Abstract
In the tricarboxylic acid (TCA) cycle, NADP+-specific isocitrate dehydrogenase (NADP+-ICDH) catalyzes oxidative decarboxylation of isocitric acid to form α-ketoglutaric acid with NADP+ as a cofactor. We constructed an NADP+-ICDH gene (icdA)-overexpressing strain (OPI-1) using Aspergillus niger WU-2223L as a host and examined the effects of increase in NADP+-ICDH activity on citric acid production. Under citric acid-producing conditions with glucose as the carbon source, the amounts of citric acid produced and glucose consumed by OPI-1 for the 12-d cultivation period decreased by 18.7 and 10.5%, respectively, compared with those by WU-2223L. These results indicate that the amount of citric acid produced by A. niger can be altered with the NADP+-ICDH activity. Therefore, NADP+-ICDH is an important regulator of citric acid production in the TCA cycle of A. niger. Thus, we propose that the icdA gene is a potentially valuable tool for modulating citric acid production by metabolic engineering.
Graphical Abstract
We revealed that NADP+-specific isocitrate dehydrogenase (NADP+-ICDH) is an important regulator of citric acid production in Aspergillus niger by constructing NADP+-ICDH gene (icdA)-overexpressing strain (OPI-1) using strain WU-2223L as a host.
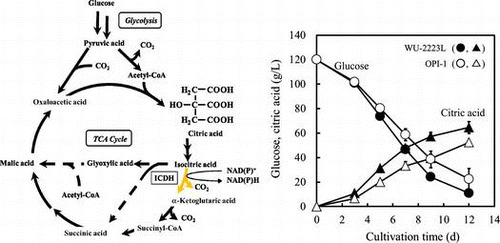
Isocitrate dehydrogenase (ICDH) is an enzyme that catalyzes the oxidative decarboxylation of isocitric acid to form α-ketoglutaric acid via oxalosuccinic acid as an intermediate; NAD+ or NADP+ is used as the cofactor in this process (Fig. ). The reaction catalyzed by ICDH is a regulatory checkpoint in the tricarboxylic acid (TCA) cycle, and α-ketoglutaric acid produced by this reaction contributes to the synthesis of glutamic acid, which is required for synthesizing amino acids.Citation1,2) Therefore, ICDH is an important enzyme in the central metabolism related to the synthesis of organic acids and amino acids.
Fig. 1. Metabolic Pathway in Relation to Citric Acid Production by A. niger.
Note: ICDH, isocitrate dehydrogenase; ICL, isocitrate lyase; TCA Cycle, tricarboxylic acid cycle. Two steps of the glyoxylate cycle—the cleavage of isocitric acid into succinic acid and glyoxylic acid by ICL and the condensation of glyoxylic acid and acetyl-CoA to form malic acid by malate synthase—are shown as dotted arrows.
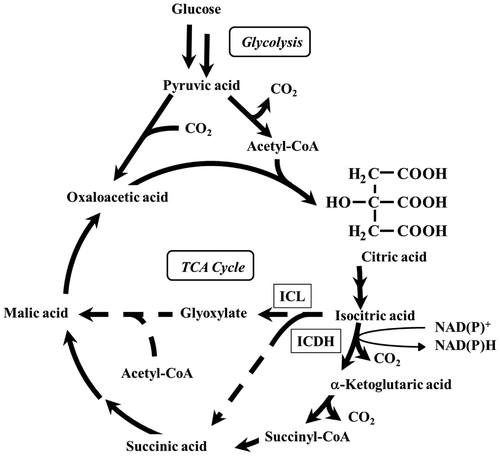
The function and role of ICDH in prokaryotes and eukaryotes are different.Citation3) For example, in Escherichia coli,Citation4,5) only a single enzyme, NADP+-specific ICDH (NADP+-ICDH; EC 1.1.1.42), is responsible for the reaction supplying α-ketoglutaric acid. In eukaryotes, ICDHs vary in their subunit structures, cofactor specificities, and cellular localization.Citation3,6,7) The yeast Saccharomyces cerevisiae has four types of ICDH: NAD+-specific ICDH (NAD+-ICDH; EC 1.1.1.41) and 3 NADP+-ICDHs.Citation8–14) The NAD+-ICDH of S. cerevisiae is a hetero-octamer composed of 2 subunits encoded by idh1 and idh2 and is localized in mitochondria.Citation8–10) In yeast, the 3 NADP+-ICDHs Idp1p, Idp2p, and Idp3p encoded by idp1, idp2, and idp3, respectively,Citation11–14) are differentially directed by targeting signals to specific compartments in the cell. Idp1p contains mitochondrial targeting signal peptides in its N-terminus,Citation11) Idp3p contains peroxisomal targeting signal peptides in its C-terminus,Citation12,13) and Idp2p contains no targeting signal peptides and is localized in the cytoplasm.Citation14) Because intracellular concentrations of citric acid and α-ketoglutaric acid in idp2 disruptants are markedly lower than those in idp1 disruptants,Citation15) mitochondrial NADP+-ICDH (Idp1p) may play a minor role in supplying α-ketoglutaric acid via the TCA cycle compared to mitochondrial NAD+-ICDH. Codisruption of idp2 in idh2 disruptants results in glutamate auxotrophy, indicating that cytosolic NADP+-ICDH (Idp2p) contributes to the synthesis of glutamic acid.Citation2) The expression of peroxisomal NADP+-ICDH gene idp3 is regulated by a carbon source.Citation12,13)
