Abstract
Certain Japonica rice plant varieties (Oryza sativa L.) show resistance to the eggs of the white backed planthopper (Sogatella furcifera Horváth) by the formation of watery lesions and production of benzyl benzoate, an active ovicidal compound. Benzyl benzoate results in high ovicidal activity of S. furcifera eggs and reduces egg hatchability. The production of benzyl benzoate by the rice plants is only induced by an unknown elicitor(s) from the female S. furcifera. Therefore, we tried to isolate and identify these elicitors. An active 80% MeOH extract of S. furcifera was separated on a reverse-phase ODS MPLC, and the elicitor(s) was eluted in 100% MeOH and 100% EtOH fractions. Further separation of the active 100% MeOH fraction using a HPLC led to the isolation of four active compounds. The structures of each compound were determined by using NMR, LC-MS, and GC-MS spectra. The compounds were 1,2-dilinoleoyl-sn-glycero-3-phosphocholine, 1,2-dipalmitoyl-sn-glycero-3-phosphoethanolamine, 1-palmitoyl-2-oleoyl-X-glycero-3-phosphoethanolamine, and 1,2-dioleoyl-sn-glycero-3-phosphoethanolamine.
Graphical Abstract
The structures were determined by using NMR, LC-MS, and GC-MS spectra. The major compound was 1,2-dilinoleoyl-sn-glycero-3-phosphocholine. So, we show a structure of a compound.
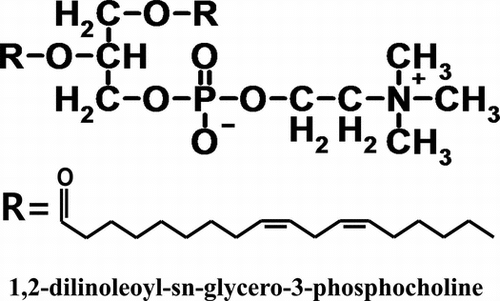
The white backed planthopper, Sogatella furcifera Horváth, migrates to Japan from the Chinese mainland every year during the early part of the rice-growing season.Citation1,2) The total immigrant density has increased since the mid 1970s.Citation3) The growing population of S. furcifera has caused a variety of damage to the rice plants, such as brown ear of the rice plants, black-cracked rice kernels,Citation4) and rusty rice kernels.Citation5) Recently, S. furcifera was classified as a serious pest of the rice plant in Japan.Citation6)
As described above, rice plants can suffer serious damage due to the planthopper; however, it was reported that some Japonica rice plant varieties are resistant to S. furcifera.Citation7) This resistance is characterized by the formation of watery lesions and production of the ovicidal active compound benzyl benzoate near the ovipositional part of the rice plant.Citation8) The benzyl benzoate results in a high ovicidal activity of S. furcifera eggs and reduces egg hatchability. Although the mechanism that produces this ovicidal compound in response to the ovipositional behavior of S. furcifera is unknown,Citation9,10) Yang et al.Citation11) recently determined that benzyl benzoate is induced by elicitor(s) present in only female S. furcifera.
Here, we sought to isolate and determine the structure of the elicitor(s) in female S. furcifera.
Materials and methods
Insects
Stock colonies of S. furcifera were successively reared on rice seedlings (cv. Nihonbare) at 25 ± 1 °C with a relative humidity of 60% and 16/8-h (L/D) illumination.
Rice plants
The rice plants (cv. Natsuhikari) used for extraction were cultivated in a greenhouse without insecticidal treatment after the seedlings were individually planted in plastic cups (3 cm i.d.×6 cm).
