Abstract
Resveratrol was glucosylated to its 3- and 4′-β-glucosides by cultured cells of Phytolacca americana. On the other hand, cultured P. americana cells glucosylated pterostilbene to its 4′-β-glucoside. P. americana cells converted piceatannol into its 4′-β-glucoside. The 3- and 4′-β-glucosides of resveratrol were further glucosylated to 3- and 4′-β-maltosides of resveratrol, 4′-β-maltoside of which is a new compound, by cyclodextrin glucanotransferase. Resveratrol 3-β-glucoside and 3-β-maltoside showed low 2,2-diphenyl-1-picrylhydrazyl free-radical-scavenging activity, whereas other glucosides had no radical-scavenging activity. Piceatannol 4′-β-glucoside showed the strongest inhibitory activity among the stilbene glycosides towards histamine release from rat peritoneal mast cells. Pterostilbene 4′-β-glucoside showed high phosphodiesterase inhibitory activity.
Graphical Abstract
Stilbenes were glucosylated to the corresponding β-glucosides by cultured cells of Phytolacca americana. Stilbene glucosides were converted into β-maltosides by cyclodextrin glucanotransferase.
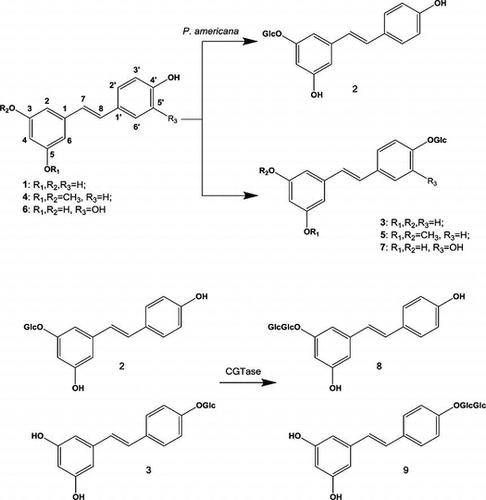
Key words:
Resveratrol (trans-3,4′,5-trihyrdoxystilbene) is the most widely studied stilbene and is found in fruit such as grape, berries, peanuts, and some medicinal plants. It exhibits anti-oxidative, anti-inflammatory, and anti-aging action and has chemopreventive effects in several biological systems.Citation1–4) Pterostilbene (trans-3,5-dimethoxy-4′-hydroxystilbene), which is a dimethyl ether analog of resveratrol, has been found in blueberries and grapes. It has been shown to exhibit anti-oxidative activity, to significantly affect cell proliferation and inflammation, and to reverse the effects of aging in rats.Citation5–7) On the other hand, piceatannol (3,3′,4,5′-trans-trihydroxystilbene), a naturally occurring hydroxylated analog of resveratrol, has been found in various plants, including grapes, passion fruit, white tea, and Japanese knotweed. It exhibits immunosuppressive, anti-oxidative, and antitumorigenic activities.Citation8–10) Despite the bio- and physiological activities of these stilbene compounds, their use as medicines has been limited, due to their insolubility in water and poor absorption after oral administration.
Plant-cultured cells can be used to convert organic molecules to more useful compounds by catalyzing hydrolysis, oxidation, reduction, esterification, isomerization, and glycosylation reactions. The glycosylation of bioactive compounds can enhance their water-solubility, physicochemical stability, intestinal absorption, and biological half-life, and improve their bio- and pharmacological properties.Citation11–18)
We report here the syntheses of glycosides of resveratrol, pterostilbene, and piceatannol by biocatalytic glycosylation using cultured cells of Phytolacca americana and cyclodextrin glucanotransferase (CGTase). In addition, we report the physiological properties of resveratrol, pterostilbene, piceatannol, and their glycosides, such as their 2,2-diphenyl-1-picrylhydrazyl (DPPH) free-radical-scavenging, anti-allergic, and phosphodiesterase (PDE) inhibitory activities.