ICDH has also drawn attention in biotechnological applications because it is an essential catalyst in the TCA cycle and is closely linked to the production of industrially important organic acids, such as citric acid. Aspergillus niger is one of the most important filamentous fungi for the industrial production of organic acids and enzymes.Citation16) In particular, A. niger has the advantage of producing high yields of citric acid in the presence of high concentrations of sugar, e.g. glucose and sucrose. The global industrial production of citric acid by fermentation with A. nigerCitation17,18) reached 1.75 million tons/year in 2011.Citation19)
Meixner-Monori et al. partially purified NADP+-ICDH and investigated its enzymatic properties.Citation20) They detected NADP+-ICDH activity in both the mitochondrial and cytoplasmic fractions; however, SDS-PAGE analysis showed only one protein.Citation20) The effects of metal ions and citric acid on the activity of NADP+-ICDH have been reported by Tsekova et al.Citation21) Bowes et al.Citation22) and Agrawal et al.Citation23) Moreover, we previously cloned the gene encoding mitochondrial NADP+-ICDH (icdA) from A. niger WU-2223L, a citric acid-producing A. niger strain, and found mitochondrial and peroxisomal targeting signal peptides in the N- and C-termini, respectively, in the deduced amino acids sequence of the NADP+-ICDH.Citation24) We found that in A. niger two mRNAs of different sizes were transcribed from a single gene, icdA.Citation24)
The relationship between the mechanism underlying citric acid production in A. nigerCitation17,18) and the catalytic function of NADP+-ICDH has been reported. A decrease in the specific activity of the enzyme during the citric acid accumulation phase is generally observed,Citation17,25–27) suggesting the possible involvement of NADP+-ICDH inhibition by citric acid in the massive production of organic acid by A. niger. Furthermore, a model whereby citric acid accumulates through the inhibition of α-ketoglutarate dehydrogenase by increase in α-ketoglutarate concentrations has also been proposed.Citation20)
In this study, we examined whether NADP+-ICDH is one of the regulators of citric acid production in the TCA cycle of A. niger. To investigate the effects of NADP+-ICDH activity on citric acid production by genetic and/or metabolic engineering, we constructed icdA-overexpressing strains derived from A. niger WU-2223L to increase NADP+-ICDH activity.
Materials and methods
A. niger and E. coli strains, cultivation conditions, and media
We used A. niger WU-2223L,Citation17,24,27–32) a hyperproducer of citric acid, as the parental strain, and OPI-1 (described in Results), an icdA-overexpressing strain. The conidia of these strains were suspended in 60 mL of citric acid production medium to a final concentration of 5.0 × 106 conidia/mL and were cultivated aerobically with shaking at 120 rpm in shaker flasks (500 mL) at 30 °C. The citric acid production (SLZ) medium consisted of the following components (per liter of distilled water): glucose, 120 g; (NH4)2SO4, 3 g; K2HPO4, 1 g; KH2PO4, 1 g; MgSO4·7H2O, 0.5 g; MnSO4·5H2O, 0.014 g; FeCl3·6H2O, 0.01 g; and ZnCl2, 0.075 mg. The initial pH was adjusted to 3.0. Unless otherwise indicated, 2% (v/v) methanol was added as a promotive substance for citric acid production.Citation17,24,28–32) For the isolation and maintenance of the transformants of A. niger, Czapek-Dox (CD) medium was used. The CD medium consisted of the following components (per liter of distilled water): glucose, 30 g; NaNO3, 2 g; K2HPO4, 1 g; MgSO4·7H2O, 0.5 g; KCl, 0.5 g; FeSO4·7H2O, 0.01 g; and 0.7 M KCl, added as osmostabilizer for protoplasts. The initial pH was adjusted to 6.0, and when necessary, 10 g/L of agar BA-10 (Ina Food Industry, Nagano, Japan) was added to prepare agar plates of CD medium.
E. coli JM109 cells were used to amplify the plasmids pPANICDH and pPAN8142. The recombinant E. coli cells harboring the plasmids were cultivated in 100-mL Erlenmeyer flasks containing 20 mL of Luria–Bertani (LB) medium with 100 mg/mL of ampicillin at 37 °C for 16 h at 120 rpm.
Preparation of powdered mycelia
After cultivation for appropriate intervals, the mycelia of A. niger were harvested with a glass microfiber filter (GF/A) and then qualitative paper filter No. 4 (Whatman, Brentford, UK). The harvested mycelia were washed twice with pure water on the paper filter, and the resulting mycelia were frozen in liquid nitrogen and ground into a fine powder with a mortar and pestle. The powdered mycelia were stored at −80 °C and used for DNA and RNA isolation.