Extraction and isolation
A total of 5000 mature females of S. furcifera obtained from stock colonies were crushed with a mortar, and immediately extracted using 80% MeOH in water. After 1 d, the MeOH extract (0.6560 g) was solved in H2O and applied on a ODS MPLC with a 15 mm i.d. × 300 mm ODS, 2 mL/min flow rate, and Yamazen 600A pump (Yamazen Corp., Osaka, Japan) to yield water (0.2510 g), 20% MeOH (0.0047 g), 60% MeOH (0.0121 g), 80% MeOH (0.0181 g), 100% MeOH (0.2580 g), and 100% EtOH (0.0232 g) fractions (Fig. ). The 100% MeOH fr. (0.2580 g) was further fractionated using reverse-phase HPLC, (Shimadzu, Kyoto, Japan) (Fig. Citation1)) consisting of a 21.5 mm i.d.×300 mm ODS column (GL Science, Tokyo, Japan), a UV detector set at 210 nm, and 100% MeOH as a solvent with a flow rate of 5 mL/min. Frs. A (Rt = 0–25 min), B (Rt = 25–49 min), C (Rt = 49–80 min), D (Rt = 80–99 min), and E (Rt = 99–125 min) were obtained. In addition to these, further fractionation of C fr. using HPLC (Fig. Citation2)) yielded C-1 (Rt = 49–52 min), C-2 (Rt = 52–70 min), and C-3 (Rt = 70–80 min) frs. The C-2 fr. (0.0192 g) was then fractionated by a reverse-phase HPLC (Shimadzu, Kyoto, Japan) (Fig. Citation3)) consisting of a 4.6 mm i.d. × 250 mm column (Capcellpak MG-II, Shiseido, Tokyo, Japan), a UV detector set at 210 nm, and 100% MeOH as a solvent with a flow rate of 1 mL/min. This yielded fractions of C-2-1 (Rt = 0–5.5 min), C-2-2 (Rt = 5.5–10 min), C-2-3 (Rt = 10–13 min), and C-2-4 (Rt = 13–20 min). From the C-2-3 fr., compound A (10.2 mg) was obtained. The yield was 40.8 μg/20 females. Similarly, Compound B (7.0 mg) was obtained from C-2-4. Compound B was a mixture that included B-1 (3.32 μg/20 females), B-2 (7.96 μg/20 females), and B-3 (5.12 μg/20 females).
Instruments
LC-MS (LCMS 2010, Shimadzu, Kyoto, Japan) was performed in the full-scan mode with a range of m/z 650–950. Nitrogen was used as the drying gas at a flow rate of 1.5 L/min, and the CDL temperature was 250 °C. After methanolysis, the compounds were analyzed using a GC (Agilent Technologies 6890 N, Santa Clara, USA) coupled to a MS (JEOL JMS-600, Tokyo, Japan). The column was a HP-5MS phase (0.32 mm i.d. × 30 m, 0.25 m film thickness, J&W Scientific, Agilent Technologies) programmed for 70 °C for 2 min, 70 to 230 at 10 °C/min, and then held at 230 °C for 5 min. Helium gas was used as the carrier at a flow of 1.3 mL/min. The ionization method used was electron ionization (positive) with an ionization energy at 70 eV. The 1H-, 13C-, H-H COSY, DEPT-135, HMQC, and HMBC NMR spectra were measured using an ECP-500 instrument (JEOL). The chemical shifts are reported in δ ppm using tetramethylsilane (TMS) as an internal standard. Coupling constants (J) are given in Hz.
Bioassays
The stems of rice plants at the 10 leave stage were punctured 50 times using needles. A total of 0.2 mL of H2O that included the MeOH extract fractions from S. furcifera (equivalent to 20 females) was injected into the punctured areas of the plants using a syringe. The stems of additional rice plants were injected with 0.2 mL of H2O only to serve as a negative control. The stems (2 cm length) of additional rice plants were injected with the MeOH extract of S. furcifera (equivalent to 20 females) to serve as a positive control. The amount of benzyl benzoate produced was measured using HPLC.Citation11)
Statistical analysis
The results are expressed as the mean ± SE. Statistically significant differences were assessed using Student’s t-test. P-values < 0.05 and < 0.01 were considered significant, whereas values greater than 0.05 were considered non-significant (ns).
