Abstract
Homeodomain-leucine zipper (HD-Zip) proteins are a kind of transcriptional factors that play a vital role in plant growth and development. However, no detailed information of HD-Zip family in tomato has been reported till now. In this study, 51 HD-Zip genes (SlHZ01-51) in this family were identified and categorized into 4 classes by exon–intron and protein structure in tomato (Solanum lycopersicum) genome. The synthetical phylogenetic tree of tomato, Arabidopsis and rice HD-Zip genes were established for an insight into their evolutionary relationships and putative functions. The results showed that the contribution of segmental duplication was larger than that of tandem duplication for expansion and evolution of genes in this family of tomato. The expression profile results under abiotic stress suggested that all SlHZ I genes were responsive to cold stress. This study will provide a clue for the further investigation of functional identification and the role of tomato HD-Zip I subfamily in plant cold stress responses and developmental events.
Graphical Abstract
Tomato HD-Zip family genes were classified into four groups by their gene structures and the highly homology with Arabidopsis and rice HD-Zip subfamily proteins.
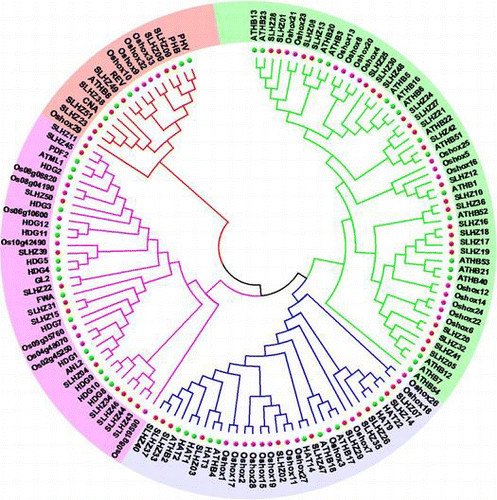
Homeobox (HB) proteins are a kind of important transcription factors that contain a highly conserved 60 or 61 amino acids of homeodomain (HD) in plant growth and development. According to HD locus, difference and homology of sequences as well as other conserved domains, HB can be classified into 14 families, they are named Homeodomain-leucine zipper (HD-Zip)(I-IV), PLINC, WOX, NDX, DDT, PHD, LD, PINTOX, WAWADEE, KNOX, and BEL, respectively.Citation1) HD-Zip family can be only found in plants, and the proteins in HD-Zip family are very abundant.Citation2) All HD-Zip proteins contain a HD domain and an adjacent leucine zipper (LZ) motif. In the proteins of this family, there are five or six special structures as one leucine (leu) residue appears after every seven amino acids. Every special structure comprises one LZ domain. Two LZ domains in two adjacent special structures dimerize a dimer motif.Citation2,3) HD domain forms a three dimensional structure of three α-helixes, and the third α-helix of this domain inserts into the target DNA’s gap for executing its activation function.
Based on the genes’ structures, specific cis-acting elements, other conserved motifs and functions, HD-Zip proteins can be divided into four classes: HD-Zip I-IV.Citation2) In Arabidopsis, most of 48 HD-Zip proteins, which have the high homology, are in class I and II. Meanwhile, they are distinctive in different subclasses. For instance, all class II HD domains contain Arg13, Lys10, Glu,18 or Asp18. And all class I HD domains contain Val3 or Ile,13 and Gln41. Class I LZ motif have Thr and Leu at al and dl position, but Leu and Thr at the corresponding position of class II.Citation4) Class I and II bind the same pseudopalindromic sequence CAAT(A/T)ATTG or CAAT(C/G)ATTG (named HDE1 and HDE2 or AH1 and AH2) specifically.Citation5) Comparing to class I, class II have extra N-Term and CPSCE (Cys, Pro, Ser, Cys, and Glu) motif that are located at the downstream of LZ motif.Citation6) Class III and IV are very different with class I and II structurally except the HD and LZ domain. Both class III and IV have START motif followed by an adjacent conserved SAD domain.Citation7,8) Class III has a special conserved MEKHLA domain in the C-terminus which is involved in light, oxygen, and redox potential sensing. The conserved nucleotide sequence, GTAAT(G/C)ATTAC, was bound by class III proteins.Citation9) Comparing to class III, Class IV lacks MEKHLA motif. And the target sequence CATT(A/T)AATG is recognized by Class IV proteins.Citation4) CTRs and NTRs also play an important role in the signaling networks of some HD proteins participated in developmental events. By analyzing motifs of 178 HD-Zip I proteins from different species, the results revealed that an AHA motif in CTR could perform the activation role at the molecular level as that in HSFs.Citation10)
HD-Zip proteins exist in all tissues and organs of higher plants. They mainly participated in plants growth, development, morphological formation, regulation of biotic and abiotic stress responses. HD-Zip I proteins were associated with abiotic stress responses, abscisic acid (ABA) responses, and embryogenesis.Citation11–14) ATHB-13 participated in sucrose-responsive development,Citation15) and ATHB-20 is involved in vascular patterning and integration of light and internal ABA signaling during seed germination.Citation16,17) ATHB-1 is the first discovered HD-Zip I gene in Arabidopsis, which is involved in cotyledon and leaf development.Citation1) ATHB-5, ATHB-6, ATHB-7, ATHB-12 can be up- or down-regulated by drought stress or external ABA. ATHB-6 was regulated by osmotic stress; ATHB-7 was induced by salt and osmotic stress. These results suggested that ATHB-6 and ATHB-7 play a role in dehydration response reaction.Citation18–20) ATHB-16 not only participated in blue-light signal pathway, but also negatively regulated leaves development and flowering time in Arabidopsis.Citation13) CpHB-7 was isolated from Craterostigma plantagineum, and this protein was associated with the early development of plant organs and also regulated by ABA as a negative regulator.Citation21)
HD-Zip II subfamily mainly responded to light stress and participated in plant organs development and shading.Citation22–24) The expression of ATHB2/HAT4 increased under white light and also can be induced by lower red/far-red light in Arabidopsis so as to lead to plant shade avoidance response. Plants that over-expressing ATHB2/HAT4 genes showed leaf size reduction and inhibition of cell differentiation under white light.Citation24) JAIBA is a HD-Zip II protein of Arabidopsis, which interacted with some transcription factors to regulate meristematic activity, fruit development, and floral meristem (FM) determinacy.Citation22) Besides responding to shade, ATHB-4 and HAT-3 also play a critical role in establishing the dorsoventral axis of cotyledons and developing leaves.Citation23)
HD-Zip III class proteins were extensively investigated in recent years. It is mainly involved in the differentiation of apical meristem, vascular tissue formation, polar transport of auxin, the development of embryonic and near-axis region lateral organ, etc.Citation25–27) ATHB8 gene mainly expressed in vascular tissue formation parts. It functioned as a differential promoter of translation factor. Xylem could be over-accumulated if ATHB8 gene is overexpressed.Citation27) ATH15 gene expressed in lateral meristem, FM, and myelocyte, especially much expressed in fibrovascular tissue. ATHB8, ATHB15, and PHB genes expressed in vascular cambium and immature secondary xylem of poplar, which meant that they regulated the development progress of secondary vascular tissue.Citation28,29)
HD-Zip IV participated in the development of roof growth and cell fates, and also regulated the accumulation of anthocyanin and trichome formations. ATML1 and PDF2 genes mainly expressed in the developing embryo dermatogens and the outer cells of shoot apical meristem (SAM). The atml1/pdf2 double mutant never forms an organized protodermal layer in the apical part of proembryo.Citation30–33) ANL2 plays a role in the accumulation of anthocyanin and cellular organization of the primary root.Citation32) Wo is a GL2-like HD-Zip IV protein of tomato, which is important to trichome formation. Wo gene interacted with cell cycle gene SLCycB2 to promote the transit from G2 to M. Wo homozygote caused tomato embryo lethal.Citation33)
Tomato is an important agricultural and economic crop, also a significant model plant in investigating evolution, genetics, and other basic biological research. After completion of tomato whole genomic sequencing,Citation34) classification and function of large number of genes will be predicted and identified.