DNA and RNA isolation
Genomic DNA was isolated from the powdered mycelia using Isoplant II (Nippon Gene, Tokyo) following the manufacturer’s instructions. Total RNA was isolated from the powdered mycelia by using the guanidinium thiocyanate–phenol–chloroform extraction method,Citation33) with some modifications.Citation28–30)
Construction of plasmids
The plasmid pPANICDH for the overexpression of icdA in A. niger WU-2223L was constructed as follows: The cDNA fragment of icdA was amplified by reverse-transcription PCR (RT-PCR) using primer Pr1 (5′-GAC AAG CTT ATG TCG TCT GTC CGG T-3′), which contained a HindIII site, and primer Pr2 (5′-GCA CTA GTT AAA GGC GGG ACT TGA-3′), which contained an SpeI site. RT-PCR was performed using ReverTra-Plus-TM (Toyobo, Osaka, Japan) in accordance with the manufacturer’s instructions. The amplified cDNA fragment and the vector pPAN8142 were digested with HindIII and SpeI and ligated using DNA Ligation Kit Ver. 2.1 (Takara Bio, Shiga, Japan). The vector pPAN8142 was constructed according to the method described by Sakamoto et al.Citation34) The vector pPAN8142 was constructed by ligating a fragment of pNAN8142 digested by PstI and SmaI containing the promoter and terminator regions,Citation35) and a fragment of pPTR ICitation36) digested by PstI and SmaI, containing the pyrithiamine-resistance gene (ptrA).
Transformation of A. niger and overexpression of icdA. The icdA-overexpressing transformants derived from A. niger WU-2223L were constructed using pPANICDH by the protoplast-PEG method, as described previously.Citation37) The transformants were isolated on CD medium containing 100 μg/mL pyrithiamine as the selection marker.
Southern and Northern blot analyses
Southern and Northern blot analyses were performed as described previously.Citation32) An ~500-bp fragment of PCR-amplified icdA from the genomic DNA of A. niger WU-2223L was used as the template and probed with primers Pr3 (5′-ATA CTA GTC GGC GGT ACC GTC TTC CGT-3′) and Pr4 (5′-ATT CTA GAT CTT CAG GGC CAT GAC GAA T-3′) for the Southern blot analysis. For Northern blot analysis, the fragment of icdA amplified by PCR using the Pr1 and Pr2 primers was also labeled by random priming with digoxigenin-dUTP (Roche Diagnostics, Basel, Switzerland) and used as the icdA-specific probe. The cDNA fragment encoding the cytoskeleton protein, i.e. the actin gene (act1), from A. niger WU-2223L was labeled and used as the probe for the internal standard. Northern blot analysis was performed using Scion Image (Scion).
Preparation of cell-free extracts and enzymatic analysis
Cell-free extracts of A. niger strains were used for the enzymatic analysis of NAD+-ICDH, NADP+-ICDH, and isocitrate lyase (ICL; EC 4.1.3.1). Powdered mycelia were suspended in 50 mm KH2PO4-K2HPO4 buffer (pH 7.0) and centrifuged (15,000 × g, 4 °C, 20 min) for the removal of mycelial debris, and the resulting cell-free extracts were used for enzymatic analyses. The protein concentration in the cell-free extract was determined using the Coomassie Protein Assay Reagent (Thermo Fisher Scientific, Waltham, MA) with bovine serum albumin as the standard. NAD+-ICDH and NADP+-ICDH activities were measured according to the methods reported by KornbergCitation38) and Kornberg,Citation39) respectively, by following the reduction rate of NADP+ and NAD+, respectively, at 340 nm. The ICL activity was measured according to the method reported by Dixon et al.Citation40) by following the rate of glyoxylate phenylhydrazone formation at 324 nm. These reactions were monitored spectrophotometrically by using a U-3010 spectrophotometer (Hitachi, Tokyo).
Transcriptional analyses by RT-PCR
The transcript levels of genes encoding enzymes related to isocitrate metabolism in A. niger WU-2223L and OPI-1, such as NADP+-ICDH, NAD+-ICDH, and ICL, were analyzed. cDNA was synthesized using total RNA isolated by PrimeScript First Strand cDNA Synthesis Kit (Takara Bio, Shiga, Japan) according to the manufacturer’s protocol. The analyzed genes and the specific primers used for these genes are listed in Table . The act1 gene was used as an internal standard. Quantitative analysis of transcriptional levels was performed using Scion Image (Scion).
Table 1. Primers used for transcriptional analyses.
Measurement of intracellular organic acids
Intracellular organic acids were isolated from the mycelia of A. niger by using an alkaline extraction method.Citation41) The intracellular volume was calculated according to the method reported by Ruijter et al.Citation42) The detection and measurement of intracellular organic acids were performed using the HPLC LC-20 system (Shimadzu, Kyoto, Japan) equipped with a Shim-pack SCR-101H (particle size, 10 μ m; 300 × 7.9 mm I.D.) column (Shimadzu) and photodiode array detector SPD-M20A at 210 nm (Shimadzu). HClO4 (4 mm) was used as the mobile phase, under the conditions with a flow rate of 0.7 mL/min and the column temperature at 60 °C.