Methanolysis
The samples (1.0 mg) were dissolved in hexane (1.0 mL) and added to a 1.0 mL of 5% hydrogen chloride MeOH solution (Wako Pure Chemical Industries Ltd., Osaka, Japan) in the ampoule tube. The tube was soaked in a water bath for 3 h at 80 °C. After removing the solvent in the ampoule using an evaporator, the product was extracted by ether. Next, the methyl esterification product was analyzed using GC-MS to confirm its structure.
Compound A (1,2-dilinoleoyl-sn-glycero-3-phosphocholine)
1H-NMR (500 MHz, CDCl3) δ: 0.86 {6H, t, J = 6.8 Hz, Fatty acid moiety-Me (FA-Me)}, 1.31–2.74 (56H, m, FA), 3.31 {9H, s, Choline moiety-Me (Ch-Me) }, 3.73 (2H, m, Ch-CH2-N+), 3.87 {2H, m, Glycerol moiety-3 (G-3)}, 4.19 (1H, dd, J = 7.2, 12.0, G-1a), 4.26 (2H, m, Ch-CH2-O-P), 4.38 (1H, dd, J = 2.8, 12.0, G-1b), 5.16 (1H, m, G-2), 5.31 (8H, m, FA-CH=). 13C-NMR (125 MHz, CDCl3): 14.1 (2C, q, FA-Me), 22.6–34.3 (28C, t, FA-CH2), 54.4 (3C, q, Ch-Me), 59.3 (t, d, J = 5.0, Ch-CH2-O-P), 62.9 (t, G-1), 63.3 (t, d, J = 5.7, G-3), 66.3 (t, d, J = 7.3, Ch-CH2-N+), 70.4 (d, d, J = 7.4,G-2), 127.7 (d, FA), 127.9 (d, FA), 129.8 (d, FA), 130.1 (d, FA), 173.0 (s, FA-COO-), 173.3 (s, FA-COO-). LC-MS (ESI-positive) m/z: 783 [M+H]+, 805 [M+Na]+.
Compound B (mixture of 1,2-dipalmitoyl-sn-glycero-3-phosphoethanolamine, 1-palmitoyl-2-oleoyl-X-glycero-3-phosphoethanolamine and 1,2-dioleoyl-sn-glycero-3- phosphoethanolamine)
1H-NMR (500 MHz, CDCl3) δ: 0.82 (6H, m, FA-Me), 1.21–2.69 (m, FA), 3.11 {2H, m, Ethanolamine moiety-CH2-NH2 (EA-CH2-NH2)}, 3.89 (2H, m, G-3), 4.03 (2H, m, EA-CH2-O-P), 4.07 (1H, m, G-1a), 4.31 {1H, dd, J = 3.2, 12.0, G-1b), 5.14 (1H, m, G-2), 5.27 (m, FA-CH=). 13C-NMR (125 MHz, CDCl3): 14.1 (C, q, FA-Me), 14.2 (C, q, FA-Me), 22.6–34.2 (t, FA-CH2), 40.5 (t, d, J = 3.3, EA-CH2-NH2), 62.0 (t, G-1) 62.2 (t, d, J = 4.9, EA-CH2-O-P), 63.9 (t, d, J = 2.4, G-3), 70.1 (d, d, J = 3.2, G-2), 127.8 (d, FA-CH=), 128.0 (d, FA-CH=), 129.9 (d, FA-CH=), 130.1 (d, FA-CH=), 172.9 (s, FA-COO-), 173.3 (s, FA-COO-). LC-MS (ESI-positive) m/z: Compound B-1 (1,2-dipalmitoyl-sn-glycero-3-phosphoethanolamine); 691 [M+H]+, 713 [M+Na]+, Compound B-2 (1-palmitoyl-2-oleoyl-X-glycero-3-phospholethanolamine); 717 [M+H]+, 739 [M+Na]+ and Compound B-3 (1,2-dioleoyl-sn-glycero-3-phosphoethanolamine); 743 [M+H]+, 765 [M+Na]+.