Materials and methods
Analyses
The structures of some products were determined based on the analysis of HRFABMS, 1H- and 13C-nuclear magnetic resonance (NMR), H–H and C–H COSY, HMQC, and heteronuclear multiple bond correlation (HMBC) spectra. The 1H and 13C NMR, H–H and C–H COSY, HMQC, and HMBC spectra were recorded using a JNM-ECS400 spectrometer in CD3OD or dimethyl sulfoxide (DMSO)-d6 solutions, and the chemical shifts are expressed in δ (ppm) with reference to TMS. The fast atom bombardment mass spectroscopy (FABMS) spectra were measured using a JMS-700 MStation in CH3OH solution.
Cell line and culture conditions
P. americana cells were subcultured at four-week intervals on solid Murashige and Skoog (MS) medium (100 mL in a 300-mL conical flask) containing 3% sucrose, 10 mM 2,4-dichlorophenoxyacetic acid, and 1% agar (adjusted to pH 5.7) at 25 °C in the dark. A suspension culture was started by transferring the cultured cells to 100 mL of liquid medium in a 300-mL conical flask, and incubated on a rotary shaker (120 rpm) at 25 °C in the dark. Prior to use for this work, some of the callus tissue (fresh weight [fr. wt] 20 g) was transplanted to freshly prepared MS medium (100 mL in a 300-mL conical flask) and grown with continuous shaking for 2 days on a rotary shaker (120 rpm).
Biotransformation procedures
Substrates 1, 4, and 6 were purchased from Tokyo Chemical Industry Co., Ltd. The substrates, stilbenes, were individually biotransformed using plant-cultured cells as biocatalysts. To a 300-mL flask containing 100 mL of the culture medium and suspension-cultured cells (25 g) was added 15 mg of substrate. The culture was incubated at 25 °C for 2 days on a rotary shaker (120 rpm) in the dark. After the incubation period, the cells and medium were separated by filtration with suction. The filtered medium was extracted with ethyl acetate (EtOAc). The cells were extracted by homogenization with MeOH, and the resulting extract was concentrated. The residue was partitioned between H2O and EtOAc. The EtOAc layer was evaporated and the residue was re-dissolved in MeOH (3 mL) and purified by preparative high-performance liquid chromatography (HPLC) [column: CrestPak C18S; solvent: resveratrol and its glycosides: CH3CN:H2O = 15:85 (v/v); pterostilbene and its glycosides: CH3CN:H2O = 15:85 (v/v); piceatannol and its glycosides: CH3CN:H2O = 10:90 (v/v); flow rate: 1.0 mL/min; column temperature: 40 °C].
The spectral data of the product 7 are as follows.
Piceatannol 4′-β-glucoside (7): amorphous powder; NMR δH (CD3OD): 3.41–3.91 (6H, multiplet, H-2′′-H-6′′), 4.75 (1H, doublet, J = 7.2 Hz, H-1′′), 6.15 (1H, triplet, J = 2.0 Hz, H-4), 6.43 (2H, doublet, J = 2.0 Hz, H-2,6), 6.81 (1H, doublet, J = 16.4 Hz, H-7), 6.82 (1H, double-doublet, J = 8.0, 2.4 Hz, H-6′), 6.90 (1H, doublet, J = 16.4 Hz, H-8), 6.99 (1H, doublet, J = 2.0 Hz, H-2′), 7.11 (1H, doublet, J = 8.4 Hz, H-5′); NMR δC (CD3OD): see Table .
Table 1. 13C chemical shifts of the products 2, 3, 5, and 7–9 in CD3OD (7) or DMSO-d6 (2, 3, 5, 8, and 9).
Synthesis of β-maltosides of resveratrol
CGTase (Paenibacillus macerans) was purchased from Amano Enzyme Inc. Resveratrol 3-maltoside (8) and resveratrol 4′-β-maltoside (9) were produced individually as follows. To a solution containing resveratrol 3-β-glucoside (2) or resveratrol 4′-β-glucoside (3) (0.1 mmol) and α-cyclodextrin (0.5 mmol) in 50 mM citrate buffer solution (pH 5.6) was added 0.5 mL CGTase (600 units/mL). One U is defined as the amount of the enzyme that catalyzes the conversion of 1 micromole of substrate per minute. The reaction mixture was stirred at 40 °C for 24 h, and then extracted with n-butanol. The n-butanol extracts were purified by preparative HPLC [column: YMC packed C18; solvent CH3CN:H2O = 5:95; flow rate: 5.0 mL/min; column temperature: 40 °C].