Determination of mycelial dry weight and extracellular citric acid and glucose in the culture broth
The mycelial dry weights, citric acid, and glucose in the culture broth were determined according to methods described previously.Citation24,28–32,37) The culture filtrate was recovered by filtering the culture broth through a glass microfiber filter (GF/A) and qualitative paper filter No. 4. The residual mycelia on the filter paper were dried at 105 °C, and the mycelial dry weight was determined as described previously.Citation43) The culture filtrate containing citric acid and glucose was analyzed using the HPLC LC-20 system (Shimadzu) equipped with a Shim-pack SCR-101H column (Shimadzu). For the quantification, the refractive index detector RID-10A (Shimadzu) for saccharides containing glucose and photodiode array detector SPD-M20A at 210 nm (Shimadzu) for organic acids containing citric acid were used, and 4 mm HClO4 was used as the mobile phase, under the conditions with a flow rate of 0.7 mL/min and the column temperature at 60 °C. Concentrations of citric acid were also measured and reconfirmed using a fluorescent protein-based indicator method as described previously.Citation44)
Results
Construction of icdA-overexpressing strains
The icdA-overexpressing strains derived from A. niger WU-2223L were constructed by the introduction of pPANICDH using the protoplast-PEG method. Fourteen transformants were isolated on CD agar plates containing 100 μg/mL pyrithiamine as the selection marker. The pPANICDH clones were confirmed by Southern blot analysis using an icdA fragment, which was amplified by PCR with the primers Pr3 and Pr4 as hybridization probes (Fig. ). Among the icdA-overexpressing strains obtained, 1 strain (OPI-1) retained 2 copies of pPANICDH in its chromosome and demonstrated the highest NADP+-ICDH specific activity. Therefore, OPI-1 was selected and used as a representative icdA-overexpressing strain for subsequent analyses.
Fig. 2. Southern Blot Analysis for the Confirmation of pPANICDH Introduction.
Note: OPI-1 and OPI-2 are pPANICDH transformants. Genomic DNAs were isolated from mycelia obtained after a 2-d cultivation and digested with SpeI and HindIII. Lane M, λ/HindIII digest.
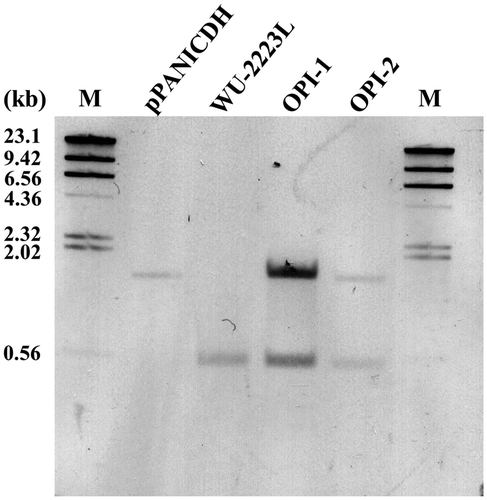
To examine the effects of defective NADP+-ICDH activity on citric acid production in A. niger, we attempted to construct icdA disruptants using strain DKL-2P (∆kueA, pyrG-::pyrG), a kueA disruptant that shows high gene-targeting frequencies, which was constructed by inserting WU-2223L-derived pyrG into the genomic DNA of the host strain DKL-2.Citation30) Although more than 50 times we have done experiments of transformation with objective to construct icdA disruptants using several media such as those containing sodium glutamate and α-ketoglutarate, we have not yet obtained icdA disruptants (details not shown). The reason why icdA disruptants were not generated is unclear, but it seems likely that the disruption of icdA is lethal for citric acid-producing A. niger WU-2223L, in contrast to other microorganisms such as E. coliCitation44) and S. cerevisiae.Citation15)
Transcription of icdA and activities of ICDHs
To confirm the overexpression of icdA, Northern blot analysis was performed using total RNAs of WU-2223L and OPI-1 isolated from mycelia obtained after a 5-d cultivation in SLZ medium. Although no significant difference was observed in the level of actin gene (act1), the transcription level of icdA in OPI-1 was 12.5-fold greater than that in WU-2223L (Fig. ).
Fig. 3. Northern Blot Analysis of mRNAs from Mycelia Obtained after a 5-d Cultivation under Citric Acid-Producing Conditions.
Note: The actin gene (act1) and the NADP+-ICDH gene (icdA) were used as specific probes. The relative values of transcriptional levels (icdA/act1) are 0.2 (1.0) and 2.5 (12.5) for WU-2223L and OPI-1, respectively; the transcription level values relative to that of WU-2223L are shown in parentheses. Because of the strong expression of both the mitochondrial and cytoplasmic ICDH transcripts, the individual bands cannot be visualized separately.
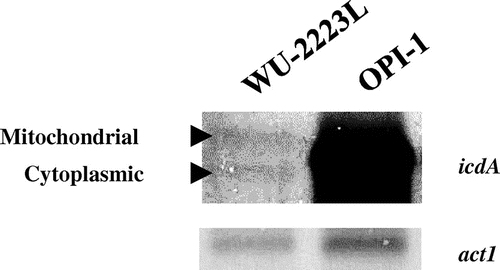
Next, the specific activity of NADP+-ICDH was measured using cell-free extracts of OPI-1 and WU-2223L cultivated in SLZ medium (Fig. (A) and (B)). Throughout the 12-d cultivation, the specific activity of NADP+-ICDH in OPI-1 was 3.20- to 4.78-fold greater than that in WU-2223L. On the 5th day of cultivation, the specific activity of NADP+-ICDH in OPI-1 reached a maximum of 4.78-fold greater (621 μmol/(min mg-protein)) than that in WU-2223L (130 μmol/(min mg-protein)). These data demonstrate that the increase of icdA transcription is accompanied by the enhancement of the specific activity of NADP+-ICDH through the forced expression of the gene. However, although the specific activity of NAD+-ICDH in OPI-1 was equivalent to that in WU-2223L (Fig. (B)), the specific activity of ICL in OPI-1 remained lower than that in WU-2223L (Fig. (C)).
