Abstract
We evaluated the substrate specificities of four proline cis-selective hydroxylases toward the efficient synthesis of proline derivatives. In an initial evaluation, 15 proline-related compounds were investigated as substrates. In addition to l-proline and l-pipecolinic acid, we found that 3,4-dehydro-l-proline, l-azetidine-2-carboxylic acid, cis-3-hydroxy-l-proline, and l-thioproline were also oxygenated. Subsequently, the product structures were determined, revealing cis-3,4-epoxy-l-proline, cis-3-hydroxy-l-azetidine-2-carboxylic acid, and 2,3-cis-3,4-cis-3,4-dihydroxy-l-proline.
Hydroxyproline (Hyp) is found commonly in animal collagen and is an important constituent of collagen in triple helices, in which Pro-Hyp-Gly is the major repetitive unit. The second component of Hyp is formed by a post-translational, enzymatic oxygenation (hydroxylation) of a proline residue with prolyl 4-hydroxylase (EC 1.4.11.2, procollagen-proline 4-dioxygenase). Furthermore, prolyl 3-hydroxylase (EC 1.4.11.7, procollagen-proline 3-dioxygenase), a minor enzyme compared to 4-hydroxylase, is also involved in the post-translational modifications of some collagen types. So far, both the peptide-bound form and the free form of Hyp occur within the plant tissueCitation1–3) and microbial fermentation broth.Citation4–6) The absolute configurations of these Hyps have been identified as the following five forms: trans-4-l-Hyp [(2S,4R)-form], cis-4-l-Hyp [(2S,4S)-form], trans-3-l-Hyp [(2S,3S)-form], cis-3-l-Hyp [(2S,3R)-form], and cis-4-d-Hyp [(2R,4R)-form]. In addition to these Hyp forms, other proline derivatives are industrially useful as a chemical building block for pharmaceuticals such as glycosidase inhibitor,Citation7) and therefore extensive studies on the efficient synthesis of these compounds have been undertaken.Citation8–11)
Enzymatic oxygenation of chemicals is generally regarded as highly simple and selective, compared to conventional chemical synthesis, with regard to efficiency, yield, and safety. Therefore, this enzymatic method has attracted considerable interest from scientists and manufacturers. Indeed, a microbial fermentation production process for trans-4-l-Hyp,Citation12,13) cis-3-l-Hyp,Citation13) and cis-4-l-Hyp has been established. In these processes, highly selective microbial hydroxylases play a critical role in the direct oxygenation of l-proline. Recently, l-proline cis-4-hydroxylase from Sinorhizobium meliloti 1021Citation14) and N-acetyltransferase Mpr1 from Saccharomyces cerevisiae Σ1278b were applied in the production of N-acetyl-cis-4-l-Hyp.Citation15) Moreover, extensive screening of l-proline hydroxylase for more selective oxygenation of l-pipecolinic acid has been carried out on the basis of microbial genome information, and we reported cis-selective l-proline hydroxylases from actinomycetes.Citation16) We found that these hydroxylases catalyzed simultaneous formation of cis-4-l-Hyp and cis-3-l-Hyp from l-proline and cis-5-hydroxy-l-pipecolinic acid (cis-5-l-HPA) and cis-3-l-HPA from l-pipecolinic acid. Although the ratios of cis-4-l-Hyp vs. cis-3-l-Hyp and cis-5-l-HPA vs. cis-3-l-HPA using these enzymes were elucidated, further information on the substrate specificity of these enzymes toward diverse proline derivatives remains unclear. Here, we report on the substrate specificity of four cis-selective proline hydroxylases from rhizobia and actinomycetes, and confirm the structure of the resulting products.
To reveal the substrate specificity and the mechanism of substrate recognition of proline cis-hydroxylases derived from Mesorhizobium loti (MlP4H), S. meliloti (SmP4H), Streptosporangium roseum (SrPH), and Catenulispora acidiphila (CaPH), the following 15 imino- and pyridine compounds were tested: l-proline, l-pipecolinic acid, 3,4-dehydro-l-proline, l-prolinamide, cis-3-l-Hyp, trans-3-l-Hyp, l-thioproline, l-azetidine-2-carboxylic acid (l-2-AZC), l-3-AZC, 2-methyl-l-proline, d-proline, d-pipecolinic acid, picolinic acid, pyrrolidine, and piperidine. cis-3-l-Hyp were kindly provided by Kyowa Hakko Bio (Tokyo, Japan) and trans-3-l-Hyp was purchased from Sigma–Aldrich (St. Louis, MO, USA). All chemicals were obtained from Wako Pure Chemical Industries (Osaka, Japan) and Tokyo Chemical Industry (Tokyo, Japan), unless otherwise stated. The heterologous expression and purification of proline cis-hydroxylases have been described previously.Citation14,16) Briefly, the genes were individually expressed in Escherichia coli as C-terminal His-tag fusions using the pET system (Merck; Darmstadt, Germany) and each enzyme was purified by Ni2+ affinity chromatography using a HisTrap HP column (GE Healthcare; Little Chalfont, UK), followed by gel filtration chromatography using a PD-10 column (GE Healthcare). After that, all four enzymes were obtained in apparent homogeneity as judged by SDS-PAGE analysis (Fig. ).
