50% reduction of cell viability associated with an only threefold increase in ROS generation.Citation29) These differences in the magnitude of ROS generation and cellular damage may account for the limited effects of treatment with CP only in hairless Sod1−/− mice. VC treatment attenuates excess oxidative stress both in vivo and in vitro; therefore, CP functions as a bioactive peptide in Sod1−/− mice.
Alternatively, Mendis et al. reported that CP functions as an antioxidant in vitro.Citation30) This phenomenon may partly explain why CP ingestion attenuates UV-induced skin pathology. On the other hand, CP-derived COP has the ability to stimulate skin cells. For example, Pro-Hyp and Hyp-Gly stimulate the proliferation of skin fibroblasts in vitro.Citation10,11) It is noteworthy that treatment with CP alone induced a significant decrease in the levels of 8-isoprostane, a marker of lipid peroxidation, in the skin of the hairless Sod1−/− mice (Fig. (B)). This finding suggests that CP ingestion alone improves, at least in part, age-related oxidative damage in the skin in vivo. We also cannot exclude that VC enhanced the absorption or bioavailability of digested CP, thus promoting bioactivity of COP in Sod1−/− mice.
As shown in Fig. , Sod1 loss induced the transcriptional downregulation of the Col1a1 gene. Proinflammatory cytokines, including TNF-α, inhibit the type I collagen expression in skin fibroblasts.Citation31) Garela and colleagues recently reported that the downstream molecule, NF-κB, suppresses COL1A1 gene transcription in human dermal fibroblasts Citation32) and accumulates prior to activation in the nucleus in association with the downregulation of the COL1A1 gene in aged human fibroblasts.Citation33).Since oxidative stress generally activates NF-κB, co-treatment with CP and VC may modulate NF-κB-mediated Col1a1 repression, resulting in improvements in collagen homeostasis in Sod1−/− skin.
Peptide uptake systems are conserved in all organisms, including bacteria, mammals, and plants.Citation34) In these systems, the POT family, including PEPT1, PEPT2, PHT1, and PHT2 (Ci1 in mice), plays an important role as oligopeptide transporters.Citation24) These POT genes can recognize approximately 8400 types of di/tripeptides and play a pivotal role in the transmembrane transport of oligopeptides in mammals.Citation35) PEPT1 expressed in the epithelium of the small intestine plays a functional role in the absorption of nutritional peptides,Citation36) while PEPT2 expressed in the renal tubules is involved in the reabsorption of peptides from primitive urine.Citation37) In addition, PHT1 has been isolated as a neuro-specific peptide/histidine transporter.Citation38) Furthermore, a novel peptide/histidine transporter, PHT2, that is primarily expressed in the lymphatic system has been cloned in rats.Citation39) We herein identified four POT genes constitutively expressed in murine skin tissues (Fig. (A)–(D)). Interestingly, only the Ci1 gene was selectively upregulated in the Sod1−/− skin (Fig. (D)) and normalized by co-treatment with CP and the VC derivative in the Sod1−/− mice (Fig. (D)). These results suggest that intrinsic oxidative stress induces the Ci1 expression and may alter the transport profile of COP uptake in skin cells. Further analyses are needed to clarify the physiological functions of POT genes, particularly Ci1, in collagen biosynthesis and the maintenance of skin thickness.
In conclusion, co-treatment with CP and VC effectively protects against intrinsic oxidative stress-induced skin atrophy. The use of combinatory therapy, such as that involving antioxidants and active substances, provides a novel strategy for treating age-related tissue damage.
Acknowledgments
This research was supported by Nippi, Inc. and Showa Denko K.K. We thank Dr. Eiichi Kitakuni (Showa Denko) for providing the VC derivative. We also thank Daichi Morikawa, Keiji Kobayashi, Masato Koike, Koichi Nakasone, Keiko Sato, Kazue Kinoshita, Ayami Goda and Yuki Nakayama (Chiba University) and Dr. Keisuke Tanaka and Masashi Kusubata (Nippi, Inc.) for their valuable technical assistance.