Comparative transcriptional analysis of isocitrate-metabolizing enzyme genes
The time-dependent transcriptional changes in the genes encoding NADP+-ICDH, NAD+-ICDH, and ICL were analyzed by RT-PCR. The transcription level of icdA in OPI-1 remained higher than that in WU-2223L throughout the cultivation period (Fig. (A)). However, there was no significant difference in the expression of the idhA gene, which encodes NAD+-ICDH, for OPI-1 and WU-2223L (Fig. (B)). The transcription level of the icl1 gene, which encodes ICL, an enzyme that catalyzes the cleavage of isocitrate to succinate and glyoxylate, in OPI-1 remained lower than that in WU-2223L (Fig. (C)). Therefore, the glyoxylate cycle is poorly activated or slightly repressed in the icdA-overexpressing A. niger clone.
Fig. 5. RT-PCR Analyses of Genes in A. niger Strains Cultivated under Citric Acid-Producing Conditions.
Note: The relative intensity of the actin gene (act1) from WU-2223L obtained after a 3-d cultivation is represented as 1.0. A, NADP+-ICDH gene (icdA). B, NAD+-ICDH gene (idhA); C, ICL gene (icl1). The quantitative analysis of the transcriptional levels was conducted with Scion Image (Scion).
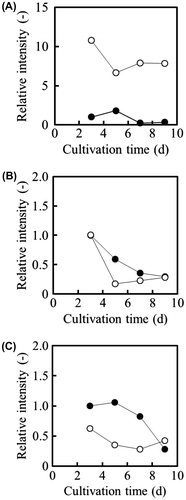
Analysis of intracellular organic acids
We measured the intracellular concentrations of organic acids in A. niger WU-2223L and OPI-1 to confirm the effects of icdA overexpression on metabolites (Table ). On the 7th day of cultivation, the intracellular concentrations of citric acid (47.3 mm) and malic acid (13.5 mm) in OPI-1 were lower than those in WU-2223L (61.8 and 37.2 mm, respectively). However, on the 3rd and 7th days of cultivation, the concentration of α-ketoglutaric acid in OPI-1 was higher than that in WU-2223L. Notably, only trace amounts of isocitric acid were detected in both WU-2223L and OPI-1 at the same periods of cultivation.
Table 2. Intracellular organic acids in the mycelia of A. niger WU-2223L and OPI-1.
Citric acid production by OPI-1
To confirm the effects of icdA overexpression on extracellular citric acid production by A. niger, WU-2223L and OPI-1 were cultivated in SLZ medium for 12 d. In the culture broth, the concentrations of citric acid produced and glucose consumed by OPI-1 were lower than those by WU-2223L throughout the 12-d cultivation period (Fig. ). On the 12th day, the concentrations of citric acid produced (52.5 g/L) and glucose consumed (97.6 g/L) by OPI-1 were 81.3 and 89.5% of those by WU-2223L (64.6, 109.1 g/L), respectively. Therefore, the yield of citric acid produced based on glucose consumed by WU-2223L and OPI-1 were 59.2 and 53.8% (w/w), respectively. The mycelial dry weight of OPI-1 (15.1 g/L) increased by 13% compared with that of WU-2223L (13.4 g/L). Moreover, citric acid productivity, defined as the ratio of the amount of citric acid to mycelial dry weight, for OPI-1 (3.5 g/g) decreased by 27.1% compared with that for WU-2223L (4.8 g/g).
Fig. 6. Time Courses of Citric Acid Production by A. niger WU-2223L and OPI-1.
Note: The time courses of glucose consumption (○, ●) and citric acid production (△, ▲) by A. niger WU-2223L (solid) and A. niger OPI-1 (hollow) are displayed. The average and standard deviation values (I) of three individual cultivations are shown.
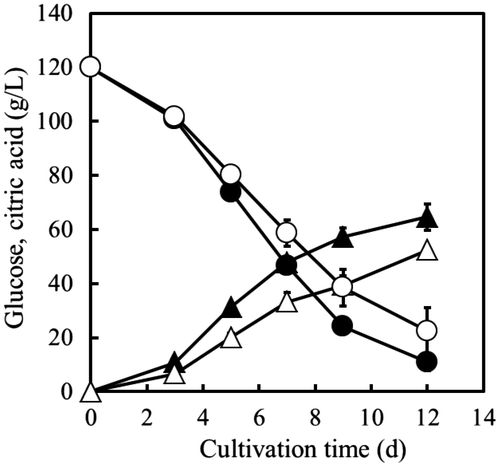
Discussion
To our knowledge, this study is the first time to show the relationship between NADP+-ICDH-specific activity changes and citric acid production in A. niger induced by metabolic engineering. Briefly, we constructed an icdA-overexpressing strain, OPI-1, derived from A. niger WU-2223L and examined the citric acid production-related metabolic differences between OPI-1 and WU-2223L.