HD-Zip is an important gene family in plant development and growth, and it has been widely investigated in Arabidopsis, rice, maize, etc. A few HD-Zip genes of tomato have been reported, but the whole family has not been systematically analyzed in tomato yet. In this study, 51 putative HD-Zip genes of tomato were found, analyzed, and classified. And we found some cis-acting elements related to cold tolerance in HD-Zip I gene promoters. And these results were also confirmed using semi-quantitative PCR. This study will provide a useful information and theoretical basis for elucidating the roles of HD-Zip genes in tomato growth and development.
Materials and methods
Database search and HD-Zip gene identification
Arabidopsis HD-Zip proteins’ sequences were obtained from the Arabidopsis Information Resource (TAIR, http://www.arabidopsis.org). Rice sequences were downloaded from Phytozome (http://www.phytozome.net, release 9.1) To identify putative HD-Zip genes in Solanum lycopersicum, E-value cutoff was set to 1e−10, a BLASTP searching was performed against S. lycopersicum protein released by SGN (http://solgenomics.net, release v2.31) using HD-Zip protein sequences in Arabidopsis and rice as queries. All candidate sequences of S. lycopersicum were further confirmed by analyzing the conserved HD and LZ domains using software SMART (http://smart.embl-heidelberg.de/) and Pfam (http://pfam.janelia.org). The information about proteins, genome, cDNAs, exon–intron, and chromosome locations of S. lycopersicum were obtained from the SGN sequence database. Molecular weight and theoretical pI of HD-Zip amino acids were calculated by ProtParam online (http://expasy.org/tools/protparam.html).Citation35) Subcellular localizations of these family genes were predicted using CELLO v2.5 serve online (http://cello.life.nctu.edu.tw/).Citation36)
Analysis of tomato HD-Zip genes phylogenetic, conserved motifs, and exon–intron structures
The HD-Zip proteins of tomato, Arabidopsis, and rice were aligned using software ClustalX1.83. Two unrooted trees were generated by the neighbor-joining (NJ) method and maximum parisomony (MP) method using software MEGA 5.0 and bootstrap analysis. Thousand replicates were performed with the pairwise deletion option.Citation37) An unrooted tree of tomato HD-Zip proteins was constructed by NJ method using software MEGA 5.0. Motif 4.6.1 online (http://meme.nbcr.net/meme/intro.html) was used to display the conserved motifs of HD-Zip genes.Citation38) The optimized parameters were set as following: the optimal motif widen was ≥3 and ≤200; the maximum number of motifs was 20. The exon–intron structure analysis of tomato HD-Zip genes were displayed by inputting sequences into GSDS (http://gsds.cbi.pku.edu.cn).Citation39)
Chromosomal location and promoter analysis
The S. lycopersicum HD-Zip genomic sequences from SGN were downloaded to determine the positions of HD-Zip genes on chromosomes. About 2000 bp of genomic DNA sequences at the upstream of initiation codon of candidate HD-Zip I genes were obtained from SGN for promoter cis-elements analysis. The cis-elements of promoter domains were analyzed on PLACE website (http://www.dna.affrc.go.jp/PLACE).Citation40)
Plant materials and cold stress treatment
S. lycopersicum cv. Micro-Tom was grown in a greenhouse at the horticultural experimental station of the Northeast Agricultural University. Three-week-old seedlings (four-leaf stage) of Micro-Tom were used to examine the expression patterns of HD-Zip I genes under cold stress. For cold stress experiment, three-week-old tomato seedlings were grown in growth chamber at 5 °C (Conviron, Canada). The upper layers leaves were sampled at 0, 1, 3, 6, 9, 12 h after cold treatment in daytime, and three biological replicates of every treatment were performed.
RNA extraction and semi-quantitative RT-PCR analysis
Total RNA was extracted using Trizol reagent (Invitrogen) according to the manufacturer’s instructions. The extracted total RNA was treated using DNase I (Fermentas) for 20 min to remove possible contaminated genomic DNA. RNA integrity was verified by 1.2% agar gel electrophoresis. A first strand cDNA was synthesized using first strand cDNA synthesis kit (Fermentas). The special PCR primers of each SlHZ I genes (Table_S4) were designed using Prime Premier 5.0 software, and amplicon size 89 bp-577 bp, melting temperature from 50–60 °C, primers’ sizes between 18–24 bp. The GAPDH gene primers were designed according to GenBank Accession No. NM_001247874.1. Every PCR reaction system contained cDNA 0.5 μL, forward primer (10 mM) 1 μL, reverse primer (10 mM) 1 μL, 10 × EasyTaq buffer 2 μL, dNTP (2 mM) 2 μL, EasyTaq DNA polymerase (Transgene) 0.5 μL, and add ddH2O to total volume of 25 μL. The PCR amplification was performed for 30–40 cycles (30 s at 95 °C, 30 s at 60–65 °C, 30 s at 72 °C) followed by 10 min at 72 °C. The PCR results were checked by 2% agar gel electrophoresis and analyzed by AlphaEaseFC image analysis software. Triplicates of PCR amplifications for every cDNA sample were conducted. Statistical analyses of RT-PCR data were performed using SPSS (Statistical Package for the Social Sciences version) 16.0. Changes of relative expression levels were calculated as Mean ± SEM and evaluated by LSD (Least Significant Difference). Differences were considered statistically significant if p < 0.05. The frequency histogram was created using Origin7.5.
Results
Identification of HD-Zip gene family
By blasting and searching the SGN website (http://solgenomics.net, release v2.31), 51 HD-Zip genes (named SlHZ01 to SlHZ51) were identified in tomato genome. Tomato genome contains more HD-Zip genes than that of Arabidopsis (48) and rice (48). The detailed representative HD-Zip protein sequences of HD-Zip members in tomato are showed in supplemental Text_S1, which also provided the identified Arabidopsis and rice HD-Zip protein sequences.
The length of 51 HD-Zip proteins in tomato varied from 175 to 852aa with the average of about 500aa. The molecular weight ranged from 20.26 to 93.51 kD and the theoretical isoelectric point ranged from 4.57 to 9.54. As show in Table_S1, HD-Zip genes were mainly located in plasma membrane and nucleolus. These location results of HD-Zip genes need to be experimentally confirmed.
Phylogenetic classification and structural analysis of SlHZ genes
After identifying the whole sequences of all tomato HD-Zip proteins, an unrooted phylogenetic tree was generated (Fig. (A)). All tomato HD-Zip proteins were classified into four groups (class I, II, III, IV) by the phylogenetic tree using the well-supported bootstraps. Class I with 22 members was the biggest group, the second group was class II with 11 members, and class III and class IV had 12 and 6 genes, respectively. Fifty one tomato HD-Zip genes formed 17 gene pairs and most of them were supported by high bootstraps (>95%) except SlHZ34/46, SlHZ02/47, SlHZ15/31, SlHZ05/41. The exon–intron structure analysis using GSDS online software also confirmed the construction results of phylogenetic tree (Table_S1). The introns’ numbers of four classes were regular (class I: 0–3 introns; class II: 2–3 introns; class III: 17–19 introns; class IV: 7–10 introns). The structures of the genes in class I and II were much simpler than those in class III and IV. The results indicated a tight correlation between phylogeny and exon–intron structure. The genes in same cluster had a similar structure. The coding regions of most of genes were disrupted by one or more introns except SlHZ10, SlHZ36 without introns. The results indicated that the genes might gain or lost exons or introns in the process of evolution.
Fig. 1. Classification and Conserved Motif of the HD-Zip Gene Superfamily in Tomato.
