Abstract
Rhodococcus rubropertinctus N82 possesses unique regiospecific hydroxylation activity in biotransformation of compounds. In this study, the ability of whole cells of the strain R. rubropertinctus N82 in biotransformation was studied. The hydroxylation activity resulted in transforming 6,7-dihydro-4H-thieno[3,2-c]-pyridine-5-carboxylic acid tert-butyl ester (LS1) into 2-hydroxy-6,7-dihydro-4H-thieno[3,2-c]-pyridine-5-carboxylic acid tert-butyl ester (LP1), a pharmaceutical intermediate. By optimizing conditions for the hydroxylating biotransformation using whole cells of R. rubropertinctus N82 as biocatalyst, 3.3 mM LP1 was successfully produced from 4 mM LS1 with a molar yield of 83%. Thus, effective method was newly developed to produce LP1, which is a synthetic intermediate of a platelet inhibitor active pharmaceutical ingredient drug, prasugrel.
Graphical Abstract
By the hydroxylating biotransformation using whole cells of Rhodococcus rubropertinctus N82 as biocatalyst, a pharmaceutical intermediate was successfully produced with a molar yield of 83%.

Monooxygenation reactions are important and are often used in the industrial production of fine chemicals and in developing pharmaceutical intermediates.Citation1) Although use of chemical oxidation in monooxygenation reactions have major disadvantages such as non-specific oxidation and low regioselective monooxygenation leading to formation of various byproducts, monooxygenation enzymes are usually highly selective and hence, are more valid as biocatalysts for industrial monooxygenation.Citation2,3) Several monooxygenation enzymes that are also known to hydroxylate wide range of chemical compounds have been reported earlier, such as cytochrome P450 monooxygenases,Citation4) flavin-containing monooxygenases,Citation5) and Fe(II)/α-ketoglutarate-dependent dioxygenases.Citation6–9) These enzymes are known to act on non-activated carbon–hydrogen bonds in alkanes, fatty acids, terpenes, and steroids and usually exhibit high regioselective hydroxylation- and chemoselectivity. Thus, monooxygenases are promising biocatalysts for many hydroxylation reactions in industrial processes of high-value added products.
2-Hydroxy-6,7-dihydro-4H-thieno[3,2-c]-pyridine-5-carboxylic acid tert-butyl ester (LP1, Fig. (A)) is used as a synthetic intermediate of prasugrel, a platelet inhibitor active pharmaceutical ingredient.Citation10) One of the possible synthetic routes of LP1 is hydroxylation of a precursor, 6,7-dihydro-4H-thieno[3,2-c]-pyridine-5-carboxylic acid tert-butyl ester (LS1, Fig. (B)).Citation11) However, it would be difficult to hydroxylate LS1 regioselectively by chemical hydroxylation, because there are several positions possible to be oxidized in the LS1 structure. In this work, we found that an actinomycete; Rhodococcus rubropertinctus N82 had hydroxylation activity of LS1 into LP1. After optimization of the conditions for cultivation and hydroxylation reaction, LP1 was effectively produced by the biotransformation using whole cells of this strain.
Materials and methods
Microbial screening conditions for biotransformation of LS1
LS1 and LP1 were provided from Lonza Ltd (Visp, Switzerland). Micro-organisms in a culture collection of Faculty of Agriculture, Kyoto University that include bacteria, actinomycetes, ascomycetes, and basidiomycetes were grown in basal medium containing 2.0% (w/v) glucose, 0.5% peptone, 0.4% yeast extract, 0.4% KH2PO4, 0.2% K2HPO4, and 0.02% MgSO4·7H2O at 28 °C with continuous shaking at 300 rpm for 48 h. After cultivation, 1/20 vol of the mixture of 40 mM LS1 and 16 mM β-cyclodextrin, which enhances the solubility of LS1, in 50% ethanol were added to the culture broth. The final concentrations were 2 mM LS1, 0.4 mM β-cyclodextrin, and 2.5% ethanol. The biotransformation was allowed to proceed at 28 °C with shaking at 300 rpm for 48 h. The supernatant was extracted with a mixture comprised of equal volume of chloroform and methanol, which was mixed thoroughly, and centrifuged at 3,000 rpm for 10 min. The extract in chloroform layer was further dried by evaporation, dissolved in ethyl acetate, and stored for analysis by high-performance liquid chromatography (HPLC).