Methyl linoleate, Rt = 11.13 min in GC-MS, m/z (%): 294 (M+, 88.5) 263 (32.7), 220 (9.8), 109 (39.3), 95 (73.8), 67 (100.0).
Methyl palmitate, Rt = 13.40 min in GC-MS, m/z (%): 270 (M+, 4.8), 239 (4.4), 227 (11.0), 143 (14.5), 87 (81.6), 74 (100.0).
Methyl oleate, Rt = 15.10 min in GC-MS, m/z (%): 296 (M+, 2.6), 264 (28.1), 222 (18.4), 180 (14.5), 97 (89.0), 55 (100.0).
Results and discussion
Isolation of the elicitor(s) in S. furcifera
The stems (2 cm length) of rice plants at the 10 leave stage were punctured 50 times using a needle. Next, 0.2 mL of water (negative control) or 0.2 mL of H2O that contains the MeOH extract of S. furcifera (equivalent to 20 females) was injected into the puncture holes using a syringe. The water did not induce the production of benzyl benzoate {1.87 μg/g fresh rice plant equivalent (frp)}, but the MeOH extract significantly induced production of the compound (6.12 μg/g frp).
The active MeOH extract was separated by reverse phase ODS MPLC to obtain H2O, 20% MeOH/H2O, 60% MeOH/H2O, 80% MeOH/H2O, 100% MeOH, and 100% EtOH fractions. Of these 6 frs., the 100% MeOH (43.95 ± 12.72 μg/g frp) and 100% EtOH (31.04 ± 7.08 μg/g frp) frs. clearly induced the production of benzyl benzoate (Fig. ). The H2O fr. (8.58 ± 4.08 μg/g frp), 20% MeOH/H2O fr. (8.90 ± 4.39 μg/g frp), 60% MeOH/H2O fr. (16.79 ± 6.70 μg/g frp), and 80% MeOH/H2O fr. (9.78 ± 1.70 μg/g frp) did not induce the production of benzyl benzoate. These results indicate that the elicitor(s) in female S. furcifera does not consist of a single chemical but rather multiple compounds.
Fig. 2. The amount of benzyl benzoate induced by different S. furcifera fractions.
Note: Each value represents the mean ± SE (n = 6). Fraction data were compared with the negative control (**p < 0.01, ns = not significant).
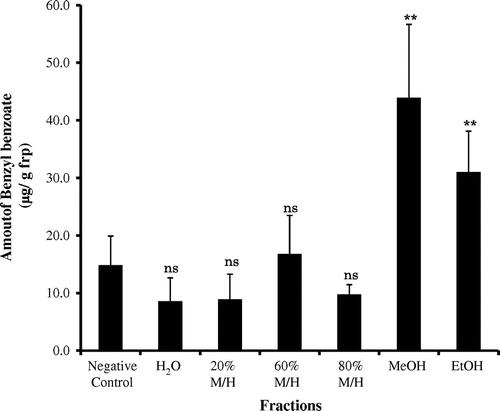
The MeOH fr. was further separated into 5 frs. (A to E) using HPLC. A bioassay clearly indicated that the A fr. (1.58 ± 0.79 μg/g frp), B fr. (5.19 ± 1.97 μg/g frp), and D fr. (6.21 ± 1.70 μg/g frp) showed low levels of induction while the C fr. (22.25 ± 5.78 μg/g frp) and E fr. (14.64 ± 3.83 μg/g frp) showed high levels of activity (Fig. ). Specifically, the C fr. produced the highest induction of benzyl benzoate.
Fig. 3. The amount of benzyl benzoate induced by 100% MeOH fractions.
Note: Each value represents the mean ± SE (n = 6). Fraction data were compared with the negative control (**p < 0.01, *p < 0.05, ns = not significant).