The spectral data of the product 8 and 9 are as follows.
Resveratrol 3-β-maltoside (8): amorphous powder; HRFABMS m/z ([M + Na]+): Calcd. for C26H32O13Na: 575.1270, Found: 575.1255; NMR δH (DMSO-d6): 3.09–3.75 (12H, multiplet, H-2′′-H-6′′, H-2′′′-H-6′′′), 4.86 (1H, doublet, J = 8.8 Hz, H-1′′), 5.06 (1H, doublet, J = 3.6 Hz, H-1′′′), 6.30 (1H, triplet, J = 2.0 Hz, H-4), 6.53 (1H, broad-singlet, H-6), 6.68 (1H, broad-singlet, H-2), 6.69 (2H, doublet, J = 8.8 Hz, H-3′, 5′), 6.79 (1H, doublet, J = 16.8 Hz, H-7), 6.99 (1H, doublet, J = 16.8 Hz, H-8), 7.34 (2H, doublet, J = 8.8 Hz, H-2′, 6′); NMR δC (DMSO-d6): see Table .
Resveratrol 4′-β-maltoside (9): amorphous powder; HRFABMS m/z ([M + Na]+): Calcd. for C26H32O13Na: 575.1270, Found: 575.1259; NMR δH (DMSO-d6): 3.08–3.73 (12H, multiplet, H-2′′-H-6′′, H-2′′′-H-6′′′), 4.96 (1H, doublet, J = 7.6 Hz, H-1′′), 5.06 (1H, doublet, J = 4.4 Hz, H-1′′′), 6.11 (1H, triplet, J = 2.0 Hz, H-4), 6.38 (2H, doublet, J = 2.0 Hz, H-2, 6), 6.91 (1H, doublet, J = 16.8 Hz, H-7), 6.97 (1H, doublet, J = 16.8 Hz, H-8), 7.02 (2H, doublet, J = 8.8 Hz, H-3′, 5′), 7.51 (2H, doublet, J = 8.8 Hz, H-2′, 6′); NMR δC (DMSO-d6): see Table .
DPPH free-radical-scavenging activity
The DPPH free-radical-scavenging activities of resveratrol, pterostilbene, piceatannol, their β-glucosides, resveratrol 3-maltoside, and resveratrol 4′-β-maltoside were determined as follows.Citation19,Citation20) DPPH was dissolved in methanol (MeOH) (0.15 mM). The sample solutions were prepared by dissolving each compound in MeOH (2 mM). To a solution containing various concentrations of each sample (0.5 mL, 0.002–0.5 mM) was added DPPH solution (0.5 mL) at room temperature. After 30 min at 25 °C, the absorbance was measured at 517 nm. The percentage reduction of the initial DPPH adsorption, i.e. the free-radical-scavenging activity, was calculated as follows: E = [(Ac − At)/Ac] × 100, where At and Ac are the absorbance at 517 nm for sample solutions with and without the test compounds, respectively. Antioxidant activity was expressed as the 50% inhibitory concentration (IC50).
Effects of stilbenes and their glycosides on histamine release from rat peritoneal mast cells
The effects of resveratrol, pterostilbene, piceatannol, and their glycosides on compound 48/80-induced histamine release from rat peritoneal mast cells were examined as follows.Citation21–23) Peritoneal mast cells were collected from the abdominal cavities of rats (Male Wistar rats, Nippon SLC) and purified to a level higher than 95% according to the method described previously.Citation21–23) The purified mast cells were suspended in a physiological buffered solution (PBS) containing 145 mM NaCl, 2.7 mM KCl, 1.0 mM CaCl2, 5.6 mM glucose, and 20 mM 4-(2-hydroxyethyl)-1-piperazineethanesulfonic acid (HEPES) (pH 7.4) to give approximately 104 mast cells/mL. Cell viability was always greater than 90% as judged by the trypan blue exclusion test. Mast cells were preincubated with the test compound (1 μM) for 15 min at 37 °C, and subsequently exposed to compound 48/80 at 0.35 μg/mL. Histamine release was determined by a fluorometric assay as described previously,Citation21–23) and was expressed as a percentage of total histamine.