High-performance liquid chromatography
The resulting compound was quantitatively measured by HPLC. HPLC analysis was performed with a LC10A-VP system (Shimadzu, Kyoto, Japan) equipped with an Inertsil ODS-2 column (4.6 × 250 mm, 5 μm, GL Sciences Inc., Tokyo, Japan) at 40 °C. Compounds were eluted with a gradient of acetonitrile/water (50–100% acetonitrile) for 20 min at a flow rate of 1.0 ml/min. LS1 and LP1 were detected using UV absorbance at 254 nm. The quantities of LP1 and LS1 were determined by using standard reference compounds. The electrospray ionization mass spectrometry was performed on a LCMS-2010A system (Shimadzu).
Analysis of appropriate culture conditions for optimal growth of R. rubropertinctus N82
The effects of the culture medium composition for R. rubropertinctus N82 (JCM 3204; Japan Collection of Microorganisms, RIKEN BioResource Center, Japan) on biotransformation of LS1 into LP1 were investigated. For optimization of carbon source in the basal medium, 2.0% of fructose, sorbitol, mannitol, glycerol, galactose, or ribose were used instead of glucose. For optimization of nitrogen source in basal medium, 0.4% of malt extract, meat extract, soybean meal, tryptone, or ammonium sulfate were used instead of peptone. For optimization with regards to the concentration of yeast extract in the basal medium, 0.2–6.0% of yeast extract was used. R. rubropertinctus N82 was pre-cultured individually in 20 ml of each medium at 28 °C with shaking at 300 rpm for 48 h. The individual inoculum for each culture (2% v/v) was used to inoculate each 100 ml of respective broth, and incubated at 28 °C with shaking at 300 rpm. After 48 h, 4 mM LS1, 0.4 mM β-cyclodextrin, and 2.5% ethanol was added to the cultures and incubated for up to 48 h. In order to optimize the cultivation time to obtain R. rubropertinctus N82 cells with high LP1 productivity, the cultivation was carried out in the same way as above, except that the optimized medium contained 2.0% glucose, 0.5% peptone, 1.2% yeast extract, 0.4% KH2PO4, 0.2% K2HPO4, and 0.02% MgSO4·7H2O was used. At various time points of the cultivation such as 40, 48, 60, and 72 h, 4 mM LS1, 0.4 mM β-cyclodextrin, and 2.5% ethanol was added to the cultures and incubated for up to 48 h. Both LS1 and LP1 in the reaction mixture were analyzed by the HPLC.
Standardization of optimal conditions for biotransformation
In order to optimize the amount of ethanol as an additive to the reaction mixture, R. rubropertinctus N82 cells were cultivated in the optimized medium for 60 h, and 4 mM LS1, 16 mM β-cyclodextrin, and 0.5–2.5% ethanol was added to the cultures and incubated at 28 °C with shaking at 300 rpm for 48 h. Also, optimal concentration of LS1 used in the reaction mixture was investigated. R. rubropertinctus N82 cells were cultivated in the optimized medium for 60 h, and 4–20 mM LS1, 16 mM β-cyclodextrin, and 1.5% ethanol was added to the cultures and incubated at 28 °C with shaking at 300 rpm for 48 h. LS1 and LP1 in the reaction mixture were analyzed by HPLC.