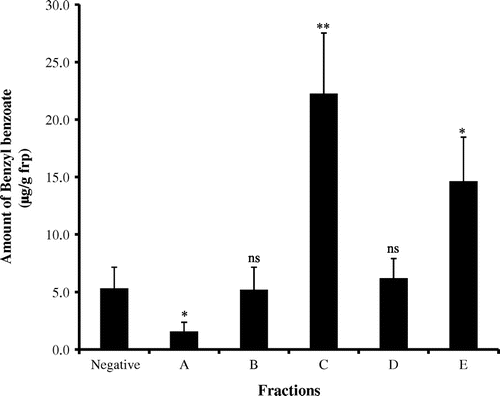
The active C fr. was then separated into 3 frs. identified as C-1 to C-3 {C-1 fr. (2.46 ± 0.68 μg/g frp), C-2 fr. (5.97 ± 1.52 μg/g frp) and C-3 fr. (4.19 ± 1.14 μg/g frp), negative control (0.88 ± 0.41 μg/g frp),} using HPLC (Fig. ). The C-2 fr. was further separated into 4 frs. identified as C-2-1 to C-2-4. The bioassay was completed to yield the following results: C-2-1 fr. (1.34 ± 0.89 μg/g frp), C-2-2 fr. (4.01 ± 2.22 μg/g frp), C-2-3 fr. (5.25 ± 2.12 μg/g frp), C-2-4 fr. (3.06 ± 1.34 μg/g frp), negative control (1.11 ± 0.71 μg/g frp), and positive control (7.07 ± 3.42 μg/g frp) (Fig. ). Based on these results, the C-2-3 fr. was the most active. The C-2-4 fr. also showed high levels of activity. The C-2-3 fr. consisted of a pure compound (compound A) under many HPLC conditions. The C-2-4 fr. (compound B) appeared to be similar to the C-2-3 fr. The yield for compound A was 10.2 mg/5000 females of S. furcifera. The yield for compound B was 7.0 mg/5000 females of S. furcifera.
Structural determination of compound A (1, 2-dilinoleoyl-sn-glycero-3-phosphocholine)
In the 13C-NMR spectrum of compound A, a methyl carbon at δ = 14.1, many methylene carbons at δ = 22.6–34.3, methine carbons based on a double bond at δ = 127.7–130.1, and two carbonyl carbons at δ = 170.0 and 173.3 were observed. Based on this information, compound A has two unsaturated fatty acid moieties, and the moieties exist as an ester. There are also two methylene carbons at δ = 62.9 and 63.3 (d, J = 5.7) and one methine carbon at δ = 70.4 (d, J = 7.4). These three carbons were considered to be constituents of a glycerol moiety. In addition to these suppositions, two coupling methylene carbons (δ = 59.3, d, J = 5.0, δ = 66.3, d, J = 7.3) and three methyl carbons (δ = 54.4) were observed (Fig. ). These carbons were considered to be choline with a phosphoric acid moiety. Based on these observations, compound A is a phospholipid. In the 1H NMR spectrum of compound A, many protons based on two unsaturated fatty acid moieties were observed at δ = 0.86 (6H, t, J = 6.8, FA-Me) 1.31–2.74 (56H, m, FA-CH2), and 5.31 (8H, m, FA-CH=). Five protons of the glycerol moiety at δ = 3.87 (2H, m, G-3), 4.19 (1H, dd, J = 7.2, 12.0, G-1a), 4.38 (1H, dd, J = 2.8, 12.0, G-1b), and 5.16 (1H, m, G-2) and 13 protons of the choline moiety at δ = 3.31 (9H, s, Ch-Me), 3.73 (2H, m, Ch-CH2-N+), and 4.26 (2H, m, Ch-CH2-O-P) were observed. This information confirmed that compound A was phosphatidylcholine. The LC-MS analysis of compound A (ESI-positive) resulted in a m/z at 783 [M+H]+ and 805 [M+Na]+. This result shows that the molecular weight of compound A is 782.