Note: (A) Phylogenetic analysis of tomato SlHZ proteins. The tree was constructed from a complete alignment of 51 HD-Zip proteins by the NJ method with bootstrapping analysis (1000 replicates). Bootstrapping values are indicated as percentages (>50%) along the branches. The resulting groups are classed into four types by being marked with I, II, III, and IV, and (B) distribution of conserved motifs in tomato identified by MEME. Twenty conserved motifs are shaded in different colors. The results showed the special function names by being identified in Pfam and START.
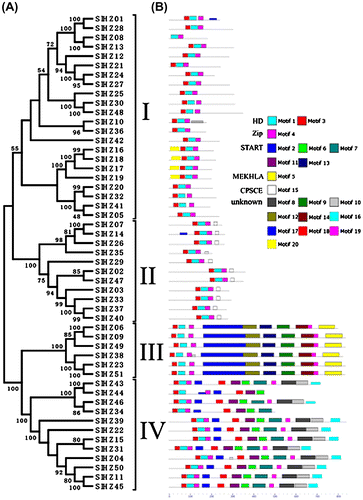
We also reconstructed a combined phylogenetic tree by aligning the full length sequences of tomato, Arabidopsis, and rice HD-Zip proteins (Text_S1) to reveal the phylogenetic relationships in different species. The phylogenetic tree was obviously divided into four groups (Fig. ). According to the previous results in Arabidopsis and the nomenclature previously proposed by Henriksson et al.Citation41) the HD-Zip I genes were divided into 10 monophyletic subclasses(namely α, β1, β2, γ, δ, ε, φ1, φ2, ζ, η). All tomato and rice HD-Zip I genes could be classified together with Arabidopsis counterparts except ζ, η clades. ζ clade only has four rice members. Oshox4, -20, -8, and -13 were supported by high bootstrap (>88%). The results were consistent with that of Agalou.Citation42) η clade had three tomato members, SlHZ21 (VAHOX1), -24, -27, which shared the same new branch with high bootstrap support (>93%). The original β clade was also divided into β1 and β2 clades as reported in maize and rice.Citation42,43) β2, φ1, ε clades were grouped by tomato and Arabidopsis genes. SlHZ12 in β1 clade, SlHZ08, -13 and -28,-01 in α clade, SlHZ16, -17, -18, -19 in δ clade, SlHZ20, -32, -41, -05 in γ clade were clustered with Arabidopsis and rice in their clades. Henriksson et al. reported that ATHB52 and -54 were in φ clade.Citation41) However, in this study, ATHB54 did not had any counterparts of two other species or did not belong to any clade as Agalou reported.Citation42) ATHB54 is a atypical HD-Zip I protein with an extremely long CTR (325aa) which a RNA recognition motif can be identified in.Citation10)
Fig. 2. The Phylogenetic Analysis of HD-Zip Genes in Arabidopsis, Rice, and Tomato.
Note: The tree was constructed from a complete alignment of Arabidopsis, rice, and tomato HD-Zip proteins by the NJ method with bootstrapping analysis (1000 replicates). Bootstrapping values are indicated as percentages (>50%) along the branches. The resulting groups are shown in different colors.
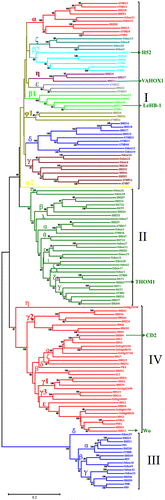
In class II, HD-Zip genes of three species were divided into several subclasses as reported in Arabidopsis.Citation44) The new clade ε with Oshox27, -11, -19, -15, and SlHZ02 had no Arabidopsis counterparts, which was consistent with classification of maize HD-Zip family.Citation43) The Oshox26, -18 composed a single η clade; Oshox28, -2 were in ι clade; Oshox7, Oshox17, -1 belongs to λ, ζ, and κ clade, respectively; These five clades had just only rice HD-Zip genes. Clade α was composed of the genes from three species. The other mainly clades were grouped by the genes from tomato and Arabidopsis with bootstrap support >72%.
Class III had the fewest members, only six tomato HD-Zip genes. But it still had two sister pairs. SlHZ23/ SlHZ51 showed close relationship with CAN/ATHB8 in α clade and SlHZ06/SlHZ09, Oshox32/Oshox33 were close to PHB/PHV sister pair in γ clade. The results were consisted with the previous studies of HD-Zip III in poplar and maize.Citation11,43)Footnote1 Oshox29 did not belong to any clades. The phylogenetic clades of class IV were more complicated than that of class III as showed in poplar,Citation11) though they had the similar conserved domains HD, Zip, and START. α, γ1,ε clades in class IV were generated by the genes of tomato, rice, and Arabidopsis, and the tomato genes are close to that of Arabidopsis in their clades. Clades γ2 and β only contained the genes of tomato and Arabidopsis. SlHZ15/SlHZ31 sister pair belonged to a single ζ clade. The whole synthetic evolutionary tree showed that most clades are clustered by tomato, Arabidopsis, and rice, the tomato HD-Zip genes only tended to group with eudicot Arabidopsis, such as α, β1, γ, δ in class I, α in class II, β clade in class III, α, γ1 clades in class IV. A few clades were clustered by tomato and Arabidopsis, such as β2, ε, φ1 in class I, β, θ, δ, γ clades in class II, α clade in class III, γ2, β clades in class IV. There were also two singletons, clade η in class I and ζ clade in class IV. They did not have any other counterparts of Arabidopsis or rice members. The phylogenetic tree also revealed that 14 tomato HD-Zip pairs with high bootstrap support were assigned as paralogous pairs for their sequence similarities. These 14 pairs of proteins occupied more than half of all proteins of tomato HD-Zip family, and the sequence similarities ranged from 43 to 92% (Table_S2). Six tomato HD-Zip genes have been reported. SlHZ25 (H52) was involved in cellular protection by limiting spread of programmed cell death in plants, which showed a close relationship with ATHB5, -6, and -16 of class I in clade β2.Citation19,45) VAHOX1 is one of tomato HD-Zip I protein in η clade protein whose expression increased in sepals after cold treatment, its regulation induced by cool temperatures was independent of ethylene.Citation46) LeHB-1 of tomato HD-Zip I gene in β2 clade is not only involved in the control of ripening but also plays a critical role in floral organogenesis.Citation47) SlHZ03 (THOM1) was grouped in δ clade of class II with ATHB4 and HAT3, they all played an important role in the development of SAM. And THOM1 expression levels decreased with increasing age of the respective tissue.Citation48,49) SlHZ11 (Wo, woolly) and SlHZ45 were clustered with PDF2, HDG2, and ATML1 in the same clade ε, which suggested that these genes of this clade might played an important role in epidermal cell fate.Citation33,50–53) CD2 gene is another HD-Zip IV member of tomato in α clade, which is associated with cutin deficiency in tomato fruit and preferentially expressed in epidermal cells of tomato stems. CD2 is a homolog of Arabidopsis ANTHOCYANINLESS2 (ANL2).Citation54)
The conserved motifs of tomato HD-Zip genes
The 20 conserved motifs of putative tomato HD-Zip proteins were analyzed on the MEME web server. Based on the distribution of the 20 predicted motifs, the tomato HD-Zip genes were also categorized into four classes which were consistent with the phylogenetic analysis (Fig. (B)). We found motifs 1 and 3 in all of HD-Zip genes encoded the HD domain except SlHZ08 without motif 3. Motif 4 encoded Zip domain in all the genes. We also found that START domains encoded motif 2, 6, 7, 11, 18 of all class III and IV, but not existed in class I and II. Representative MEKHLA domains, motif 5, were only in class III. CPSCE domain, motif 15, existed in class II. The other 10 motifs’ functions were not identified by Pfam and SMART analysis. But they still distributed regularly, as motif 8,10,16,17,18,19 were in class IV and motif 9,12,14,19 were in class III, and motif 20 was in SlHZ16,-17,-18, and -19 of class I. The results further supported the category of tomato HD-Zip family. Arce et al. have described six motifs associated with the CTRs of HD-Zip I subfamily in signal transduction pathways of different species, which were always ignored in the past.Citation10) But in this study, the 10 non-identified motifs were not found to match Arce’s results. Therefore, we concluded that the 10 non-identified motifs could have special functions, and this need to be further verified. All the putative motifs’ sequences were listed in Table .