Results and discussion
Screening of micro-organisms producing LP1 from LS1
In this paper we determined a pharmaceutical intermediate via biotransformation of LS1 in R. rubropertinctus N82 strain. Our collection comprising many kinds of micro-organisms including bacteria, actinomycetes, ascomycetes, and basidiomycetes were analyzed for its respective ability to selectively hydroxylate and transform LS1 into LP1. In this screening of a total of 1345 strains, a novel peak corresponding to LP1 on the HPLC chromatogram was detected in the reaction mixtures from those of 15 strains that were involved in the metabolic process which mainly belong to the actinomycetes genera. Among those, R. rubropertinctus N82 showed increased production and activity for the oxidation of LS1 to LP1 and hence, this strain was detected as the predominant species involved in the metabolic process. With regards to LC-MS analysis of the reaction mixture, a molecular weight of the compound corresponding to LP1 was observed in the R. rubropertinctus N82 strain. Furthermore, this compound showed the same Rf value in thin-layer chromatography analysis to and the same UV–vis spectrum as the authentic standard of LP1 (data not shown). Thus, R. rubropertinctus N82 was confirmed to possess hydroxylation activity towards LS1 and transform to LP1. This work demonstrates for the first time using micro-organisms that R. rubropertinctus N82 strain is able to biotransform LS1 to LP1. The genus Rhodococcus is demonstrated to have the ability to degrade a large number of recalcitrant and toxic organic compounds, and many types of monooxygenases were found to be involved in its various degradation pathways.Citation12)
Optimization of culture conditions of R. rubropertinctus N82 for biotransformation
To obtain active cells of R. rubropertinctus N82 for LP1 production, the effect of various carbon sources in the culture medium was examined. Various carbohydrates were used as a sole source of carbon, and the production of LP1 was evaluated in hydroxylation reaction of LS1 (Fig. (A)). R. rubropertinctus N82 grew to the same extent with all carbon sources tested. R. rubropertinctus N82 cells demonstrated maximum LP1 production when glucose was used as the carbon source in the hydroxylation reaction of LS1. Similarly, the effect of various nitrogen sources in the culture medium was examined. Various nitrogen-containing reagents were used as the nitrogen source, and the production of LP1 was evaluated in hydroxylation reaction of LS1 (Fig. (B)). R. rubropertinctus N82 grew to the same extent with all nitrogen sources tested. When peptone was used as the nitrogen source, R. rubropertinctus N82 demonstrated maximum LP1 production in the hydroxylation reaction of LS1. And also, the effects of concentration of yeast extract in the culture medium were examined. Various concentration of yeast extract was used, and production of LP1was evaluated in hydroxylation reaction of LS1 (Fig. (C)). R. rubropertinctus N82 grew to the same extent at all concentrations tested. When the medium containing 1.2% yeast extract was used, R. rubropertinctus N82 demonstrated maximum LP1 production in the hydroxylation reaction of LS1. Although we tested the degree of biotransformation by hydroxylating reaction of LS1 using the cell lysate prepared from R. rubropertinctus N82 cells cultivated on the optimized culture medium, no LP1 production was observed in the reaction mixture. Thus, purification and identification of the enzyme responsible for hydroxylation of LP1 in this strain will remain scope for future work.
Fig. 2. Standardization of optimal conditions for culture medium of R. rubropertinctus N82 for the biotransformation of LS1 into LP1.
Note: (A) LP1 production as observed in cultures grown in modified basal media containing various kinds of carbohydrates. (B) LP1 production of cells cultivated in modified basal media containing various kinds of nitrogen-containing reagents. (C) LP1 production of cells cultivated in modified basal media containing various concentration of yeast extract.
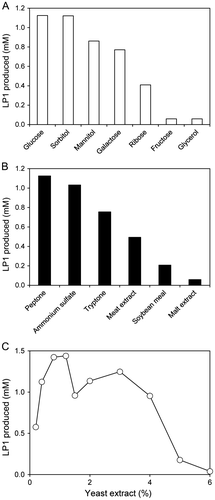
Optimization of reaction conditions for biotransformation
By using the optimized culture medium, the effect of cultivation time of R. rubropertinctus N82 on the biotransformation was investigated. R. rubropertinctus N82 cells were cultured at various time points, and were used to analyze hydroxylation reaction of LS1 (Fig. ). As the result, R. rubropertinctus N82 cells with 60 h-cultivation time showed the maximum degree of biotransformation with increased production of LP1. Also, the effect of additive amount of ethanol used for solvent to dissolve LS1 on the biotransformation was investigated (Table ). As a result, R. rubropertinctus N82 showed the highest production with 3.0 mM of LP1 at 48 h when 1.5% of ethanol was used. Interestingly, only at the concentration of 0.5% ethanol, initial production and following degradation of LP1 were observed in the reaction mixture. This result suggested that high concentration of ethanol (1.5% and more) had inhibitory effects and could influence the metabolism of LP1. Effects of concentration of the substrate, LS1, were also examined in the hydroxylation reaction with R. rubropertinctus N82 cells (Table ). When 8 mM concentration of LS1 was used in the reaction mixture, the production of LP1 increased to 5.6 mM. However, when a concentration of more than 8 mM of LS1 was used, decreased molar yield of LP1 was observed. The maximum production yield of LP1 was observed to be 75% when 4 mM concentration of LS1 was used (Fig. ).