Notes
Abbreviations: COP, collagen oligopeptide; CP, collagen peptide; Hyp, hydroxyproline; MDA, malondialdehyde; POT, proton-coupled oligopeptide transporter; ROS, reactive oxygen species; VC, vitamin C.
References
- Shuster S, Black MM, McVITIE E. The influence of age and sex on skin thickness, skin collagen and density. Br. J. Dermatol. 1975;93:639–643.10.1111/bjd.1975.93.issue-6
- Moskowitz RW. Role of collagen hydrolysate in bone and joint disease. Semin. Arthritis. Rheum. 2000;30:87–99.10.1053/sarh.2000.9622
- Zague V. A new view concerning the effects of collagen hydrolysate intake on skin properties. Arch. Dermatol. Res. 2008;300:479–483.10.1007/s00403-008-0888-4
- Proksch E, Segger D, Degwert J, Schunck M, Zague V, Oesser S. Oral supplementation of specific collagen peptides has beneficial effects on human skin physiology: a double-blind, placebo-controlled study. Skin Pharmacol. Physiol. 2014;27:47–55.10.1159/000351376
- Nomura Y, Oohashi K, Watanabe M, Kasugai S. Increase in bone mineral density through oral administration of shark gelatin to ovariectomized rats. Nutrition. 2005;21:1120–1126.10.1016/j.nut.2005.03.007
- Liang J, Pei X, Zhang Z, Wang N, Wang J, Li Y. The protective effects of long-term oral administration of marine collagen hydrolysate from chum salmon on collagen matrix homeostasis in the chronological aged skin of Sprague-Dawley male rats. J. Food Sci. 2010;75:H230–H238.10.1111/j.1750-3841.2010.01782.x
- Inoue Y, Itoh H, Aoki M, Ogawa S, Yamane T, Baba T, Tachibana N, Kohno M, Oishi Y, Kobayashi-Hattori K. Accelerating effect of soy peptides containing collagen peptides on type I and III collagen levels in rat skin. Biosci. Biotechnol. Biochem. 2012;76:1549–1551.10.1271/bbb.120088
- Iwai K, Hasegawa T, Taguchi Y, Morimatsu F, Sato K, Nakamura Y, Higashi A, Kido Y, Nakabo Y, Ohtsuki K. Identification of food-derived collagen peptides in human blood after oral ingestion of gelatin hydrolysates. J. Agric. Food. Chem. 2005;53:6531–6536.10.1021/jf050206p
- Ohara H, Matsumoto H, Ito K, Iwai K, Sato K. Comparison of quantity and structures of hydroxyproline-containing peptides in human blood after oral ingestion of gelatin hydrolysates from different sources. J. Agric. Food. Chem. 2007;55:1532–1535.10.1021/jf062834s
- Shigemura Y, Iwai K, Morimatsu F, Iwamoto T, Mori T, Oda C, Taira T, Park EY, Nakamura Y, Sato K. Effect of Prolyl-hydroxyproline (Pro-Hyp), a food-derived collagen peptide in human blood, on growth of fibroblasts from mouse skin. J. Agric. Food. Chem. 2009;57:444–449.10.1021/jf802785h
- Shigemura Y, Akaba S, Kawashima E, Park EY. Identification of a novel food-derived collagen peptide, hydroxyprolyl-glycine, in human peripheral blood by pre-column derivatisation with phenyl isothiocyanate. Food Chem. 2011;129:1019–1024.10.1016/j.foodchem.2011.05.066
- Murad S, Grove D, Lindberg KA, Reynolds G, Sivarajah A, Pinnell SR. Regulation of collagen synthesis by ascorbic acid. Proc. Nat. Acad. Sci. 1981;78:2879–2882.10.1073/pnas.78.5.2879
- Franceschi RT. The developmental control of osteoblast-specific gene expression: role of specific transcription factors and the extracellular matrix environment. Crit. Rev. Oral. Biol. Med. 1999;10:40–57.10.1177/10454411990100010201