When compound A underwent methanolysis, one methyl ester was obtained that gave an m/z at 294 using GC-MS. The retention time and MS fragment pattern suggests that the methyl ester is methyl linoleoate. These GC-MS data coincide with those obtained from a pure sample (Wako Pure Chemical Industries Ltd., Osaka, Japan). As mentioned above, the structure of compound A was determined to be 1,2-dilinoleoyl-sn-glycero-3-phosphocholine.
Structural elucidation of compound B (1,2-dipalmitoyl-sn-glycero-3-phosphoethanolamine, 1-palmitoyl-2-oleoyl-X-glycero-3-phosphoethanolamine, and 1,2-dioleoyl-sn-glycero-3-phosphoethanolamine)
1H-NMR and 13C-NMR spectra of compound B gave very similar spectra to those of compound A. Compound B also appeared to be a phospholipid. Closely comparing the spectra of compound A and B revealed the disappearance of the choline moiety (1H: δ = 3.31, 9H, s, Ch-Me, 13C: δ = 54.4, 3C, q, Ch-Me) in the 1H- and 13C-NMR spectra of compound B. In addition, the chemical shift value of the methylene carbon at δ = 40.5 (t, d, J = 3.3, EA-CH2-NH2) was shifted to a very high field when compared with that of compound A (δ = 66.3, t, d, J = 7.3, Ch-CH2-N+). These observations prove that compound B is a phospholipid. More specifically, it is not phosphatidylcholine but rather phosphatidylethanolamine. The LC-MS analysis of compound B (ESI-positive) gave an m/z at 691, 713, 717, 739, 743, and 765. These results indicate that compound B is not a pure compound but a mixture. The mixture consisted of compound B-1 (691 [M+H]+, 713 [M+Na]+), compound B-2 (717 [M+H]+, 739 [M+Na]+), and compound B-3 (743 [M+H]+, 765 [M+Na]+).
When compound B underwent methanolysis, two methyl esters were obtained with an m/z at 270 and 296 using GC-MS. The retention times and MS fragment patterns completely coincided with those obtained from pure samples (Wako Pure Chemical Industries Ltd., Osaka, Japan). The fatty acid moieties of the three compounds were determined to be palmitoyl and oleoyl moieties. Based on these LC-MS data, compounds B-1, B-2, and B-3 were 1,2-dipalmitoyl-sn-glycero-3-phosphoethanolamine, 1-palmitoyl-2-oleoyl-X-glycero-3-phosphoethanolamine, and 1,2-dioleoyl-sn-glycero-3-phosphoethanolamine, respectively. The yields of single ions of these three compounds on a LC-MS spectrum were 3.32 μg/20 females for B-1, 7.96 μg/20 females for B-2, and 5.12 μg/20 females for B-3.
We attempted to quantify the activity of compound A in our bioassay using the standard compound 1,2-dilinoleoyl-sn-glycero-3-phosphocholine. This standard compound showed approximately the same activity level as the positive control (Fig. ).
Fig. 7. The amount of benzyl benzoate induced by the 1,2-dilinoleoyl-sn-glycero-3-phosphatidylcholine Standard.
Note: Each value represents the mean ± SE (n = 9). The data were compared with the negative control (**p < 0.01).