Table 1. Major MEME motif sequences in tomato HD-Zip proteins.
Chromosomal location and gene duplication
The 51 SlHZ genes were distributed on 12 tomato chromosomes (Fig. ). Most of genes, nine SlHZ genes, were on chromosome II and III. Five genes were on chromosome I and VIII. Moreover, there were four genes on chromosome V, three genes on chromosome IV, VI, VII, IX, X, two genes on chromosome XI, XII, respectively. High densities of SlHZ genes were found at the top of chromosome III and V, the bottom of chromosome I, II, III, and VIII. The SlHZ genes on chromosome IV, XI, X were located at both ends of chromosomes. Overall, the gene duplication patterns including segmental duplication were primary, and tandem duplication was secondary (Fig. ). The segmental duplications events were found based on the phylogenetic analysis and chromosomal distribution of the 14 gene pairs (SlHZ08/13, SHLHZ24/27, SlHZ30/48, SlHZ10/36, SlHZ20/32, SlHZ16/18, SlHZ07/14, SlHZ43/44, SlHZ37/40, SlHZ06/09, SlHZ23/51, SlHZ15/31, SlHZ11/45, SlHZ46/34). Two pairs (SlHZ08/13, SlHZ07/14) were located on chromosome II, and the gene pair SlHZ37/40 was segmental duplication at chromosome VIII, SlHZ16/18 and SlHZ43/44 were located at chromosome III and IX, respectively. Three tandem duplications were found. One cluster contained genes SlHZ16, 17, 18, 19 at chromosome II, and another cluster contained genes SlHZ22 and 23 at chromosome III, the third tandem duplication were SlHZ43 and 44 at chromosome IX that were homologous genes within the range of 100 kb distance.
Fig. 3. Chromosomal Location of Tomato HD-Zip Genes on all 12 Chromosomes.
Note: Chromosomal mapping was based on the physical position (Mb) in 12 tomato chromosomes. The chromosome number is indicated at the top of each chromosome. Chromosomal positions of the tomato SlHZ genes are indicated by gene name (assigned in Text_S1). Scale represents a 5 Mb chromosomal distance. Three tandem duplications are clustered together within 100 kb distance and marked with orange color. The dash blue lines connect the other corresponding 12 pairs of paralogous genes in duplicated blocks were segmental duplication.
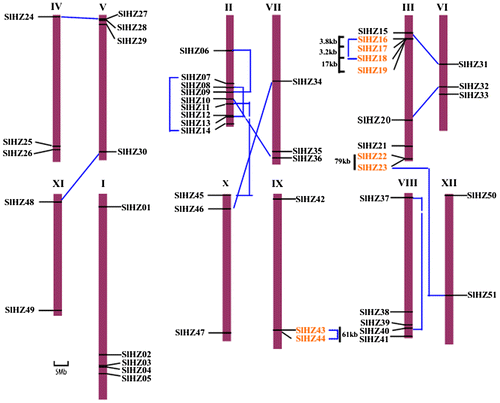
Promoter analysis of the HD-Zip gene I stress responsive cis-elements
Promoter is an important indicator of gene expression. So we investigated 2000 bp upstream sequences from the translation start codon in SlHZ I gene. The sequences were detected by searching the PLACE (http://www.dna.affrc.go.jp/PLACE). Four types of cis-elements, which were related to the ABA stress responsive element-ABRE,Citation55–57) low temperature responsive element-LTRE,Citation58–60) drought stress responsive element-DRE,Citation55,61,62) and salinity stress responsive elementCitation63) were found (Table_S3). The results showed all the SlHZ I genes’ promoters contained these four cis-acting elements except SlHZ16 without salinity stress responsive element in its promoter. The six sister pairs of HD-Zip I were not same in the cis-acting element number and distribution by comparing the promoters’ sequences. These results implied that these genes’ functions could be different, but they might be under a similar regulatory pathway of plants’ growth and development.
Expression patterns of SlHZ I genes under cold stress
HD-Zip I genes have been reported to play an important role in abiotic and biotic stress.Citation11,42,43) Expression patterns of SlHZ I genes were checked in leaves of three-week-old tomato cv. Micro-Tom under cold stress using semi-quantitative RT-PCR. Cold stress was the important environmental factor affecting tomato development. The expression levels of all the 22 SlHZ I genes were significantly different comparing to the untreated plant (Fig. (A) and (B)). Among the 22 SlHZ I genes, the expressions of 13 genes (SlHZ01, SlHZ05, SlHZ08, SlHZ13, SlHZ16, SlHZ19, SlHZ20, SlHZ21, SlHZ25, SlHZ27, SlHZ30, SlHZ36, SlHZ41) were up-regulated under cold stress. Expression of SlHZ19,-16, -27, -30, -08, and -05 increased with time. SlHZ13 was also up-regulated with time except dropping at 12 h point. From 6 to 9 h expression of SlHZ01 increased, while expression of SlHZ20 and -25 only increased at 9 and 3 h, respectively. Almost all class I genes changed gradually, but SlHZ05 and -16 increased significantly comparing to the low expression at normal 0 h point. The other nine SlHZ I genes were down-regulated under cold stress treatment. Almost all genes’ expression declined at first and had a rebound to a peak, then dropped gradually. Only SlHZ17 gene decreased gradually with time. SlHZ21 (VAHOX1) transcription level increased in cold-treated sepal,Citation46) also in cold-treated tomato leaves.
Expression patterns of six paralogous pairs of SlHZ I genes were also investigated. The results showed a sister pair had the similar expression patterns under cold stress as SlHZ08/SlHZ13 pair, both were up-regulated. The other five pairs have the similar expression trends and peak points. For instance, both SHLZ20/32 and SlHZ24/27 increased gradually at early stages, and then reached to a peak at 9 h point, declined at 12 h finally. SlHZ10/36 and SlHZ30/48 had a highest express level at 3 h. The pair SlHZ16 and -18 did not had the same peak point, but the two members had the similar express trends as low-high-low. These expression patterns suggested a divergence in the biological functions of SlHZ genes during plants’ growth and development.
Discussion
SlHZ gene family in tomato genome
In this study, 51 HD-Zip genes in tomato genome were identified and characterized from the current version of SGN database v2.31 (http://solgenomics.net/). All the SlHZ genes contained the conversed domain HD and Zip of HD-Zip protein and classified into four classes (Fig. (A): I, II, III, IV). The number of HD-Zip members in tomato was comparable to the Arabidopsis (48)Citation2) and rice (48),Citation42) fewer than that of maize (55)Citation43) and poplar (63).Citation11) In this study, BlastP program was used to identify the SlHZ genes using E-value cutoff set to 1e−10, and the genome database we used was the most current and updated sequence assemblies. Therefore, it was likely that not all SlHZ genes had been identified. Like other HD-Zip genes in Arabidopsis and rice,Citation64) the tomato HD-Zip genes also showed regular number distribution of exon–intron, subcellular localization results were also regular, but it still needs to be further verified by specific experiments. Genes’ structure in class III and IV were much more complex than that in class I and II. The similar structures of HD-Zip proteins in three species indicated their evolutionary conservation. The further analysis of SlHZ proteins motif, the regular distribution of conserved motif in relevant class also supported the results of phylogenetic analysis (Fig. (A)).