Fig. 3. Cultivation time optimization of R. rubropertinctus N82 for the biotransformation of LS1 into LP1.
Note: The LP1 production was carried out with cells cultivated at various time in the optimized medium. Production of LP1 (▲) and wet cell weight of R. rubropertinctus N82 used (○) in the reaction mixtures were shown.
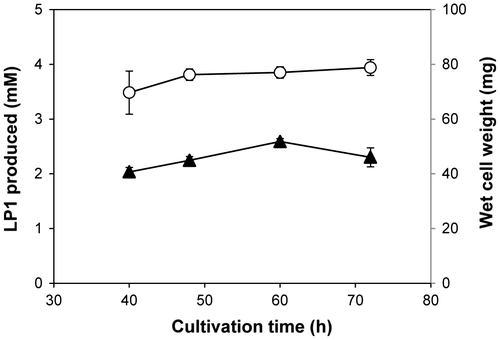
Table 1. Biotransformation of LS1 into LP1 in R. rubropertinctus N82 strain cultivated under various ethanol concentrations.
Fig. 4. Time course for the optimized biotransformation of LS1 into LP1.
Note: The biotransformation of 4 mM LS1 was carried out with the cells cultivated in the optimized medium. The concentrations of LS1 (Δ) and LP1 (▲), and wet cell weight of R. rubropertinctus N82 (○) in course of the reaction time were shown.
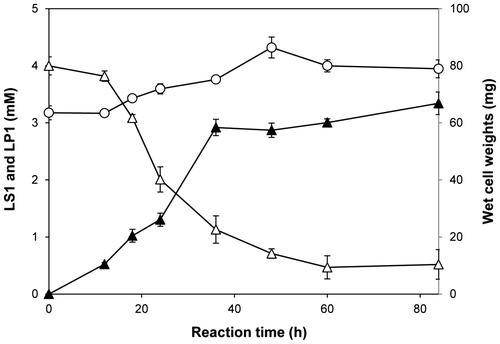
LP1 production under the optimum conditions
In an attempt to increase the degree of biotransformation, various conditions were attempted for the most favorable growth morphology. According to the aforementioned results, the ideal conditions for R. rubropertinctus N82 was at cultivation in the optimized medium containing 2.0% (w/v) glucose, 0.5% peptone, 1.2% yeast extract, 0.4% KH2PO4, 0.2% K2HPO4, and 0.02% MgSO4·7H2O at 28 °C with shaking at 300 rpm for 60 h. The degree of biotransformation was most efficient when 4 mM of LS1 was used for the hydroxylation reaction and 0.4 mM β-cyclodextrin, and 1.5% ethanol were added and the reaction mixture was incubated at 28 °C with shaking at 300 rpm. After 84 h, the production of LP1 reached the maximum at a value of 3.3 mM which corresponds to a molar yield of 83%. The specific conversion rate of LP1 based on the consumed LS1 was 96%. No significant byproducts were observed at the point. Further incubation resulted in decreased LP1 accumulation.