In OPI-1, the overexpression of icdA increased the transcription level of icdA and the specific activity of NADP+-ICDH by 12.5-fold (Fig. ) and 4.78-fold (Fig. (A)), respectively, compared with those in WU-2223L. However, both the transcription of idhA and the specific activity of NAD+-ICDH did not appear to be affected by the forced expression of icdA (Figs. (B) and (B)). These results suggest that the expression of the NAD+-ICDH gene might not be regulated by NADP+-ICDH gene transcription level changes, although both NADP+-ICDH and NAD+-ICDH receive isocitric acid as a substrate.
The specific activity of ICL in OPI-1 remained at a lower level than that in WU-2223L throughout the cultivation period (Fig. (C)), suggesting that the glyoxylate cycle, a bypass of the TCA cycle (Fig. ), was suppressed in the ICDH-overproducing clone. The suppression of ICL transcription was accompanied with an increase in NADP+-ICDH activity (Fig. (C)). In the case of OPI-1, an increase in intracellular α-ketoglutaric acid and a decrease in intracellular malic acid, compared with those in WU-2223L, were observed (Table ). These results indicate differences in the metabolic activities of the TCA cycle and the glyoxylate cycle for OPI-1 and WU-2223L.
Moreover, we confirmed that the increase in NADP+-ICDH-specific activity via the overexpression of the icdA gene caused a decrease in citric acid production and glucose consumption in A. niger (Fig. ). These results are consistent with the hypothesis that increase in NADP+-ICDH-specific activity accelerates the metabolism of isocitric acid via the TCA cycle and suppresses the glycolytic pathway. In OPI-1, the intracellular concentrations of citric acid and α-ketoglutaric acid were lower and higher, respectively, than those in WU-2223L (Table ), strongly supporting the conclusion that the manipulation of NADP+-ICDH activity by genetic engineering is an effective method for altering citric acid production in A. niger.
In general, ICDH is a key enzyme that regulates the TCA cycle, which is exemplified by the role of NAD+-ICDH in S. cerevisiae.Citation15) However, in A. niger, the alterations in the specific activity of NADP+-ICDH, but not NAD+-ICDH, are related to the extremely high production of citric acid.Citation25,27–29) In addition, neither the gene expression nor the enzymatic activity of NAD+-ICDH are affected by the increases in the transcript level of the NADP+-ICDH gene and the specific activity of its product. Our present study also suggests that NADP+-ICDH may work independently of NAD+-ICDH as one of the TCA cycle regulatory enzymes, especially in relation to citric acid production by A. niger.
In this study, we demonstrated the relationship between NADP+-ICDH-specific activity changes and citric acid production in A. niger by metabolic engineering for the first time. Based on results in this study, increase of citric acid production through NADP+-ICDH activity by down-regulation of icdA might be expected. Moreover, in some cases, the acceleration of the metabolism through TCA cycle by up-regulation of icdA resulting in decrease of citric acid production might contribute to production of other metabolites except for citric acid by using A. niger as a host. Thus, the approach to examine the effects of suppression or repression of icdA, by some genetic engineering methods, on citric acid production is at present under investigation (unpublished). In conclusion, this study shows that icdA is a potentially valuable tool, via metabolic engineering, for the control of citric acid production in A. niger.
Funding
This work was partially supported by Grants-in-Aid for Scientific Research [grant number 25450114] and by the Grants for Excellent Graduate Schools (Practical Chemical Wisdom) from the Ministry of Education, Culture, Sports, Science and Technology (MEXT), Japan.
Notes
Abbreviations: CD; Czapek-Dox; ICL; isocitrate lyase; LB, Luria–Bertani; NAD+-ICDH, NAD+-specific isocitrate dehydrogenase; NADP+-ICDH, NADP+-specific isocitrate dehydrogenase; RT-PCR, reverse-transcription PCR.
References
- Suzuki M, Sahara T, Tsuruha J, Takada Y, Fukunaga N. Differential expression in Escherichia coli of the Vibrio sp. strain ABE-1 icdI and icdII genes encoding structurally different isocitrate dehydrogenase isozymes. J. Bacteriol. 1995;177:2138–2142.
- Zhao WN, McAlister-Henn L. Expression and gene disruption analysis of the isocitrate dehydrogenase family in yeast. Biochemistry. 1996;35:7873–7878.10.1021/bi9605189
- Cohen GN. Microbial biochemistry. 2nd ed. Springer Science+Business Media B.V., Dordrecht; 2011. p. 86–88.10.1007/978-90-481-9437-7
- Plaut GWE. PD Boyer, K Lardy, and Myerback K, editors. The enzymes, Academic Press, New York, NY; 1963. Isocitrate dehydrogenases; p. 105–126.