- Geesin JC, Darr D, Kaufman R, Murad S, Pinnell SR. Ascorbic acid specifically increases type I and type III procollagen messenger RNA levels in human skin fibroblast. J. Invest. Dermatol. 1988;90:420–424.10.1111/jid.1988.90.issue-4
- Nusgens BV, Humbert P, Rougier A, Colige AC, Haftek M, Lambert CA, Richard A, Creidi P, Lapiere CM. Topically applied vitamin C enhances the mRNA level of collagens I and III, their processing enzymes and tissue inhibitor of matrix metalloproteinase 1 in the human dermis. J. Invest. Dermatol. 2001;116:853–859.10.1046/j.0022-202x.2001.01362.x
- Murakami K, Inagaki J, Saito M, Ikeda Y, Tsuda C, Noda Y, Kawakami S, Shirasawa T, Shimizu T. Skin atrophy in cytoplasmic SOD-deficient mice and its complete recovery using a vitamin C derivative. Biochem. Biophys. Res. Commun. 2009;382:457–461.10.1016/j.bbrc.2009.03.053
- Shibuya S, Kinoshita K, Shimizu T. Protective effects of vitamin C derivatives on skin atrophy caused by Sod1 deficiency. In: Preedy BS, editor. Handbook of diet, nutrition and the skin. Wageningen Academic Publishers: Wageningen, The Netherlands; 2011. p. 351–364.
- Shibuya S, Nojiri H, Morikawa D, Koyama H, Shimizu T. Protective effects of vitamin C on age-related bone and skin phenotypes caused by intracellular reactive oxygen species. In: Preedy BS, editor. Aging: Oxidative stress and dietary antioxidants. New York, NY: Academic Press. 2014. p. 137–144.
- Kageyama K, Yamada R, Otani S, Hasuma T, Yoshimata T, Seto C, Takada Y, Yamaguchi Y, Kogawa H, Miwa N. Abnormal cell morphology and cytotoxic effect are induced by 6-0-palmitol-ascorbate-2-0-phosphate, but not by ascorbic acid or hyperthermia alone. Anticancer Res. 1999;19:4321–4325.
- Morikawa D, Nojiri H, Saita Y, Kobayashi K, Watanabe K, Ozawa Y, Koike M, Asou Y, Takaku T, Kaneko K, Shimizu T. Cytoplasmic reactive oxygen species and SOD1 regulate bone mass during mechanical unloading. J. Bone Miner. Res. 2013;28:2368–2380.10.1002/jbmr.1981
- Uchiyama S, Shimizu T, Shirasawa T. CuZn-SOD deficiency causes ApoB degradation and induces hepatic lipid accumulation by impaired lipoprotein secretion in mice. J. Biol. Chem. 2006;281:31713–31719.10.1074/jbc.M603422200
- Nojiri H, Shimizu T, Funakoshi M, Yamaguchi O, Zhou H, Kawakami S, Ohta Y, Sami M, Tachibana T, Ishikawa H, Kurosawa H, Kahn RC, Otsu K, Shirasawa T. Oxidative stress causes heart failure with impaired mitochondrial respiration. J. Biol. Chem. 2006;281:33789–33801.10.1074/jbc.M602118200
- Nojiri H, Saita Y, Morikawa D, Kobayashi K, Tsuda C, Miyazaki T, Saito M, Marumo K, Yonezawa I, Kaneko K, Shirasawa T, Shimizu T. Cytoplasmic superoxide causes bone fragility owing to low-turnover osteoporosis and impaired collagen cross-linking. J. Bone Miner. Res. 2011;26:2682–2694.10.1002/jbmr.489
- Steiner HY, Naider F, Becker JM. The PTR family: a new group of peptide transporters. Mol. Microbiol. 1995;16:825–834.10.1111/mmi.1995.16.issue-5
- Watanabe K, Shibuya S, Koyama H, Ozawa Y, Toda T, Yokote K, Shimizu T. Sod1 Loss Induces Intrinsic Superoxide Accumulation Leading to p53-Mediated Growth Arrest and Apoptosis. Int. J. Mol. Sci. 2013;14:10998–11010.10.3390/ijms140610998