Fig. 1. SDS-PAGE analysis of the purified proline cis-hydroxylases.
Notes: Lane M, molecular mass standards with size indicated; lane 1, MlP4H; lane 2, SmP4H; lane 3, SrPH; lane 4,CaPH.
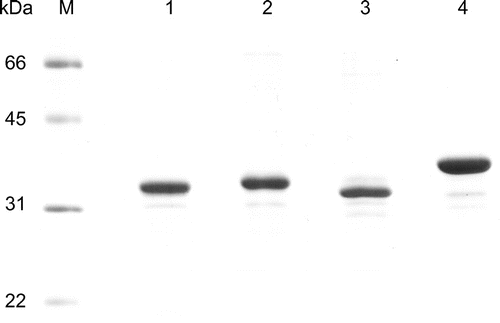
We initially evaluated the enzymes’ capacity to oxygenate 15 proline derivatives by the released succinate in reactions with purified enzyme, since substrate oxygenation is accompanied by oxidative decarboxylation of 2-oxoglutarate leading to succinate formation by 2-oxoglutarate-dependent proline cis-hydroxylases. The reaction mixture contained 5 mm substrate, 10 mm 2-oxoglutarate, 1 mm l-ascorbate, 0.5 mm FeSO4, and 0.1 mg/mL purified enzyme in a total volume of 0.5 mL. This was incubated for 20 h at the optimum temperature for each enzyme (MlP4H, 25 °C; SmP4H, 25 °C; SrPH, 15 °C; CaPH, 30 °C). Upon completion of the reaction, the quantity of released succinate was determined by an L-7000 series high performance liquid chromatography (HPLC) system (Hitachi High-Technologies; Tokyo, Japan) according to the following conditions: eluent, 0.1% (v/v) perchloric acid; flow rate, 0.5 mL/min; column, Inertsil ODS-3 (4.6 mm I.D. × 150 mm; GL Science; Tokyo, Japan); column oven, 40 °C; detection wavelength, 210 nm. The amount of succinate released is summarized in Fig. . These results show that all of the enzymes recognized l-proline, l-pipecolinic acid, 3,4-dehydro-l-proline, cis-3-l-Hyp, and l-thioproline as substrates. MlP4H, SmP4H, and CaPH were also possibly recognized l-2-AZC, but except for CaPH the amount of succinate was slight (less than 0.2 mm). In contrast, no succinate formation was observed for l-prolinamide, trans-3-l-Hyp, l-3-AZC, 2-methyl-l-proline, d-proline, d-pipecolinic acid, picolinic acid, pyrrolidine, and piperidine, suggesting that none of the enzymes recognized these compounds as substrates.
Fig. 2. Amounts of succinate released after enzymatic reaction of proline derivatives.
Notes: Unfilled, MlP4H; light grey, SmP4H; dark grey, SrPH; filled, CaPH. The average values are shown as bars with error values (standard deviation) obtained from three independent experiments.
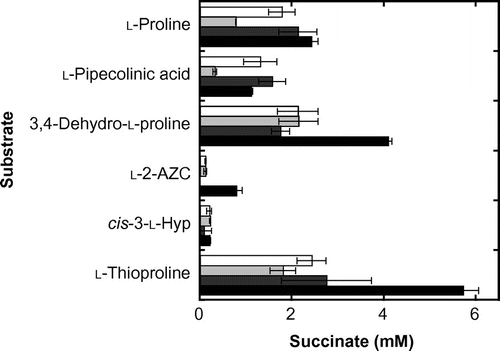
Next, we determined the chemical structures of the oxygenated proline derivatives. Imino groups of proline derivatives were labeled with Nα-(5-fluoro-2,4-dinitrophenyl)-l-alaninamide (FDAA)Citation17) and separated by HPLC according to the following conditions: eluent A, 50 mm KH2PO4 at pH 2.7, adjusted with 85% H3PO4/CH3OH/CH3CN (18/1/1, v/v/v); eluent B, 50 mm KH2PO4 at pH 2.7, adjusted with 85% H3PO4/CH3OH/CH3CN (12/1/7, v/v/v); linear gradient from 40 to 100% B (0–7 min) and then 100% B (7–15 min); flow rate, 1 mL/min; column, COSMOSIL 5C18-AR-II (4.6 mm I.D. × 150 mm; Nacalai Tesque; Kyoto, Japan); column oven, 40 °C; detection wavelength, 340 nm.