- Borthwick AC, Holms WH, Nimmo HG. Isolation of active and inactive forms of isocitrate dehydrogenase from Escherichia coli ML 308. Eur J. Biochem. 1984;141:393–400.10.1111/ejb.1984.141.issue-2
- Martinez-Rivas JM, Vega JM. Purification and characterization of NAD-isocitrate dehydrogenase from Chlamydomonas reinhardtii. Plant Physiol. 1998;118:249–255.10.1104/pp.118.1.249
- Kawachi H, Shimizu K, Atomi H, Sanuki S, Ueda M, Tanaka A. Gene analysis of an NADP-linked isocitrate dehydrogenase localized in peroxisomes of the n-alkane-assimilating yeast Candida tropicalis. Eur. J. Biochem. 1997;250:205–211.10.1111/ejb.1997.250.issue-1
- Keys DA, McAlister-Henn L. J. Bacteriol. Subunit structure, expression, and function of NAD(H)-specific isocitrate dehydrogenase in Saccharomyces cerevisiae. 1990;172:4280–4287.
- Cupp JR, McAlister-Henn L. NAD(+)-dependent isocitrate dehydrogenase. Cloning, nucleotide sequence, and disruption of the IDH2 gene from Saccharomyces cerevisiae. J. Biol. Chem. 1991;266:22199–22205.
- Cupp JR, McAlister-Henn L. Cloning and characterization of the gene encoding the IDH1 subunit of NAD(+)-dependent isocitrate dehydrogenase from Saccharomyces cerevisiae. J. Biol. Chem. 1992;267:16417–16423.
- Haselbeck RJ, McAlister-Henn L. Isolation, nucleotide sequence, and disruption of the Saccharomyces cerevisiae gene encoding mitochondrial NADP(H)-specific isocitrate dehydrogenase. J. Biol. Chem. 1991;266:2339–2345.
- Henke B, Gizalsky W, Berteaux-Lecellier V, Erdmann R. IDP3 encodes a peroxisomal NADP-dependent isocitrate dehydrogenase required for the β-oxidation of unsaturated fatty acids. J. Biol. Chem. 1998;273:3702–3711.10.1074/jbc.273.6.3702
- van Roermund CWT, Hettema EH, Kal AJ, Van den Berg M, Tabak HF, Wanders RJA. Peroxisomal β-oxidation of polyunsaturated fatty acids in Saccharomyces cerevisiae: isocitrate dehydrogenase provides NADPH for reduction of double bonds at even positions. EMBO J. 1998;17:677–687.10.1093/emboj/17.3.677
- Loftus TM, Hall LV, Anderson SL, McAlister-Henn L. Isolation, characterization, and disruption of the yeast gene encoding cytosolic NADP-specific isocitrate dehydrogenase. Biochemistry. 1994;33:9661–9667.10.1021/bi00198a035
- Contreras-Shannon V, Lin AP, McCammon MT, McAlister-Henn L. Kinetic properties and metabolic contributions of yeast mitochondrial and cytosolic NADP+-specific isocitrate dehydrogenases. J. Biol. Chem. 2004;280:4469–4475.10.1074/jbc.M410140200
- F Schauer, R Borriss. In: Tkacz J, Lene L, editors. Advances in fungal biotechnology for industry, agriculture, and medicine. New York, NY: Kluwer Academic/Plenum Publishers; 2004. 11, Biocatalysis and Biotransformation; p. 237–306.
- Usami S. Production of citric acid by submerged culture. Mem. Sch. Eng. Waseda Univ. 1978;42:17–26.
- Karaffa L, Kubicek CP. Aspergillus niger citric acid accumulation: do we understand this well working black box? Appl. Microbiol. Biotechnol. 2003;61:189–196.10.1007/s00253-002-1201-7
- Demain AL, Sanchez S. In: El-Mansi EMT, Bryce CFA, Dahhou B, Sanchez S, Demain AL, editors. Fermentation Microbiology and Biotechnology. Boca Raton: CRC Press; 2011. 4, Microbial Synthesis of Primary Metabolites: Current Trends and Future Prospects; p. 77–100.
- Meixner-Monori B, Kubicek CP, Harrer W, Schreferl G, Rohr M. NADP-specific isocitrate dehydrogenase from the citric acid-accumulating fungus Aspergillus niger. Biochem. J. 1986;236:549–557.