The SlHZ duplication events
SlHZ gene family expansion was mainly showed by segmental duplication and tandem duplication. Tandem duplication was two or more genes located on the same chromosome within the range of 100 kb distance, while the segmental duplication means the homologous genes duplicated on different chromosomomes.Citation65,66) In this study, the results showed the SlHZ genes were distributed at all the 12 chromosomes. SlHZ genes were distributed with high density at chromosome II and III, each contained nine genes. The results suggested that they might be generated from tandem duplications. For instance, SlHZ16/17/18/19 were tandem duplication event at chromosome III, they belonged to SlHZ class I. SlHZ43/44 sister pair of class SlHZ IV at chromosome IX were found to be involved in tandem duplication. SlHZ22 and SlHZ23 at chromosome III were categorized into IV and III, they were tandem duplication. The results of tandem duplication genes from different classes suggested the tandem duplication genes might generate new functions. Fourteen sister pairs of close paralogs were involved in segmental duplication event by scattering throughout the genome, while chromosome II conferred the highest number of segmental duplication events with SlHZ06/09, SlHZ07/14, and SlHZ08/13 sister pairs. The other segmental duplication events were on different chromosomes as SlHZ10 (chromosome II) and SlHZ36 (chromosome VII), SlHZ11 (chromosome II), and SlHZ45 (chromosome X). In contrast, chromosome I had not any duplication events. These 14 sister pairs of genes involved in segmental duplications represented approximately 55% of SlHZ genes (51). And the other eight genes were involved in tandem duplications which accounted for 15.6%. The results showed the segmental duplication of tomato HD-Zip genes, not tandem duplication, was the main gene expansion pattern.
Evolution of the gene family might be complicated and influenced by both gene duplication and gene loss. It was reported that tomato was likely to be a paleopolyploid,Citation67) in which a large scale of genome duplication occurred approximately 50–52 million years ago.Citation68) In this study, most of SlHZ genes in tomato occurred in segmental duplication (55%), and segmental duplications were also identified in Arabidopsis (75%),Citation41,44,69,70) rice (54%)Citation71), and maize (62%).Citation43) Therefore, the segmental duplication was regarded as the major mechanism for expansion of HD-Zip family, although segmental and tandem duplication both occurred in the expansion of the four species. The results suggested they might experience similar evolutionary mechanisms.
Phylogenetic and evolutionary analysis of the HD-Zip gene family in tomato, Arabidopsis, and rice
Based on the whole protein sequences, a combined phylogenetic tree including the genes of three species (tomato, Arabidopsis, and rice) was established to clarify the relationships among the HD-Zip genes and infer the evolutionary history of this gene family. Two combined phylogenetic trees were constructed using the NJ (Fig. ) and MP (Fig. S1) methods by software MEGA5.0 both the two phylogenetic trees showed the similar topology results. We further analyzed the NJ tree to study the evolutionary relationships of the three species therefore.
In phylogenetic tree, tomato, Arabidopsis, and rice HD-Zip genes were divided into four classes (Fig. ). The genes of these three species were distributed on all the classes, which indicated that the basic features of HD-Zip gene family were formed earlier than the divergence period of monocots and dicots. In addition, all the four classes were divided into many subclasses with high bootstraps to analyze the intraspecific and interspecific relationships. The results suggested their similar original features. Fourteen pairs of paralogous genes were identified (Fig. ), which was important to reveal the evolutionary mechanisms for more recent duplication.
Orthologs and paralogs are the key concepts of evolutionary genomics, they are two fundamentally different types of homologous genes that evolved by vertical descent from a single ancestral gene and duplication, respectively.Citation72) Orthologs are defined as genes in different species that are similar in their nucleotide sequences, suggesting that they originated from a common ancestral gene. Orthologs often retain the same functions in the course of evolution, thus functions may be found to be lost or gained through comparing a pair of orthologs. Paralogs are genes produced via gene duplication within a genome. Paralogs typically evolve new functions or eventually become pseudogenes.Citation73) Based on evolutionary origins of orthologous and paralogous genes, comparison of their sequences can facilitates a better understanding of the physiological roles of individual genes. In this phylogenetic tree, we got 14 tomato paralogous pairs (e.g. SlHZ06/SlHZ09, SlHZ30/SlHZ48), 14 rice paralogous pairs (e.g. Oshox26/Oshox18, Oshox10/Oshox9), and 13 Arabidopsis paralogous pairs (e.g. ATHB12/ATHB23, HAT22/HAT9), seven orthologous pairs (SlHZ42/ATHB51, SlHZ12/ATHB1, SlHZ29/ATHB17, SlHZ47/HAT14, SlHZ04/ANL2, SlHZ22/GL2, SlHZ49/REV). All the orthologs were SlHZ/ATHB pairs; the results indicated the tomato HD-Zip genes were more closely allied with Arabidopsis homologous genes than that of rice. The results were consistent with the evolutionary relationships among tomato, Arabidopsis, and rice. A large scale of segmental duplication events occurred in the evolutionary process of tomato when tomato/Arabidopsis split around 90 Mya.Citation74) And the data of HD-Zip genes in Arabidopsis indicated the family members expansion occurred approximately between 20 and 60 Mya.Citation75,76) So the phylogenetic results also illustrated that tomato and Arabidopsis had the common ancestor. But more paralogous pairs suggested the SlHZ genes duplication events occurred after tomato/Arabidopsis divergence and the HD-Zip gene family is a conserved evolving family in plant genomes.
Expression patterns of SlHZ I genes in cold stress
It has been reported the HD-Zip families been regulated by many kinds of biotic or abiotic stresses as dehydration, salinity, and hormone.Citation11,42,43) However, there is few investigation about HD-Zip gene under cold stress, especially in tomato. So we investigated the expression patterns of tomato HD-Zip I genes under cold stress. The results showed that all the 22 genes have been induced by cold stress. The 59% (13) of 22 SlHZ I genes were up-regulated significantly. 36% (8) genes were down-regulated firstly, and then they still rebounded to a high expression peak though they expressed lower than the 0 h control. The results suggested that the low expression level of genes also provided the defensive and tolerant protection to the tomato plant. The similar expression patterns of six duplicated gene pairs were also found, but their expression levels were different. Numbers and positions of cold stress-related cis-acting elements of the six gene pairs were distributed differentially in their promoters (Fig. ). The results indicated that the duplicated genes with redundant functions might respond to cold stress. They could diverge with the evolution of each gene after duplication. Only expression of SlHZ17 gene decreased gradually without any rebound under cold stress treatment (Fig. ). The results implied that SlHZ17 was neofunctionaliztion or subfunctionalization of the evolutionary reason. The results suggested that SlHZ genes played an important role in stress defense and tolerance. These candidate genes would provide the basic information for further investigate tomatoes’ response to different stress conditions.
Fig. 4. Distribution of Major Stress-related cis-elements in the Promoter Sequences of the 22 SlHZ I Genes.
Note: About 2000 bp upstream sequences of SlHZ I genes from the start codon were investigated and analyzed by searching PLACE. Putative ABRE, LTRE, DRE, and salt stress-related cis-elements are represented by different colors as indicated and distributed on the black lines.
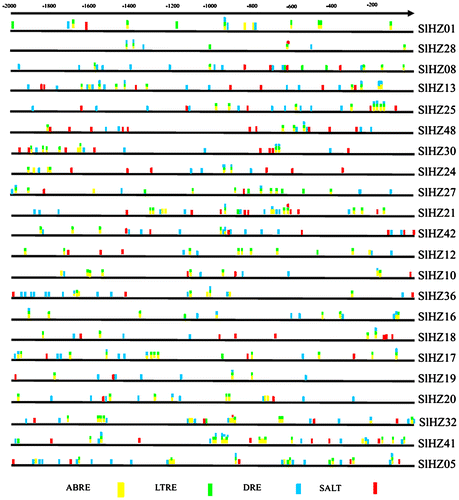
Fig. 5. Expression Patterns of the 22 SlHZ I Genes under Cold Stress.