Effects of stilbenes and their glycosides on PDE activity
BIOMOL Green, which is used for calculation of the amount of phosphate released, was purchased from Funakoshi Co. PDE activity was measured using a cyclic nucleotide PDE assay kit from Enzo Life Sciences (BML-AK800) according to the manufacturer’s instructions, except that PDE 100 mU per well were used.Citation24–26) Stock solutions of inhibitors were prepared as 100 mM in DMSO and diluted to the appropriate concentrations. In the assay, 1 μL of each inhibitor solution was added before the addition of PDE enzyme solution. 3-Isobutyl-1-methylxanthine (IBMX) was used as a positive control. The PDE reaction was conducted at 37 °C for 60 min and terminated by the addition of BIOMOL Green and further incubation at room temperature for 30 min. The color reaction was measured by reading OD at 620 nm with a Multiscan FC (Thermo Scientific).
Results and discussion
After cultured cells of P. americana were incubated with resveratrol (1) for 2 days, the glycosylated products 2 and 3 were isolated from the cells by extraction with MeOH. Based on the results of FABMS, 1H and 13C NMR (Table ), and HMBC analyses, the products were determined to be resveratrol 3-β-glucopyranoside (2, 35%) and resveratrol 4′-glucoside (3, 22%) (Fig. ). The FABMS spectrum of 2 showed a pseudomolecular ion [M + Na]+ peak at m/z 413. The 1H NMR spectrum of 2 had a signal at δ 4.80 (1H, d, J = 7.6 Hz) corresponding to its attachment to the anomeric carbon (C-1′). The 13C NMR spectrum of 2 showed 20 carbon signals including the anomeric carbon signal at δ 100.6. Based on the coupling pattern of the proton signals and the chemical shifts of the carbon resonances due to the sugar moiety, the sugar component in 2 was concluded to be β-glucopyranose. Structure of the product 2 was identified by direct comparison of their NMR spectra with the reported data.Citation27) Thus, the structure of 2 was determined to be resveratrol 3-β-glucopyranoside (glucoside). The MS spectrum of the product 3 showed a [M + Na]+ peak at m/z 413. Based on the coupling pattern of the proton signals and the chemical shifts of the carbon resonances due to the sugar moiety, the sugar component in 3 was concluded to be β-glucopyranose. The 1H NMR spectrum of 3 had a proton signal at δ 4.88 (1H, d, J = 7.6 Hz, H-1′), and an analysis of 13C NMR data revealed anomeric carbon resonance at δ 100.2. Structure of the product 3 was identified by direct comparison of their NMR spectra with the reported data.Citation27) Thus, compound 3 was determined to be resveratrol 4′-β-glucopyranoside (glucoside).
Pterostilbene (4) was subjected to the same biotransformation system. Glycoside product 5 was obtained from the MeOH extracts of the cells. The product was identified as pterostilbene 4′-β-glucopyranoside (5, 7%). The MS spectrum of the product 5 showed a [M + Na]+ peak at m/z 441. The sugar component in 5 was concluded to be β-glucopyranose based on the coupling pattern of the proton signals and the chemical sifts of the carbon resonances due to the sugar moiety. The 1H NMR spectrum of 5 had a signal at δ 4.92 (1H, d, J = 7.2 Hz) corresponding to its attachment to the anomeric carbon (C-1′), and the 13C NMR spectroscopic data of 5 showed an anomeric carbon signal at δ 102.5 (Table ). The structure of the product 5 was identified by direct comparison of its NMR spectra with the reported data.Citation28) Thus, compound 5 was determined to be pterostilbene 4′-β-glucopyranoside (glucoside).