- Tsekova K, Dentchev D, Todorova D. Effect of cadmium and copper on the production of citric acid by Aspergillus niger. Folia Microbiol. 2000;45:331–334.10.1007/BF02817556
- Bowes I, Mattey M. The effect of manganese and magnesium ions on mitochondrial NADP+-dependent isocitrate dehydrogenase from Aspergillus niger. FEMS Microbiol. Lett. 1979;6:219–222.10.1111/fml.1979.6.issue-4
- Agrawal PK, Bhatt CS, Viswanathan L. Inhibition of fungal NADP+-isocitrate dehydrogenase by citric acid. Z. Allg. Mikrobiol. 1983;23:403–406.10.1002/(ISSN)1521-4028
- Kirimura K, Yoda M, Kumatani M, Ishii Y, Kino K, Usami S. Cloning and expression of Aspergillus niger icdA gene encoding mitochondrial NADP+-specific isocitrate dehydrogenase. J. Biosci. Bioeng. 2002;93:136–144.10.1016/S1389-1723(02)80005-6
- Mattey M. Citrate regulation of citric acid production in Aspergillus niger. FEMS Microbiol. Lett. 1977;2:71–74.10.1111/fml.1977.2.issue-2
- Szczodrak J. Biosynthesis of citric acid in relation to the activity of selected enzymes of the Krebs cycle in Aspergillus niger mycelium. Eur. J. Appl. Microbiol. Biotechnol. 1981;13:107–112.10.1007/BF00499697
- Kirimura K, Kumagai K, Morisada S, Kawabe S, Usami S. The changes in enzyme activities during citric acid fermentation by Aspergillus niger in solid culture. Hakkokogaku. 1984;62:127–133. Japanese
- Hattori T, Honda Y, Kino K, Kirimura K. Expression of alternative oxidase gene (aox1) at the stage of single-cell conidium in citric acid-producing Aspergillus niger. J. Biosci. Bioeng. 2008;105:55–57.10.1263/jbb.105.55
- Honda Y, Hattori T, Kirimura K. Visual expression analysis of the responses of the alternative oxidase gene (aox1) to heat shock, oxidative, and osmotic stresses in conidia of citric acid-producing Aspergillus niger. J. Biosci. Bioeng. 2012;113:338–342.10.1016/j.jbiosc.2011.10.026
- Honda Y, Kobayashi K, Kirimura K. Increases in gene-targeting frequencies due to disruption of kueA as a ku80 homolog in citric acid-producing Aspergillus niger. Biosci. Biotechnol. Biochem. 2011;75:1594–1596.10.1271/bbb.110015
- Kirimura K, Yoda M, Usami S. Cloning and expression of the cDNA encoding an alternative oxidase gene from Aspergillus niger WU-2223L. Curr. Genet. 1999;34:472–477.10.1007/s002940050422
- Kirimura K, Yoda M, Ko I, Oshida Y, Miyake K, Usami S. Cloning and sequencing of the chromosomal DNA and cDNA encoding the mitochondrial citrate synthase of Aspergillus niger WU-2223L. J. Biosci. Bioeng. 1999;88:237–243.10.1016/S1389-1723(00)80003-1
- Chomczynski P, Sacchi N. Single-step method of RNA isolation by acid guanidinium thiocyanate-phenol-chloroform extraction. Anal. Biochem. 1987;162:156–159.10.1016/0003-2697(87)90021-2
- Sakamoto Y, Watanabe H, Nagai M, Nakade K, Takahashi M, Sato T. Lentinula edodes tlg1 encodes a thaumatin-like protein that is involved in lentinan degradation and fruiting body senescence. Plant Physiol. 2006;141:793–801.10.1104/pp.106.076679
- Ozeki K, Kanda A, Hamachi M, Nunokawa Y. Construction of a promoter probe vector autonomously maintained in Aspergillus and characterization of promoter regions derived from A. niger and A. oryzae genomes. Biosci. Biotechnol. Biochem. 1996;60:383–389.10.1271/bbb.60.383
- Kubodera T, Yamashita N, Nishimura A. Pyrithiamine resistance gene (ptrA) of Aspergillus oryzae: cloning, characterization and application as a dominant selectable marker for transformation. Biosci. Biotechnol. Biochem. 2000;64:1416–1421.10.1271/bbb.64.1416
- Kirimura K, Ogawa S, Hattori T, Kino K. Expression analysis of alternative oxidase gene (aox1) with enhanced green fluorescent protein as marker in citric acid-producing Aspergillus niger. J. Biosci. Bioeng. 2006;102:210–214.10.1263/jbb.102.210
- Kornberg A. In: Colowick SP, Kaplan NO, editors. Methods in Enzymology. vol. I. New York, NY: Academic Press; 1955. IV, Enzymes of Citric Acid Cycle; p. 705–706.
- Kornberg A. In: Colowick SP, Kaplan NO, editors. Methods in Enzymology. vol. I. New York, NY: Academic Press; 1955. IV, Enzymes of Citric Acid Cycle; p. 707–709.
- Dixon GH, Kornberg HL. Assay methods for key enzymes of the glyoxylate cycle. Biochem. J. 1959;72:3P.
- Jernejc K. Comparison of different methods for metabolite extraction from Aspergillus niger mycelium. Acta Chim. Slov. 2004;51:567–578.
- Ruijter GJG, Visser J. Determination of intermediary metabolites in Aspergillus niger. J. Microbiol. Methods. 1996;25:295–302.10.1016/0167-7012(95)00104-2
- Kirimura K, Nakajima I, Lee SP, Kawabe S, Usami S. Citric acid production by the diploid strains of Aspergillus niger obtained by protoplast fusion. Appl. Microbiol. Biotechnol. 1988;27:504–506.10.1007/BF00451621
- Honda Y, Kirimura K. Generation of circularly permuted fluorescent-protein-based indicators for in vitro and in vivo detection of citrate. PLoS One. 2013;8:e64597.10.1371/journal.pone.0064597
- Baba T, Ara T, Hasegawa M, Takai Y, Okumura Y, Baba M, Datsenko KA, Tomita M, Wanner BL, and Mori H. Construction of Escherichia coli K-12 in-frame, single-gene knockout mutants: the Keio collection. Mol. Syst. Biol., 2, 2006 2008 (2006).