The oxygenated products of l-proline and l-pipecolinic acid were confirmed in the previous study.Citation14,16) cis-4-l-Hyp was formed from l-proline by MlP4H and SmP4H; cis-4-l-Hyp and cis-3-l-Hyp were simultaneously formed from l-proline by SrPH and CaPH. cis-3-l-HPA and cis-5-l-HPA were simultaneously formed from l-pipecolinic acid by all four enzymes with a different molar ratio.
The oxygenated product of 3,4-dehydro-l-proline using the four cis-hydroxylases was revealed to be cis-3,4-epoxy-l-proline—the retention time of the product was identical to that of cis-3,4-epoxy-l-proline, created using the l-proline cis-3-hydroxylase I from Streptomyces sp. TH1 as the standard.Citation18)
The oxygenated product of l-2-AZC was only detected in CaPH and revealed to be cis-3-hydroxy-l-2-AZC because the retention time of the product was identical to that of cis-3-hydroxy-l-2-AZC, created using the l-proline cis-3-hydroxylase I from Streptomyces sp. TH1 as the standard.Citation18) Although MlP4H and SmP4H may have a possibility of l-2-AZC oxygenation owing to the slight succinate formations (Fig. ), the reaction products were not detected by HPLC analysis.
The oxygenated product of cis-3-l-Hyp was unable to be determined because we could not obtain an authentic sample of this product. Therefore, the product was purified by preparative thin layer chromatography, and nuclear magnetic resonance (NMR) and mass spectrometry (MS) analyses were conducted. 1H NMR and 13C NMR spectra of dihydroxyproline were measured at 600 and 125 MHz in D2O on an Avance 600 (Bruker; Billerica, MA, USA), respectively. The 1H NMR (600 MHz, D2O) chemical shifts (δ) were as follows: 4.26 (1H, m, H-4), 4.21 (1H, dd, JH2,3 = JH3,4 = 3.7 Hz, H-3), 3.96 (1H, d, JH2,3 = 3.7 Hz, H-2), 3.35 (1H, dd, JH5a,5b = 12 Hz, JH4,5b = 7.8 Hz, H-5b), and 3.04 (1H, dd, JH5a,5b = 12 Hz, JH4,5a = 9.1 Hz, H-5a). The 13C-NMR (125 MHz, D2O) chemical shifts (δ) were as follows: 170.65 (C-1), 71.02 (C-3), 70.73 (C-4), 64.73 (C-2), and 47.15 (C-5). Electrospray ionization mass spectrometry (ESI-MS) analysis of the FDAA-labeled dihydroxyproline was performed on a LCQ-Fleet (Thermo Fisher Scientific; Waltham, MA, USA). The ESI-MS [M + H]+m/z = 399.95 (experiment) and 400.11 (calculated). The 1H NMR and 13C-NMR spectral data were almost identical to those previously reported for 2,3-cis-3,4-cis-3,4-dihydroxy-l-proline [(2S,3S,4R)-3,4-dihydroxyproline].Citation19) Thus, the oxygenated product of cis-3-l-Hyp was identified as 2,3-cis-3,4-cis-3,4-dihydroxy-l-proline (Fig. ).
Fig. 3. Summary of enzymatic oxygenations and corresponding products using proline cis-hydroxylases.
Notes: All reactions proceed in a 2-oxoglutarate-dependent manner.