After piceatannol (6) was incubated for 2 days with cultured cells of P. americana, the product 7 was isolated from the cells by extraction with MeOH. No additional conversion products were detected in the MeOH extracts of the cells. Based on the MS, 1H, 13C NMR (Table ), and HMBC spectra, the product was identified as piceatannol 4′-β-glucopyranoside (7, 77%). The MS spectrum of 7 showed a pseudomolecular ion [M + Na]+ peak at m/z 429. The 1H NMR spectrum of 7 showed anomeric proton signals at δ 4.78 (1H, d, J = 7.6 Hz). The 13C NMR spectrum of 7 showed 20 carbon signals including an anomeric carbon signal at δ 102.0 (Table ). Based on the coupling pattern of the proton signals and the chemical shifts of the carbon resonances due to the sugar moiety, the sugar component in 7 was determined to be β-glucopyranose. Recently, we have reported that the glucosyltransferase from cultured cells of P. americana glucosylated piceatannol (6) to its 4′-glucoside (7) in the presence of UDP-glucose.Citation29) Here, we report the convenient synthesis of piceatannol 4′-glucoside (7) from piceatannol (6) by cultured cells of P. americana without costly co-factor, i.e. UDP-glucose. The NMR spectral data of 7 were in good agreement with the previously reported data of piceatannol 4′-β-glucoside.Citation29) Thus, the structure of 7 was determined to be piceatannol 4′-β-glucopyranoside (glucoside).
Next, resveratrol 3-β-glucoside (2) and resveratrol 4′-β-glucoside (3) were further glycosylated by CGTase to give compounds 8 and 9 in respective yields of 17 and 27%. The structures of products 8 and 9 were identified as resveratrol 3-β-maltoside (8) and resveratrol 4′-β-maltoside (9), which has not been reported previously, by HRFABMS, 1H and 13C NMR, H–H and C–H COSY, HMQC, and HMBC spectra. The HRFABMS spectra of 8 and 9 included a pseudomolecular ion [M + Na]+ peak at m/z 575.1255 for 8 and m/z 575.1259 for 9, indicating that each of these products consisted of one substrate and two hexoses. The sugar components in these products were determined to be glucose on the basis of the chemical shifts of their carbon signals. The 1H NMR spectra showed two proton signals at δ 4.86 (1H, d, J = 8.8 Hz) and δ 5.06 (1H, d, J = 3.6 Hz) for 8, and at δ 4.96 (1H, d, J = 7.6 Hz) and δ 5.06 (1H, d, J = 4.4 Hz) for 9, indicating that the glucoside linkage in these compounds had both α- and β-orientations. The HMBC spectra of 8 and 9 included correlations between the proton signal at δ 5.06 (H-1′′′) and the carbon signal at δ 79.2 (C-4′′) and between the proton signal at δ 4.86 (H-1′′) and the carbon signal at δ 158.1 (C-3) for 8, and between the proton signal at δ 5.06 (H-1′′′) and the carbon signal at δ 79.2 (C-4′′) and between the proton signal at δ 4.96 (H-1′′) and the carbon signal at δ 156.8 (C-4′) for 9. These data indicate that 8 and 9 were β-maltosyl analogs of 1, the sugar moiety of which was attached to the 3-position of 8 and the 4′-position of 9. Thus, the structures of products 8 and 9 were determined to be resveratrol 3-β-maltoside (8) and resveratrol 4′-β-maltoside (9), which is a new compound. Recently, synthesis of resveratrol 3-maltoside (8) by glycosylation of resveratrol 3-β-glucoside (2) with amylosucrase from Alteromonas macleodii deep ecotype has been reported.Citation30) The 13C NMR spectrum of compound 8 synthesized in the present study completely agreed with the previously reported data of resveratrol 3-maltoside,Citation30) identifying that the chemical structure of 8 is resveratrol 3-maltoside.
The results of this experiment revealed that cultured cells of P. americana can convert the stilbene compounds, such as resveratrol (1), pterostilbene (4), and piceatannol (6), into the corresponding β-glucosides (2, 3, 5, and 7), which are then accumulated in the cells. It is well known that glycosylation by plant-cultured cells can detoxify phenolic compounds which could arise from either the normal plant metabolism or the environment. The present results suggest that high concentrations of exogenous compounds, i.e. resveratrol (1), pterostilbene (4), and piceatannol (6), act as chemical stress toward cultured P. americana cells. The plant enzymes that are responsible for these biotransformations should be useful for preparing more water-soluble derivatives of stilbene compounds. This procedure is fairly simple and environmentally friendly.