Note: Semi-RT PCR was performed using primers specific for each SlHZ I gene. (A) PCR products were run on 2% agarose gels. GAPDH primers generated a 120 bp products were used as the inner standard for each gene. Ck, 0 h plant, leaves sampled at 1, 3, 6, 9, 12 h after treatment, and (B) relative expression of SlHZ I genes were analyzed RT-PCR products agarose gels and normalized by GAPDH expression. The Y axis is the scale of the relative expression level. The X axis is the time course of cold stress treatment. The statistical bars mean the relative expressions of SLHZ genes in different treatments. *p < 0.05, **p < 0.01, compare to 0 h.
Supplemental material
The supplemental material for this paper is available at http://dx.doi.org/10.1080/09168451.2014.923292
bbb-130944-File015_1_.pdf
Download PDF (186.7 KB)bbb-130944-File014_1_.pdf
Download PDF (44.5 KB)bbb-130944-File013_1_.pdf
Download PDF (28.6 KB)bbb-130944-File012_1_.pdf
Download PDF (54.6 KB)bbb-130944-File011_1_.pdf
Download PDF (64.4 KB)bbb-130944-File010_1_.pdf
Download PDF (119.1 KB)Funding
This work was supported by grants from the Trans-Century Training Program Foundation for the Talents by Heilongjiang Provincial Education Department [grant number 1251-NCET-004] to A.X. Wang, the Returned Oversea Scholar Foundation by Heilongjiang Provincial Education Department; and the National Science Foundation of China [grant number 31301780] to X.L. Chen.
Notes
Abbreviations: HB, homeobox protein; HD, homeodomain; HD-Zip, homeodomain-leucine zipper; PLINC, plant zinc finger; DDT, DNA binding homeobox and Different Transcription factors; WOX, wuschel-related homeobox; NDX, nodulin homeobox genes; KNOX, KNOTTED1-like homeobox; BEL, BEL1-like homeobox genes; PHD, Plant Homeo-Domain; LD, Luminidependens, a homeobox gene; PINTOX, the Plant Interactor Homeobox rice gene GF14c-int; SAWADEE, a plant-specific domain which was found to bind specifically to histone H3 containing H3K9 methylation; LZ, leucine zipper motif; START, STerodiogenic Acute Regulatory protein-related lipid Transfer; SAD, START-adjacent domain; MEKHLA, Mekhla, or various other spellings, is a goddess of lightning, water, and rain domain; CTRs, carboxy-germinal regions; NTRs, amino terminal regions; AHA, aromatic, large hydrophobic, acidic context; HSFs, heat stress transcription factors; TFs, Transcription factors; CD2, cutin deficient 2; THOM1,tomato homeobox gene 1.
1. In this study, we used Arabidopsis and rice as the representative of dicotyledon and monocotyledon to construct the phylogenetic trees, therefore we didn’t add the HD-Zip protein sequences of poplar and maize in the trees. But we still used the naming and classification styles, and the greek alphabet letters used in this paper did not mean the same clades in Arabidopsis, rice, poplar, and maize.
References
- Mukherjee K, Brocchieri L, Burglin TR. A comprehensive classification and evolutionary analysis of plant homeobox genes. Mol. Biol. Evol. 2009;26:2775–2794.10.1093/molbev/msp201
- Ariel FD, Manavella PA, Dezar CA, Chan RL. The true story of the HD-Zip family. Trends Plant Sci. 2007;12:419–426.10.1016/j.tplants.2007.08.003
- Ruberti I, Sessa G, Lucchetti S, Morelli G. A novel class of plant proteins containing a homeodomain with a closely linked leucine zipper motif. EMBO J. 1991;10:1787–1791.
- Tron AE, Bertoncini CW, Palena CM, Chan RL, Gonzalez DH. Combinatorial interactions of two amino acids with a single base pair define target site specificity in plant dimeric homeodomain proteins. Nucleic Acids Res. 2001;29:4866–4872.10.1093/nar/29.23.4866
- Sessa G, Morelli G, Ruberti I. The Athb-1 and -2 HD-Zip domains homodimerize forming complexes of different DNA binding specificities. EMBO J. 1993;12:3507–3517.
- Chan RL, Gago GM, Palena CM, Gonzalez DH. Homeoboxes in plant development. Biochim. Biophys. Acta. 1998;1442:1–19.10.1016/S0167-4781(98)00119-5
- Schrick K, Nguyen D, Karlowski WM, Mayer KF. START lipid/sterol-binding domains are amplified in plants and are predominantly associated with homeodomain transcription factors. Genome Biol. 2004;5:R41.10.1186/gb-2004-5-6-r41
- Ponting CP, Aravind L. START: a lipid-binding domain in StAR, HD-ZIP and signalling proteins. Trends Biochem. Sci. 1999;24:130–132.10.1016/S0968-0004(99)01362-6
- Mukherjee K, Bürglin TR. MEKHLA, a novel domain with similarity to PAS domains, is fused to plant homeodomain-leucine zipper III proteins. Plant Physiol. 2006;140:1142–1150.10.1104/pp.105.073833
- Arce AL, Raineri J, Capella M, Cabello JV, Chan RL. Uncharacterized conserved motifs outside the HD-Zip domain in HD-Zip subfamily I transcription factors; a potential source of functional diversity. BMC Plant Biol. 2011;11:42.10.1186/1471-2229-11-42
- Hu R, Chi X, Chai G, Kong Y, He G, Wang X, Shi D, Zhang D, Zhou G. Genome-wide identification, evolutionary expansion, and expression profile of homeodomain-leucine zipper gene family in poplar (Populus trichocarpa). PLoS One. 2012;7:e31149.10.1371/journal.pone.0031149
- Soderman E, Mattsson J, Engstrom P. The Arabidopsis homeobox gene ATHB-7 is induced by water deficit and by abscisic acid. Plant J. 1996;10:375–381.10.1046/j.1365-313X.1996.10020375.x
- Wang Y, Henriksson E, Söderman E, Henriksson KN, Sundberg E, Engström P. The Arabidopsis homeobox gene, ATHB16, regulates leaf development and the sensitivity to photoperiod in Arabidopsis. Dev. Biol. 2003;264:228–239.10.1016/j.ydbio.2003.07.017
- Aoyama T, Dong CH, Wu Y, Carabelli M, Sessa G, Ruberti I, Morelli G, Chua NH. Ectopic expression of the Arabidopsis transcriptional activator Athb-1 alters leaf cell fate in tobacco. Plant Cell. 1995;7:1773–1785.10.1105/tpc.7.11.1773
- Hanson J, Johannesson H, Engstro¨m P. Sugar-dependent alterations in cotyledon and leaf development in transgenic plants expressing the HDZhdip gene ATHB13. Plant Mol. Biol. 2001;45:247–262.10.1023/A:1006464907710
- Mattsson J, Ckurshumova W, Berleth T. Auxin signaling in Arabidopsis leaf vascular development. Plant Physiol. 2003;131:1327–1339.10.1104/pp.013623
- Barrero JM, Millar AA, Griffiths J, Czechowski T, Scheible WR, Udvardi M, Reid JB, Ross JJ, Jacobsen JV, Gubler F. Gene expression profiling identifies two regulatory genes controlling dormancy and ABA sensitivity in Arabidopsis seeds. Plant J. 2010;61:611–622.10.1111/tpj.2010.61.issue-4
- Johannesson H, Wang Y, Hanson J, Engström P. The arabidopsis thaliana homeobox gene ATHB5 is a potential regulator of abscisic acid responsiveness in developing seedlings. Plant Mol Biol. 2003;51:719–729.10.1023/A:1022567625228