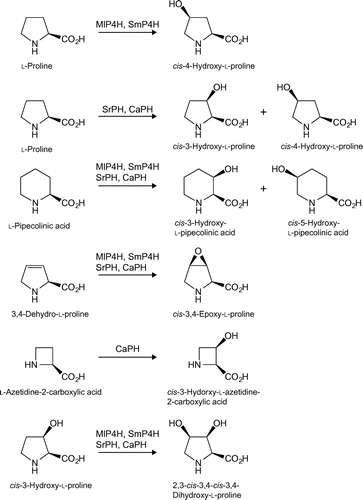
Finally, we attempted to determine the oxygenated product of l-thioproline using the four cis-hydroxylases. Although there was observable succinate formation during the initial screening (Fig. ) and a confirmed decrease in the substrate, the reaction products could not be detected using HPLC or MS analysis. Recently, 2-methylthioproline was reported to be hydrolytically unstable in aqueous solutions.Citation20) The authors suggested that the ampholytic nature of 2-methylthioproline affects its analysis with HPLC and MS. Our difficulty in detecting oxygenated products of l-thioproline was likely attributable to the same phenomena. However, the apparent decrease in substrate and formation of succinate were clearly confirmed by comparison with the enzyme-free control, and thus, the enzymes likely recognized l-thioproline as a substrate.
We revealed the substrate specificities of various proline cis-hydroxylases toward proline derivatives. The reactions and the corresponding products are summarized in Fig. . Analysis of the reactivity to 15 proline derivatives suggests that proline cis-hydroxylases recognized α-imino carboxylic acid moiety with an l-configuration. To our knowledge, this is the first report on the enzymatic synthesis of 2,3-cis-3,4-cis-3,4-dihydroxy-l-proline from cis-3-l-Hyp by direct oxygenation. In nature, several 3,4-dihydroxyproline isomers can be isolated from diatomic cell walls,Citation21) toxic peptides from mushrooms,Citation22) and animal adhesive proteins.Citation23) Because of their sugar-like structures, glycosidase inhibitors have been studied for their potential in biological and therapeutic applications.Citation24,25) For instance, 2,3-cis-3,4-cis-3,4-dihydroxy-l-proline shows potent and competitive inhibition of α-galactosidase.Citation26) Furthermore, the organic synthesis of various 3,4-dihydroxyproline isomers has been reported from easily available carbohydrates (e.g., pentose sugars)Citation27–32) and non-carbohydrates.Citation7) However, these methods still have limitations for industrial applications because complex reaction steps and multiple purification procedures result in an overall low yield.
Among four proline cis-hydroxylases, only CaPH oxygenated 2-AZC. Although the detailed mechanism of this specific recognition would be elucidated by enzyme–substrate complex analysis, it was revealed that CaPH lacks “Pro_3_hydrox_C” domain, which is a C-terminal region conserved in other cis-hydroxylases, by conserved domain search. This property would be considered as one of the reasons for the ability to oxygenate 2-AZC by CaPH.
In this paper, we demonstrated that proline cis-hydroxylases possess relaxed substrate specificity, and they have the potential to efficiently produce oxygenated proline derivatives in a one-step reaction. Furthermore, proline cis-hydroxylases are a class of 2-oxoglutarate-dependent dioxygenases that do not require expensive NAD(P)H or redox partner proteins. From an industrial point of view, 2-oxoglutarate is relatively cheap and is supplied from the more reasonably priced glucose through the metabolic pathway. Indeed, trans-4-l-Hyp and cis-3-l-Hyp can be produced by E. coli fermentation processes suggesting that the enzymes in this study have the capacity for adaptation to industrial use for oxygenation of various proline derivatives.
Acknowledgments
We would like to thank Kyowa Hakko Bio Co. Ltd. for kindly providing us with Hyp and HPA and for helpful discussions.
Funding
This work was financially supported by the Adaptable and Seamless Technology Transfer Program through target-driven R&D, JST (AS231157E).
Notes
Abbreviations: Hyp, Hydroxyproline; HPA, Hydroxypipecolinic acid; 2-AZC, Azetidine-2-carboxylic acid; HPLC, High performance liquid chromatography; FDAA, Nα-(5-Fluoro-2,4-dinitrophenyl)-l-alaninamide; NMR, Nuclear magnetic resonance; ESI-MS, Electrospray ionization mass spectrometry; MlP4H, l-Proline cis-4-hydroxylase from Mesorhizobium loti; SmP4H, l-Proline cis-4-hydroxylase from Sinorhizobium meliloti; SrPH, l-Proline cis-hydroxylase from Streptosporangium roseum; CaPH, l-Proline cis-hydroxylase from Catenulispora acidiphila.
References
- Kuttan R, Radhakrishnan AN. The biosynthesis of cis-4-hydroxy-l-proline in sandal (Santalum album L.). Biochem. J. 1970;117:1015–1017.