The anti-oxidative activities of resveratrol (1), pterostilbene (4), piceatannol (6), and their glycosides, i.e. resveratrol 3-β-glucoside (2), resveratrol 4′-β-glucoside (3), pterostilbene 4′-β-glucoside (5), piceatannol 4′-β-glucoside (7), resveratrol 3-β-maltoside (8), and resveratrol 4′-β-maltoside (9), were determined by an in vitro bioassay of their DPPH free-radical-scavenging activity. The anti-oxidative activities were expressed as IC50 values and are summarized in Table . Resveratrol (1), resveratrol 3-β-glucoside (2), pterostilbene (4), and piceatannol (6) showed DPPH free-radical-scavenging activity, whereas resveratrol 3-β-maltoside (8) had low-anti-oxidative activity. On the other hand, resveratrol 4′-β-glucoside (3), pterostilbene 4′-β-glucoside (5), piceatannol 4′-β-glucoside (7), and resveratrol 4′-β-maltoside (9) had no anti-oxidative activity (Table ).
Table 2. Antioxidant activities of compounds 1–9.
Thus, the three stilbene compounds, i.e. resveratrol (1), resveratrol 3-β-glucoside (2), pterostilbene (4), and piceatannol (6), had strong DPPH free-radical-scavenging activity. Gu et al. reported that resveratrol (1) and its dimer showed strong DPPH free-radical-scavenging activity.Citation31) Among mono-glucoside derivatives of stilbenes, only resveratrol 3-β-glucoside (2) showed anti-oxidative activity. The DPPH free-radical-scavenging activity of resveratrol 3-β-maltoside (8) was lower than that of resveratrol 3-β-glucoside (2). These findings show that the glucosylation of resveratrol (1) at the 3-position weakened its anti-oxidative activity. On the other hand, stilbene glycosides with a glucosyl linkage at their 4′-position, i.e. resveratrol 4′-β-glucoside (3), pterostilbene 4′-β-glucoside (5), piceatannol 4′-β-glucoside (7), and resveratrol 4′-β-maltoside (9), had no anti-oxidative activity.
Next, the effects of resveratrol (1), pterostilbene (4), piceatannol (6), and their glycosides, i.e. resveratrol 3-β-glucoside (2), resveratrol 4′-β-glucoside (3), pterostilbene 4′-β-glucoside (5), piceatannol 4′-β-glucoside (7), resveratrol 3-β-maltoside (8), and resveratrol 4′-β-maltoside (9), on compound 48/80-induced histamine release from rat peritoneal mast cells were examined (Table ). Rat peritoneal mast cells released a high level of histamine (control, 52%) when stimulated with 0.35 μg/mL of compound 48/80. Piceatannol (6) effectively inhibited compound 48/80-induced histamine release from rat peritoneal mast cells: the % inhibition with 6 was 90%.
Table 3. Anti-allergic activities of compounds 1–9.
Thus, the anti-allergic action of stilbene compounds, i.e. the ability to inhibit compound 48/80-induced histamine release from rat peritoneal mast cells, was examined. Piceatannol (6) showed the highest anti-allergic activity among the stilbene compounds tested. On the other hand, piceatannol 4′-β-glucoside (7) and resveratrol 4′-β-maltoside (9) had anti-allergic activity. Resveratrol (1), pterostilbene (4), resveratrol 3-β-glucoside (2), resveratrol 4′-β-glucoside (3), pterostilbene 4′-β-glucoside (5), and resveratrol 3-β-maltoside (8) showed low inhibitory effects on histamine release. Earlier, the inhibitory activity of stilbene glycosides, the glucosyl residue of which attached at 3- or 3′-position, towards histamine release has been reported.Citation32,33) This is the first report of the inhibition of histamine release by stilbene glycoside which has glucosyl residue at its 4′-position. Ko et al. reported that piceatannol (6) strongly inhibited histamine release.Citation34) The present results also showed that piceatannol (6) inhibits histamine release.
Next, the inhibitory effects of compounds 1–9 on PDE activity were examined (Table ). As a result, pterostilbene (4) and pterostilbene 4′-β-glucoside (5) showed high PDE inhibitory activity. On the other hand, resveratrol 3-β-glucoside (2), resveratrol 4′-β-glucoside (3), piceatannol (6), piceatannol 4′-β-glucoside (7), and resveratrol 4′-β-maltoside (9) had low inhibitory activity against PDE. Recently, the inhibitory action of stilbene glycosides with glucosyl residue at their 3-position toward PDE has been reported.Citation35) This is the first description of the inhibition of PDE by the stilbene glycoside which has glucosyl residue at its 4′-position.