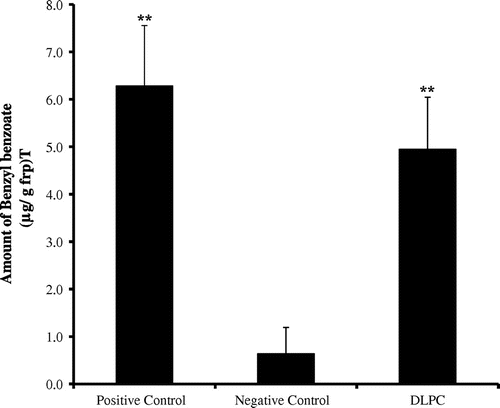
Here, we report for the first time the chemical structures of elicitors from S. furcifera. We have determined that 1,2-dilinoleoyl phosphocholine and a mixture of 1,2-dipalmitoyl-sn-glycero-3-phosphoethanolamine, 1-palmitoyl-2-oleoyl-X-glycero-3-phosphoethanolamine, and 1,2-dioleoyl-sn-glycero-3-phosphoethanolamine, trigger Japonica rice plants to produce an ovicidal compound against S. furcifera eggs. Although we could not confirm which compound(s) of the mixture specifically elicits the activity, our results suggest that it is due to phospholipids, regardless if it is phosphatidylcholine or phosphatidylethanolamine.
Phospholipids are generally found within cell membranesCitation12,13) and distributed on the surfaces of eggs and the ovipositors of insects. Female S. furcifera could secrete and/or insert these phospholipids to the inside of plant tissues with their ovipositional behavior. A review by Hilker and MeinersCitation10) summarized and discussed the elicitors of oviposition-induced plant responses. In this review, the authors suggest that the Japonica rice plant reaction is induced by a set of ovipositional behaviors of S. furcifera as a direct defense. There has been only one report on the structure determination of the specific elicitors that can induce this defensive plant response to egg deposition.
It is well known that phospholipids play a very important role as a chemical transmitter in organisms.Citation13) Salicylic acid (SA) and jasmonic acid (JA) are well-known chemical transmitters in plants. Chemical cascades involving SA are responsible for many induced reactions against pathogens.Citation14,15) Cascades that use JA are involved in many induced responses against wounds and insect attacks.Citation16) Other signaling molecules, such as abscisic acid and ethylene, are also reported to be important chemical transmitters.Citation17,18) These data highlight the importance for the elucidation of the mechanisms behind eliciting the production of the ovicidal compound by Japonica rice plants. We suggest that the phospholipid 1,2-dilinoleoyl phosphocholine and a mixture of 1,2-dipalmitoyl-sn-glycero-3-phosphoethanolamine, 1-palmitoyl-2-oleoyl-X-glycero-3-phosphoethanolamine, and 1,2-dioleoyl-sn-glycero-3-phosphoethanolamine work in a similar way as SA and JA. Thus, the phospholipids guide the ovicidal compound to kill the S. furcifera eggs.
However, the phospholipids from female S. furcifera that we identified as elicitors are very common among phospholipids. Therefore, it is extremely surprising that these common compounds have the ability to elicit the production of benzyl benzoate only when the S. furcifera lays her eggs into the Japonica rice plant varieties. It seems unlikely that only S. furcifera has these common phospholipids among the many rice planthoppers and leafhoppers, such as Nilaparvata lugens and Laodelphax striatellus.Citation9) The distribution and amount of these phospholipids in the female S. furcifera, including those of N. lugens and L. striatellus, should be determined in future studies. In addition, the compounds in other active fractions should also be elucidated.
Supplemental material
The supplemental material for this paper is available at http://dx.doi.org/10.1080/09168451.2014.917266.
Supplemental Fig. 1 caption
Download MS Word (21 KB)Supplemental Fig. 1
Download MS Power Point (110.5 KB)References
- Kisimoto R. Synoptic weather conditions indicating long-distance immigration of planthoppers, Sogatella furcifera Horvath and Nilaparvata lugens Stal. Ecol. Entomol. 1976;1:95–109.10.1111/j.1365-2311.1976.tb01210.x
- Sogawa K, Watanabe T. Outline of long-term yearly fluctuations of the rice planthopper occurrence from light-trap data at Kyushu National agricultural Experiment Station. Proc. Assoc. Plant Prot. Kyushu. 1989;35:65–68. (in Japanese).10.4241/kyubyochu.35.65
- Watanabe T, Sogawa K, Suzuki Y. Analysis of yearly fluctuation in the occurrence of migratory rice planthoppers, Nilaparvata lugens Stål and Sogatella furcifera Horváth, based on light-trap data in northern Kyushu. Jpn. J. Appl. Entomol. Zool. 1994;38:7–15. (in Japanese).10.1303/jjaez.38.7
- Noda H. Damages of ears of rice pant caused by the whitebacked planthopper, Sogatella furcifera (Homoptera: Delphacidae). Appl. Entomol. Zool. 1986;21:474–476.