- Kuttan R, Radhakrishnan AN. Studies on bound trans-4-hydroxy-l-proline in sandal (Santalum album L.). Biochem. J. 1970;119:651–657.
- Kuttan R, Pattabhiraman KSV, Radhakrishnan AN. Possible chemotaxonomic significance of occurrence of cis-4-hydroxy-l-proline in Santalaceae. Phytochemistry. 1974;13:453–454.10.1016/S0031-9422(00)91231-7
- Matsuoka T, Furuya K, Serizawa N. Fermentative production of cis-4-hydroxy-(l)-proline by Helicocerus oryzae and Acrocylindrium oryzae. Biosci. Biotechnol. Biochem. 1994;58:1747–1748.10.1271/bbb.58.1747
- Serizawa N, Matsuoka T, Hosoya T, Furuya K. Fermentative production of trans-4-hydroxy-l-proline by Clonostachys cylindrospora. Biosci. Biotechnol. Biochem. 1995;59:555–557.10.1271/bbb.59.555
- Mimura H, Nagata S, Matsumoto T. Concentrations and compositions of internal free amino-acids in a halotolerant Brevibacterium sp. in response to salt stress. Biosci. Biotechnol. Biochem. 1994;58:1873–1874.10.1271/bbb.58.1873
- El-Ashry ESH, El Nemr A. Synthesis of mono- and di-hydroxylated prolines and 2-hydroxymethylpyrrolidines from non-carbohydrate precursors. Carbohydr. Res. 2003;338:2265–2290.10.1016/j.carres.2003.09.002
- Dangerfield EM, Gulab SA, Plunkett CH, Timmer MSM, Stocker BL. A fast, efficient and stereoselective synthesis of hydroxy-pyrrolidines. Carbohydr. Res. 2010;345:1360–1365.10.1016/j.carres.2010.03.016
- Mereyala HB, Pathuri G, Nagarapu L. Synthesis of (2S,4S)-4-hydroxyproline from D-glucose. Synth. Commun. 2012;42:1278–1287.10.1080/00397911.2010.539753
- Krishnamurthy S, Arai T, Nakanishi K, Nishino N. Epoxy amino acids produced from allylglycines intramolecularly cyclised to yield four stereoisomers of 4-hydroxyproline derivatives. R. Soc. Chem. Adv. 2014;4:2482–2490.
- Klein C, Huttel W Beilstein. Tertiary alcohol preferred: hydroxylation of trans-3-methyl-l-proline with proline hydroxylases. J. Org. Chem. 2011;7:1643–1647.
- Shibasaki T, Hashimoto S, Mori H, Ozaki A. Construction of a novel hydroxyproline-producing recombinant Escherichia coli by introducing a proline 4-hydroxylase gene. J. Biosci. Bioeng. 2000;90:522–525.
- Shibasaki T, Mori H, Ozaki A. Enzymatic production of trans-4-hydroxy-l-proline by regio- and stereospecific hydroxylation of l-proline. Biosci. Biotechnol. Biochem. 2000;64:746–750.10.1271/bbb.64.746
- Hara R, Kino K. Characterization of novel 2-oxoglutarate dependent dioxygenases converting l-proline to cis-4-hydroxy-l-proline. Biochem. Biophys. Res. Commun. 2009;379:882–886.10.1016/j.bbrc.2008.12.158
- Bach TM, Hara R, Kino K, Ohtsu I, Yoshida N, Takagi H. Microbial production of N-acetyl cis-4-hydroxy-l-proline by coexpression of the Rhizobium l-proline cis-4-hydroxylase and the yeast N-acetyltransferase Mpr1. Appl. Microbiol. Biotechnol. 2013;97:247–257.10.1007/s00253-012-4204-z
- Hara R, Uchiumi N, Kino K. Identification and characterization of 2-oxoglutarate-dependent dioxygenases catalyzing selective cis-hydroxylation of proline and pipecolinic acid from actinomycetes. J. Biotechnol. 2014;172:55–58.10.1016/j.jbiotec.2013.12.003
- Bhushan R, Bruckner H. Marfey's reagent for chiral amino acid analysis: a review. Amino Acids. 2004;27:231–247.10.1007/s00726-004-0118-0
- Shibasaki T, Sakurai W, Hasegawa A, Uosaki Y, Mori H, Yoshida M, Ozaki A. Substrate selectivities of proline hydroxylases. Tetrahedron Lett. 1999;40:5227–5230.10.1016/S0040-4039(99)00944-2
- Austin GN, Baird PD, Fleet GWJ, Peach JM, Smith PW, Watkin DJ. 3,6-Dideoxy-3,6-imino-1,2-O-isopropylidene-α-d-glucofuranose as a divergent intermediate for the synthesis of hydroxylated pyrrolidines: synthesis of 1,4-dideoxy-1,4-imino-l-gulitol, 1,4-dideoxy-1,4-imino-d-lyxitol, 2S,3S,4R-3,4-dihydroxyproline and (1S,2R,8S,8aR)-1,2,8-trihydroxyoctahydroindolizine [8-Epi-Swainsonine]. X-Ray crystal structure of (1S,2R,8S,8aR)-1,2,8-trihydroxy-5-oxo-octahydroindolizine. Tetrahedron. 1987;43:3095–3108.