- Himmelbach A, Hoffmann T, Leube M, Höhener B, Grill E. Homeodomain protein ATHB6 is a target of the protein phosphatase ABI1 and regulates hormone responses in Arabidopsis. EMBO J. 2002;21:3029–3038.10.1093/emboj/cdf316
- Valdés AE, Övernäs E, Johansson H, Rada-Iglesias A, Engström P. The homeodomain-leucine zipper (HD-Zip) class I transcription factors ATHB7 and ATHB12 modulate abscisic acid signalling by regulating protein phosphatase 2C and abscisic acid receptor gene activities. Plant Mol. Biol. 2012;80:405–418.10.1007/s11103-012-9956-4
- Deng X, Phillips J, Bräutigam A, Engström P, Johannesson H, Ouwerkerk PB, Ruberti I, Salinas J, Vera P, Iannacone R, Meijer AH, Bartels D. A homeodomain leucine zipper gene from Craterostigma plantagineum regulates abscisic acid responsive gene expression and physiological responses. Plant Mol. Biol. 2006;61:469–489.10.1007/s11103-006-0023-x
- Zúñiga-Mayo VM, Marsch-Martínez N, de Folter S. The class II HD-ZIP JAIBA gene is involved in meristematic activity and important for gynoecium and fruit development in Arabidopsis. Plant Signal. Behav. 2012;7:1501–1503.10.4161/psb
- Bou-Torrent J, Salla-Martret M, Brandt R, Musielak T, Palauqui JC, Martínez-García JF, Wenkel S. ATHB4 and HAT3, two class II HD-ZIP transcription factors, control leaf development in Arabidopsis. Plant Signal. Behav. 2012;7:1382–1387.10.4161/psb
- Steindler C, Carabelli M, Borello U, Morelli G, Ruberti I. Phytochrome A, phytochrome B and other phytochrome(s) regulate ATHB-2 gene expression in etiolated and green Arabidopsis plants. Plant, Cell Environ. 1997;20:759–763.10.1046/j.1365-3040.1997.d01-123.x
- McConnell JR, Emery J, Eshed Y, Bao N, Bowman J, Barton MK. Role of PHABULOSA and PHAVOLUTA in determining radial patterning in shoots. Nature. 2001;411:709–713.10.1038/35079635
- Green KA, Prigge MJ, Katzman RB, Clark SE. CORONA, a member of the class III homeodomain leucine zipper gene family in Arabidopsis, regulates stem cell specification and organogenesis. Plant Cell. 2005;17:691–704.10.1105/tpc.104.026179
- Ohashi-Ito K, Kubo M, Demura T, Fukuda H. Class III homeodomain leucine-zipper proteins regulate xylem cell differentiation. Plant Cell Physiol. 2005;46:1646–1656.10.1093/pcp/pci180
- Zhong R, Ye ZH. Amphivasal vascular bundle 1, a gain-of-function mutation of the IFL1/REV gene, is associated with alterations in the polarity of leaves, stems and carpels. Plant Cell Physiol. 2004;45:369–385.10.1093/pcp/pch051
- Baima S, Possenti M, Matteucci A, Wisman E, Altamura MM, Ruberti I, Morelli G. The arabidopsis ATHB-8 HD-zip protein acts as a differentiation-promoting transcription factor of the vascular meristems. Plant Physiol. 2001;126:643–655.10.1104/pp.126.2.643
- Abe M, Katsumata H, Komeda Y, Takahashi T. Regulation of shoot epidermal cell differentiation by a pair of homeodomain proteins in Arabidopsis. Development. 2003;130:635–643.10.1242/dev.00292
- Vernoud V, Laigle G, Rozier F, Meeley RB, Perez P, Rogowsky PM. The HD-ZIP IV transcription factor OCL4 is necessary for trichome patterning and anther development in maize. Plant J. 2009;59:883–894.10.1111/tpj.2009.59.issue-6
- Kubo H, Peeters AJ, Aarts MG, Pereira A, Koornneef M. ANTHOCYANINLESS2, a homeobox gene affecting anthocyanin distribution and root development in Arabidopsis. Plant Cell. 1999;11:1217–1226.10.1105/tpc.11.7.1217
- Yang C, Li H, Zhang J, Luo Z, Gong P, Zhang C, Li J, Wang T, Zhang Y, Lu Y, Ye Z. A regulatory gene induces trichome formation and embryo lethality in tomato. Proc. Nat. Acad. Sci. USA. 2012;108:11836–11841.
- Tomato Genome Consortium. Nature. 2012;485:635–641.
- Wilkins MR, Gasteiger E, Bairoch A, Sanchez JC, Williams KL, Appel RD, Hochstrasser DF. Protein identification and analysis tools in the ExPASy server. Methods Mol. Biol. 1999;112:531–552.
- Yu CS, Lin CJ, Hwang JK. Predicting subcellular localization of proteins for Gram-negative bacteria by support vector machines based on n-peptide compositions. Protein Sci. 2004;13:1402–1406.10.1110/(ISSN)1469-896X
- Tamura K, Peterson D, Peterson N, Stecher G, Nei M, Kumar S. MEGA5: molecular evolutionary genetics analysis using maximum likelihood, evolutionary distance, and maximum parsimony methods. Mol. Biol. Evol. 2001;28:2731–2739.
- Bailey TL, Boden M, Buske FA, Frith M, Grant CE, Clementi L, Ren J, Li WW, Noble WS. MEME SUITE: tools for motif discovery and searching. Nucleic Acids Res. 2009;37:W202–W208.10.1093/nar/gkp335
- Guo AY, Zhu QH, Chen X, Luo JC. GSDS: a gene structure display server. Hereditas. 2007;29:1023–1026.10.1360/yc-007-1023
- Higo K, Ugawa Y, Iwamoto M, Korenaga T. Plant cis-acting regulatory DNA elements (PLACE) database: 1999. Nucleic Acids Res. 1999;27:297–300.10.1093/nar/27.1.297
- Henriksson E, Olsson AS, Johannesson H, Johansson H, Hanson J, Engström P, Söderman E. Homeodomain leucine zipper class I genes in Arabidopsis. Expression patterns and phylogenetic relationships. Plant Physiol. 2005;139:509–518.10.1104/pp.105.063461
- Agalou A, Purwantomo S, Overnäs E, Johannesson H, Zhu X, Estiati A, de Kam RJ, Engström P, Slamet-Loedin IH, Zhu Z, Wang M, Xiong L, Meijer AH, Ouwerkerk PB. A genome-wide survey of HD-Zip genes in rice and analysis of drought-responsive family members. Plant Mol. Biol. 2008;66:87–103.10.1007/s11103-007-9255-7
- Zhao Y, Zhou Y, Jiang H, Li X, Gan D, Peng X, Zhu S, Cheng B. Systematic analysis of sequences and expression patterns of drought-responsive members of the HD-Zip gene family in maize. PLoS One. 2011;6:e28488.10.1371/journal.pone.0028488
- Ciarbelli AR, Ciolfi A, Salvucci S, Ruzza V, Possenti M, Carabelli M, Fruscalzo A, Sessa G, Morelli G, Ruberti I. The Arabidopsis homeodomain-leucine zipper II gene family: diversity and redundancy. Plant Mol. Biol. 2008;68:465–478.10.1007/s11103-008-9383-8
- Mayda E, Tornero P, Conejero V, Vera P. A tomato homeobox gene (HD-zip) is involved in limiting the spread of programmed cell death. Plant J. 1999;20:591–600.10.1046/j.1365-313X.1999.00633.x
- Bartley GE, Ishida BK. Ethylene-sensitive and insensitive regulation of transcription factor expression during in vitro tomato sepal ripening. J. Exp. Bot. 2007;58:2043–2051.10.1093/jxb/erm075
- Lin ZF, Hong Y, Yin M, Li C, Zhang K, Grierson D. A tomato HD-Zip homeobox protein, LeHB-1, plays an important role in floral organogenesis and ripening. Plant J. 2008;55:301–310.10.1111/tpj.2008.55.issue-2
- Turchi L, Carabelli M, Ruzza V, Possenti M, Sassi M, Peñalosa A, Sessa G, Salvi S, Forte V, Morelli G, Ruberti I. Arabidopsis HD-Zip II transcription factors control apical embryo development and meristem function. Development. 2013;140:2118–2129.10.1242/dev.092833
- Meissner R, Theres K. Isolation and characterization of the tomato homeobox gene THOM1. Planta. 1995;195:541–547.