- Zhuang Y, Hou H, Zhao X, Zhang Z, Li B. Effects of collagen and collagen hydrolysate from jellyfish (Rhopilema esculentum) on mice skin photoaging induced by UV irradiation. J. Food Sci. 2009;74:H183–H188.10.1111/jfds.2009.74.issue-6
- Tanaka M, Koyama Y, Nomura Y. Effects of collagen peptide ingestion on UV-B-induced skin damage. Biosci. Biotechnol. Biochem. 2009;73:930–932.10.1271/bbb.80649
- Oba C, Ohara H, Morifuji M, Ito K, Ichikawa S, Kawahata K, Koga J. Collagen hydrolysate intake improves the loss of epidermal barrier function and skin elasticity induced by UVB irradiation in hairless mice. Photoderm. Photoim. Photom. 2013;29:204–211.10.1111/phpp.2013.29.issue-4
- Iovine B, Nino M, Irace C, Bevilacqua MA, Monfrecola G. Ultraviolet B and A irradiation induces fibromodulin expression in human fibroblasts in vitro. Biochimie. 2009;91:364–372.10.1016/j.biochi.2008.10.017
- Mendis E, Rajapakse N, Kim SK. Antioxidant properties of a radical-scavenging peptide purified from enzymatically prepared fish skin gelatin hydrolysate. J. Agric. Food. Chem. 2005;53:581–587.10.1021/jf048877v
- Verrecchia F, Mauviel A. TGF-beta and TNF-alpha: antagonistic cytokines controlling type I collagen gene expression. Cell. Signalling. 2004;16:873–880.10.1016/j.cellsig.2004.02.007
- Beauchef G, Bigot N, Kypriotou M, Renard E, Poree B, Widom R, Dompmartin-Blanchere A, Oddos T, Maquart FX, Demoor M, Boumediene K, Galera P. The p65 subunit of NF-kappaB inhibits COL1A1 gene transcription in human dermal and scleroderma fibroblasts through its recruitment on promoter by protein interaction with transcriptional activators (c-Krox, Sp1, and Sp3). J. Biol. Chem. 2012;287:3462–3478.10.1074/jbc.M111.286443
- Bigot N, Beauchef G, Hervieu M, Oddos T, Demoor M, Boumediene K, Galéra P. NF-kappaB accumulation associated with COL1A1 transactivators defects during chronological aging represses type I collagen expression through a -112/-61-bp region of the COL1A1 promoter in human skin fibroblasts. J. Invest. Dermatol. 2012;132:2360–2367.10.1038/jid.2012.164
- Daniel H, Spanier B, Kottra G, Weitz D. From bacteria to man: archaic proton-dependent peptide transporters at work. Physiology. 2006;21:93–102.10.1152/physiol.00054.2005
- Solcan N, Kwok J, Fowler PW, Cameron AD, Drew D, Iwata S, Newstead S. Alternating access mechanism in the POT family of oligopeptide transporters. EMBO J. 2012;31:3411–3421.10.1038/emboj.2012.157
- Fei YJ, Kanai Y, Nussberger S, Ganapathy V, Leibach FH, Romero MF, Singh SK, Boron WF, Hediger MA. Expression cloning of a mammalian proton-coupled oligopeptide transporter. Nature. 1994;368:563–566.10.1038/368563a0
- Liu W, Liang R, Ramamoorthy S, Fei YJ, Ganapathy ME, Hediger MA, Ganapathy V, Leibach FH. Molecular cloning of PEPT2, a new member of the H+/peptide cotransporter family, from human kidney. Biochim. Biophys. Acta. 1995;1235:461–466.10.1016/0005-2736(95)80036-F
- Yamashita T, Shimada S, Guo W, Sato K, Kohmura E, Hayakawa T, Takagi T, Tohyama M. Cloning and functional expression of a brain peptide/histidine transporter. J. Biol. Chem. 1997;272:10205–10211.
- Sakata K, Yamashita T, Maeda M, Moriyama Y, Shimada S, Tohyama M. Cloning of a lymphatic peptide/histidine transporter. Biochem. J. 2001;356:53–60.10.1042/0264-6021:3560053