- Isizaki H, Matsuura H. Occurrence of rusty rice kernels caused by white-backed rice planthopper, Sogatella furcifera Horváth. Proc. Assoc. Plant Prot. Hokuriku 1991;39:51–56. (in Japanese).
- Matsumura M. Characteristics of recent population growth patterns of the white-backed planthopper, Sogatella furcifera Horvath in the Hokuriku district. Proc. Assoc. Plant Prot. Hokuriku. 1991;39:47–50. (in Japanese).
- Sogawa K. Super-susceptibility to the white-backed phanthopper in Japonica-Indica hybrid rice. Kyushu Agric. Res. 1991;53:92. (in Japanese).
- Suzuki Y, Sogawa K, Seino Y. Ovicidal reaction of rice plants against the Whitebacked planthopper, Sogatella furcifera Horvath (Homoptera: Delphacidae). Appl. Entomol. Zool. 1997;32:530–532.
- Seino Y, Suzuki Y, Sogawa K. An ovicidal substance produced by rice plants in response to oviposition by the whitebacked planthopper, Sogatella furcifera (Horvath) (Homoptera: Delphacidae). Appl. Entomol. Zool. 1996;31:467–473.
- Hilker M, Meiners T. Early herbivore alert: insect eggs induce plant defense. J. Chem. Ecol. 2006;32:1379–1397.10.1007/s10886-006-9057-4
- Yang JO, Nakayama N, Toda K, Tebayashi S, Kim CS. Elicitor(s) in Sogatella furcifera (Horváth) causing the Japanese rice plant (Oryza sativa L.) to induce ovicidal substance, benzyl benzoate. Biosci., Biotechnol. Biochem. 2013;77(6):1258–1261.10.1271/bbb.130055
- Wouter HM. Lysophosphatidic Acid, a Multifunctional Phospholipid Messenger. J. Bio. Chem. 1995;270:12949–12952.
- Munnik T, Irvine RF, Musgrave A. Phospholipid signalling in plants. Biochemica et Biophysica Acta. 1998;1389:222–272.10.1016/S0005-2760(97)00158-6
- Malamy J, Carr JP, Klessig DF, Raskin I. Salicylic acid: a likely endogenous signal in the resistance response of tobacco to viral infection. Science. 1990;250:1002–1004.10.1126/science.250.4983.1002
- Metraux JP, Signer H, Ryals J, Ward E, Wyss-Benz M, Gaudin J, Raschdorf K, Schmid E, Blum W, Inverardi B. Increase in salicylic acid at the onset of systemic acquired resistance in cucumber. Science. 1990;250:1004–1006.10.1126/science.250.4983.1004
- Farmer E, Ryan C. Octadecanoid precursors of jasmonic acid activate the synthesis of wound-inducible proteinase inhibitors. Plant Cell. 1992;4:129–134.10.1105/tpc.4.2.129
- Thaler JS, Bostock RM. Interactions between abscisic-acid-mediated responses and plant resistance to pathogens and insects. Ecology. 2004;85:48–58.10.1890/02-0710
- Stout MJ, Thaler JS, Thomma BPH. Plant-mediated interactions between pathogenic microorganisms and herbiborous arthropods. J. Annual Rev. Entomol. 2006;51:663–689.10.1146/annurev.ento.51.110104.151117