- Takada S, Takada N, Yoshida A. Induction of epidermal cell fate in Arabidopsis shoots. Plant Signal. Behav. 2013;8:e26236.10.4161/psb
- Hara K, Yokoo T, Kajita R, Onishi T, Yahata S, Peterson KM, Torii KU, Kakimoto T. Epidermal cell density is autoregulated via a secretory peptide, EPIDERMAL PATTERNING FACTOR 2 in Arabidopsis leaves. Plant Cell Physiol. 2009;50:1019–1031.10.1093/pcp/pcp068
- Takada S, Takada N, Yoshida A. ATML1 promotes epidermal cell differentiation in Arabidopsis shoots. Development. 2013;140:1919–1923.10.1242/dev.094417
- Peterson KM, Shyu C, Burr CA, Horst RJ, Kanaoka MM, Omae M, Sato Y, Torii KU. Arabidopsis homeodomain-leucine zipper IV proteins promote stomatal development and ectopically induce stomata beyond the epidermis. Development. 2013;140:1924–1935.10.1242/dev.090209
- Nadakuduti SS, Pollard M, Kosma DK, Allen C Jr, Ohlrogge JB, Barry CS. Pleiotropic phenotypes of the sticky peel mutant provide new insight into the role of CUTIN DEFICIENT2 in epidermal cell function in tomato. Plant Physiol. 2012;159:945–960.10.1104/pp.112.198374
- Simpson SD, Nakashima K, Narusaka Y, Seki M, Shinozaki K, Yamaguchi-Shinozaki K. Two different novel cis-acting elements of erd1, a clpA homologous Arabidopsis gene function in induction by dehydration stress and dark-induced senescence. Plant J. 2003;33:259–270.10.1046/j.1365-313X.2003.01624.x
- Stålberg K, Ellerstöm M, Ezcurra I, Ablov S, Rask L. Disruption of an overlapping E-box/ABRE motif abolished high transcription of the napA storage-protein promoter in transgenic Brassica napus seeds. Planta. 1996;199:515–519.
- Kaplan B, Davydov O, Knight H, Galon Y, Knight MR, Fluhr R, Fromm H. Rapid transcriptome changes induced by cytosolic Ca2+ transients reveal ABRE-related sequences as Ca2+-responsive cis elements in Arabidopsis. Plant Cell. 2006;18:2733–2748.10.1105/tpc.106.042713
- Oh SJ, Song SI, Kim YS, Jang HJ, Kim SY, Kim M, Kim YK, Nahm BH, Kim JK. Arabidopsis CBF3/DREB1A and ABF3 in transgenic rice increased tolerance to abiotic stress without stunting growth. Plant Physiol. 2005;138:341–351.10.1104/pp.104.059147
- Dunn MA, White AJ, Vural S, Hughes MA. Identification of promoter elements in a low-temperature-responsive gene (blt4.9) from barley (Hordeum vulgare L.). Plant Mol. Biol. 1998;38:551–564.10.1023/A:1006098132352
- Kim HJ, Kim YK, Park JY, Kim J. Light signalling mediated by phytochrome plays an important role in cold-induced gene expression through the C-repeat/dehydration responsive element (C/DRE) in Arabidopsis thaliana. Plant J. 2002;29:693–704.10.1046/j.1365-313X.2002.01249.x
- Urao T, Yamaguchi-Shinozaki K, Urao S, Shinozaki K. An Arabidopsis myb homolog is induced by dehydration stress and its gene product binds to the conserved MYB recognition sequence. Plant Cell. 1993;5:1529–1539.10.1105/tpc.5.11.1529
- Abe H, Yamaguchi-Shinozaki K, Urao T, Iwasaki T, Hosokawa D, Shinozaki K. Role of arabidopsis MYC and MYB homologs in drought- and abscisic acid-regulated gene expression. Plant Cell. 1997;9:1859–1868.
- Park HC, Kim ML, Kang YH, Jeon JM, Yoo JH, Kim MC, Park CY, Jeong JC, Moon BC, Lee JH, Yoon HW, Lee SH, Chung WS, Lim CO, Lee SY, Hong JC, Cho MJ. Pathogen- and NaCl-induced expression of the SCaM-4 promoter is mediated in part by a GT-1 box that interacts with a GT-1-like transcription factor. Plant Physiol. 2004;135:2150–2161.10.1104/pp.104.041442
- Lijavetzky D, Carbonero P, Vicente-Carbajosa J. Genome-wide comparative phylogenetic analysis of the rice and Arabidopsis Dof gene families. BMC Evol. Biol. 2003;3:17.10.1186/1471-2148-3-17
- Liu Y, Jiang HY, Chen WJ, Xing G, Hu Z, Zhang Y, Sun Q, Ni Z. Genome-wide analysis of the auxin response factor (ARF) gene family in maize (Zea mays). Plant Growth Regul. 2003;63:225–234.
- Huang S, Gao Y, Liu J, Peng X, Niu X, Fei Z, Cao S, Liu Y. Genome-wide analysis of WRKY transcription factors in Solanum lycopersicum. Mol. Genet. Genomics. 2012;287:495–513.10.1007/s00438-012-0696-6
- Van der Hoeven RC, Ronning J Giovannoni, Martin G, Tanksley S. Deductions about the number organization, and evolution of genes in the tomato genome based on analysis of a large expressed sequence tag collection and selective genomic sequencing. Plant Cell. 2002;14:1441–1456.10.1105/tpc.010478
- Schlueter JA, Dixon P, Granger C, Grant D, Clark L, Doyle JJ, Shoemaker RC. Mining EST databases to resolve evolutionary events in major crop species. Genome. 2004;47:868–876.10.1139/g04-047
- Prigge MJ, Otsuga D, Alonso JM, Ecker JR, Drews GN, Clark SE. Class III homeodomain-leucine zipper gene family members have overlapping, antagonistic, and distinct roles in Arabidopsis development. Plant Cell. 2005;17:61–76.10.1105/tpc.104.026161
- Nakamura M, Katsumata H, Abe M, Yabe N, Komeda Y, Yamamoto KT, Takahashi T. Characterization of the class IV homeodomain-Leucine zipper gene family in Arabidopsis. Plant Physiol. 2006;141:1363–1375.10.1104/pp.106.077388
- Jain M, Tyagi AK, Khurana JP. Genome-wide identification, classification, evolutionary expansion and expression analyses of homeobox genes in rice. FEBS J. 2008;275:2845–2861.10.1111/j.1742-4658.2008.06424.x
- Koonin EV. Orthologs, paralogs, and evolutionary genomics. Annu. Rev. Genet. 2005;39:309–338.10.1146/annurev.genet.39.073003.114725
- Li WH, Yang J, Gu X. Expression divergence between duplicate genes. Trends Genet. 2005;21:602–607.10.1016/j.tig.2005.08.006
- Ku HM, Vision T, Liu J, Tanksley SD. Comparing sequenced segments of the tomato and Arabidopsis genomes: large-scale duplication followed by selective gene loss creates a network of synteny. Proc. Nat. Acad. Sci. USA. 2000;97:9121–9126.10.1073/pnas.160271297
- Maere S, De Bodt S, Raes J, Casneuf T, Van Montagu M, Kuiper M, Van de Peer Y. Modeling gene and genome duplications in eukaryotes. Proc. Nat. Acad. Sci. USA. 2005;102:5454–5459.10.1073/pnas.0501102102
- Blanc G, Hokamp K, Wolfe KH. A recent polyploidy superimposed on older large-scale duplications in the Arabidopsis genome. Genome Res. 2003;13:137–144.10.1101/gr.751803