- Reischl RJ, Bicker W, Keller T, Lamprecht G, Lindner W. Occurrence of 2-methylthiazolidine-4-carboxylic acid, a condensation product of cysteine and acetaldehyde, in human blood as a consequence of ethanol consumption. Anal. Bioanal. Chem. 2012;404:1779–1787.10.1007/s00216-012-6255-5
- Nakajima T, Volcani BE. 3,4-Dihydroxyproline: a new amino acid in diatom cell walls. Science. 1969;164:1400–1401.10.1126/science.164.3886.1400
- Buku A, Faulstich H, Wieland T, Dabrowski J. 2,3-trans-3,4-trans-3,4-Dihydroxy-l-proline: an amino acid in toxic peptides of Amanita virosa mushrooms. Proc. Nat. Acad. Sci. USA. 1980;77:2370–2371.10.1073/pnas.77.5.2370
- Taylor SW, Waite JH, Ross MM, Shabanowitz J, Hunt DF. trans-2,3-cis-3,4-Dihydroxyproline, a new naturally occurring amino acid, is the sixth residue in the tandemly repeated consensus decapeptides of an adhesive protein from Mytilus edulis. J. Am. Chem. Soc. 1994;116:10803–10804.10.1021/ja00102a063
- Asano N, Nash RJ, Molyneux RJ, Fleet GWJ. Sugar-mimic glycosidase inhibitors: natural occurrence, biological activity and prospects for therapeutic application. Tetrahedron: Asymmetry. 2000;11:1645–1680.10.1016/S0957-4166(00)00113-0
- el Ashry ES, Rashed N, Shobier AH. Glycosidase inhibitors and their chemotherapeutic value, Part 2. Pharmazie. 2000;55:331–348.
- Fleet GWJ, Nicholas SJ, Smith PW, Evans SV, Fellows LE, Nash RJ. Potent competitive-inhibition of α-galactosidase and α-glucosidase activity by 1,4-dideoxy-1,4-iminopentitols: syntheses of 1,4-dideoxy-1,4-imino-d-lyxitol and of both enantiomers of 1,4-dideoxy-1,4-iminoarabinitol. Tetrahedron Lett. 1985;26:3127–3130.10.1016/S0040-4039(00)98636-2
- Bashyal BP, Fleet GWJ, Gough MJ, Smith PW. Synthesis of the α-mannosidase inhibitors swainsonine [(1S, 2R, 8R, 8aR)-1,2,8-trihydroxyoctahydroindolizine] and 1,4-dideoxy-1,4-imino-d-mannitol from mannose. Tetrahedron. 1987;43:3083–3093.10.1016/S0040-4020(01)86850-2
- Weir CA, Taylor CM. Synthesis of a protected 3,4-dihydroxyproline from a pentose sugar. Org. Lett. 1999;1:787–789.10.1021/ol990763v
- Weir CA, Taylor CM. Synthesis of l-2,3-trans-3,4-cis-dihydroxyproline building blocks for peptide synthesis. J. Org. Chem. 1999;64:1554–1558.10.1021/jo982009d
- Defoin A, Sifferlen T, Streith J. Straightforward synthesis of pyrrolidine glycosidase inhibitors via asymmetric hetero-Diels-Alder reaction. Synlett. 1997;11:1294–1296.
- Taylor CM, Barker WD, Weir CA, Park JH. Toward a general strategy for the synthesis of 3,4-dihydroxyprolines from pentose sugars. J. Org. Chem. 2002;67:4466–4474.10.1021/jo025538x
- Moss WO, Bradbury RH, Hales NJ, Gallagher T. Ketene dithioacetals as 1,3-dipolarophiles. Applications to the synthesis of cyclic amino acids. J. Chem. Soc., Chem. Commun. 1990;1:51–53.10.1039/c39900000051