Table 4. Inhibitory effects of compounds 1–9 on PDE activity.
Three stilbene compounds were biotransformed to their glucoside products by cultured cells of P. americana. On the other hand, 3- and 4′-β-glucosides (2 and 3) of resveratrol were further glucosylated to 3- and 4′-β-maltosides (8 and 9) of resveratrol, 4′-β-maltoside (9) of which is a new compound, by CGTase. Resveratrol 3-β-glucoside (2) and 3-β-maltoside (8) showed DPPH free-radical-scavenging activity. Among the stilbene glycosides synthesized here, piceatannol 4′-β-glucoside (7) and resveratrol 4′-β-maltoside (9) showed inhibited histamine release from rat peritoneal mast cells more strongly than resveratrol 3-β-glucoside (2), resveratrol 4′-β-glucoside (3), pterostilbene 4′-β-glucoside (5), and resveratrol 3-β-maltoside (8). Pterostilbene 4′-β-glucoside (5) showed high PDE inhibitory activity.
Notes
Abbreviations: DPPH, 2,2-diphenyl-1-picrylhydrazyl; PDE, phosphodiesterase; FABMS, fast atom bombardment mass spectroscopy; NMR, nuclear magnetic resonance; MeOH, methanol; HMBC, heteronuclear multiple bond correlation; CGTase, cyclodextrin glucanotransferase; cAMP, cyclic adenosine monophosphate; cGMP, cyclic guanosine monophosphate; EtOAc, ethyl acetate; HPLC, high performance liquid chromatography; MS, Murashige and Skoog; fr. wt, fresh weight; DMSO, dimethyl sulfoxide; PBS, physiological buffered solution; HEPES, 4-(2-hydroxyethyl)-1-piperazineethanesulfonic acid; IBMX, 3-isobutyl-1-methylxanthine.
References
- Jubilee RS, Marlene CA, Catherine AO. J. Nutr. 2003;133:2440–2443.
- José L, Ezequiel Á, Juan AA, Reyes L, Eugenio U, Francisco O. J. Leukoc. Biol. 2004;75:1156–1165.
- Baur JA, Pearson KJ, Price NL, Jamieson HA, Lerin C, Kalra A, Prabhu VV, Allard JS, Lopez-Lluch G, Lewis K, Pistell PJ, Poosala S, Becker KG, Boss O, Gwinn D, Wang M, Ramaswamy S, Fishbein KW, Spencer RG, Lakatta EG, Le Couteur D, Shaw RJ, Navas P, Puigserver P, Ingram DK, de Cabo R, Sinclair DA. Nature. 2006;444:337–342.10.1038/nature05354
- Miller RA, Harrison DE, Astle CM, Baur JA, Boyd AR, de Cabo R, Fernandez E, Flurkey K, Javors MA, Nelson JF, Orihuela CJ, Pletcher S, Sharp ZD, Sinclair D, Starnes JW, Wilkinson JE, Nadon NL, Strong R. J. Gerontol. A Biol. Sci. Med. Sci. 2011;66A:191–201.10.1093/gerona/glq178
- Remsberg CM, Good RL, Davies NM. Pharmaceutics. 2010;2:50–60.10.3390/pharmaceutics2010050
- Wang Y, Ding L, Wang X, Zhang J, Han W, Feng L, Sun J, Jin H, Wang XJ. Am. J. Transl. Res. 2012;4:44–51.
- McCormack D, McFadden D. Oxid. Med. Cell Longev. 2013;1–15.
- Rimando AM, Kalt W, Magee JB, Dewey J, Ballington JR. J. Agric. Food. Chem. 2004;52:4713–4719.10.1021/jf040095e
- Niles RM, Cook CP, Meadows GG, Fu YM, McLaughlin JL, Rankin GO. J. Nutr. 2006;136:2542–2548.