Conclusion
In this study we determined the optimal conditions for the degree of transformation and potent hydroxylation activity of various micro-organisms. The work demonstrates for the first time that using R. rubropertinctus N82, had the maximum degree of biotransformation and hydroxylation activity and generated a pharmaceutical intermediate, LP1. By optimization of conditions for the biotransformation, the pharmaceutical intermediate LP1 was formed regioselectively and was produced to be used as a synthetic intermediate of a platelet inhibitor active pharmaceutical ingredient drug, prasugrel. Further research will focus on identification of the enzyme responsible for the hydroxylation reaction, and once such an enzyme is identified, the research will focus on production of a valuable and effective hydroxylation biocatalyst by creating a recombinant version of the respective enzyme.
Supplemental material
The supplemental material for this paper is available at http://dx.doi.org/10.1080/09168451.2014.925781.
Notes
Abbreviations: HPLC, high-performance liquid chromatography; LS1, 6,7-dihydro-4H-thieno[3,2-c]-pyridine-5-carboxylic acid tert-butyl ester; LP1, 2-hydroxy-6,7-dihydro-4H-thieno[3,2-c]-pyridine-5-carboxylic acid tert-butyl ester.
References
- Hudlucky M. Oxidations in organic chemistry. Washington (DC): American Chemical Society; 1990.
- Ullrich R, Hofrichter M. Enzymatic hydroxylation of aromatic compounds. Cell. Mol. Life Sci. 2007;64:271–293.10.1007/s00018-007-6362-1
- Urlacher V, Schmid R. Recent advances in oxygenase-catalyzed biotransformations. Curr. Opin. Chem. Biol. 2006;10:156–161.10.1016/j.cbpa.2006.02.001
- Urlacher V, Eiben S. Cytochrome P450 monooxygenases: perspectives for synthetic application. Trends Biotechnol. 2006;24:324–330.10.1016/j.tibtech.2006.05.002
- Stewart J. Cyclohexanone monooxygenase: a useful reagent for asymmetric Baeyer-Villiger reactions. Curr. Org. Chem. 1998;2:195–216.
- Hibi M, Kawashima T, Kasahara T, Sokolov PM, Smirnov SV, Kodera T, Sugiyama M, Shimizu S, Yokozeki K, Ogawa J. A novel Fe(II)/α-ketoglutarate-dependent dioxygenase from Burkholderia ambifaria has β-hydroxylating activity of N-succinyl L-leucine. Lett. Appl. Microbiol. 2012;55:414–441.
- Hibi M, Kawashima T, Kodera T, Smirnov SV, Sokolov PM, Sugiyama M, Shimizu S, Yokozeki K, Ogawa J. Characterization of Bacillus thuringiensis L-isoleucine dioxygenase for production of useful amino acids. Appl. Environ. Microbiol. 2011;77:6926–6930.10.1128/AEM.05035-11
- Hibi M, Kawashima T, Sokolov PM, Smirnov SV, Kodera T, Sugiyama M, Shimizu S, Yokozeki K, Ogawa J. L-leucine 5-hydroxylase of Nostoc punctiforme is a novel type of Fe(II)/α-ketoglutarate-dependent dioxygenase that is useful as a biocatalyst. Appl. Microbiol. Biotechnol. 2013;97:2467–2472.10.1007/s00253-012-4136-7
- Hibi M, Kawashima T, Yajima H, Smirnov SV, Kodera T, Sugiyama M, Shimizu S, Yokozeki K, Ogawa J. Enzymatic synthesis of chiral amino acid sulfoxides by Fe(II)/α-ketoglutarate-dependent dioxygenase. Tetrahedron: Asymmetry. 2013;24:990–994.10.1016/j.tetasy.2013.07.017
- Jakubowski J, Winters K, Naganuma H, Wallentin L. Prasugrel: a novel thienopyridine antiplatelet agent. A review of preclinical and clinical studies and the mechanistic basis for its distinct antiplatelet profile. Cardiovasc. Drug Rev. 2007;25:357–374.10.1111/cdr.2007.25.issue-4
- Thijs L, Zhu J, Overeem A, Keltens R. WO 2011/110219 A1 (2011 Sep 15).
- Larkin M, Kulakov L, Allen C. Biodegradation and Rhodococcus – masters of catabolic versatility. Curr. Opin. Biotechnol. 2005;16:282–290.10.1016/j.copbio.2005.04.007