- Son PS, Park SA, Na HK, Jue DM, Kim S, Surh YJ. Carcinogenesis. 2010;31:1442–1449.10.1093/carcin/bgq099
- Furuya T, Ushiyama M, Asada Y, Yoshikawa T. Phytochemistry. 1989;28:483–487.10.1016/0031-9422(89)80036-6
- Kamel S, Brazier M, Desmet G, Fliniaux M-A, Jacquin-Dubreuil A. Phytochemistry. 1992;31:1581–1583.10.1016/0031-9422(92)83111-B
- Morand C, Manach C, Crespy V, Remesy C. Free Radical Res. 2000;33:667–676.10.1080/10715760000301181
- Moriguchi Y, Kita M, Hasegawa S, Omura S. J. Food Agri. Environ. 2003;1:22–25.
- Shimoda K, Kondo Y, Nishida T, Hamada H, Nakajima N, Hamada H. Phytochemistry. 2006;67:2256–2261.10.1016/j.phytochem.2006.07.006
- Takenaka S, Mulyono, Sasano Y, Takahashi Y, Murakami S, Aoki K. J. Biosci. Bioeng. 2006;102:21–27.10.1263/jbb.102.21
- Yang G, Zhang Z, Bai H, Gong J, Wang Y, Li B, Li J. J. Biosci. Bioeng. 2008;105:558–561.10.1263/jbb.105.558
- Imai H, Kitagawa M, Ishihara K, Masuoka N, Shimoda K, Nakajima N, Hamada H. Biosci. Biotechnol. Biochem. 2012;76:1552–1554.10.1271/bbb.120126
- Khanduja KL, Bhardwaj A. Indian J. Biochem. Biophys.. 2003;40:416–422.
- Ozsoy N, Candoken E, Akev N. Oxid. Med. Cell Longev. 2009;2:99–106.10.4161/oxim.2.2.8493
- Akagi M, Katakuse Y, Fukuishi N, Kan T, Akagi R. Biol. Pharm. Bull. 1994;17:732–734.10.1248/bpb.17.732
- Shimoda K, Kobayashi T, Akagi M, Hamada H, Hamada H. Chem. Lett. 2008;37:876–877.10.1246/cl.2008.876
- Shimoda K, Akagi M, Hamada H. Molecules. 2009;14:3106–3114.10.3390/molecules14083106
- Medina AE. Front Neurosci. 2011;5:1–5.
- Chung JH. Adipocyte. 2012;1:256–258.10.4161/adip
- Park SJ, Ahmad F, Philp A, Baar K, Williams T, Luo H, Ke H, Rehmann H, Taussig R, Brown AL, Kim MK, Beaven MA, Burgin AB, Manganiello V, Chung JH. Cell. 2012;148:421–433.10.1016/j.cell.2012.01.017
- Chu X, Sun A, Liu R. J. Chromatogr. A. 2005;1097:33–39.10.1016/j.chroma.2005.08.008
- Joseph JA, Fisher DR, Cheng V, Rimando AM, Shukitt-Hale B. J. Agric. Food. Chem. 2008;56:10544–10551.10.1021/jf802279h
- Iwakiri T, Imai H, Hamada H, Nakayama T, Ozaki S. Nat. Prod. Commun. 2013;8:119–120.
- Park H, Kim J, Park J-H, Baek N-I, Park C-S, Lee H-S, Cha J. J. Microbiol. Biotechnol. 2012;22:1698–1704.10.4014/jmb
- Gu B, Xu Y, He S. Molecules. 2013;18:7486–7491.10.3390/molecules18077486
- Tsuruga T, Chun YT, Ebizuka Y, Sankawa U. Chem. Pharm. Bull. 1991;39:3276–3278.10.1248/cpb.39.3276
- Matsuda H, Tomohiro N, Hiraba K, Harima S, Ko S, Matsuo K, Yoshikawa M, Kubo M. Biol. Pharm. Bull. 2001;24:264–267.10.1248/bpb.24.264
- Ko YJ, Kim HH, Kim EJ, Katakura Y, Lee WS, Kim GS, Ryu CH. Int. J. Mol. Med. 2013;31:951–958.
- Atta-ur-Rahman H, Naz T, Fadimatou, Makhmoor N, Yasin FN, Fatima SF, Ngounou BL, Kimbu MI, Sondengam BL, Choudhary MI. J. Nat. Prod. 2005;68:189–193.10.1021/np040142x