Abstract
Removal of a side product to overcome unfavorable equilibrium is a prerequisite for the asymmetric amination of ketones using ω-transaminase (ω-TA). Alanine has been preferred as an amino donor because its deamination product (i.e. pyruvate) is easily removable by several enzymatic methods. Here, we demonstrated that the removal of pyruvate by an innate metabolic pathway could afford equilibrium shift of the ω-TA reactions.
A wide range of chiral amines are used as essential building blocks of diverse pharmaceutical drugs.Citation1,2) Compared with kinetic resolution of racemic amines permitting only 50% yield, asymmetric amination of ketones is preferred for the preparation of the chiral amines owing to a twofold higher maximum yield. To date, ω-transaminase (ω-TA) is the only naturally occurring enzyme that can mediate the asymmetric reductive amination of ketones, which renders the enzyme attractive for the industrial production of chiral amines.Citation3,4)
One of the challenges for the ω-TA-catalyzed amination of ketones is unfavorable reaction equilibrium.Citation5,6) For example, the equilibrium constant (Keq) of the transamination between acetophenone and alanine was determined to be 8.8 × 10−4.Citation7) To address this problem, extensive studies have focused on shifting the unfavorable equilibrium to a product side by coproduct removal.Citation5,6,8) To this end, isopropylamine and alanine have been preferred as amino donors owing to the facile removal of resulting deamination products (i.e. acetone and pyruvate, respectively). Although acetone can be easily removed at a reduced pressure due to high volatility,Citation3,9) a drawback of using isopropylamine is its low reactivity towards most ω-TAs, unless modified by extensive protein engineering as demonstrated by Savile et al.Citation3)
In contrast, all known ω-TAs show high activities to alanine. Moreover, the removal of pyruvate can be easily achieved by coupling an additional enzyme, including alanine dehydrogenase, lactate dehydrogenase, acetolactate synthase, and pyruvate decarboxylase.Citation9–12) The reason why several enzymatic options are available to convert pyruvate into other chemicals is that pyruvate is a pivotal metabolite connecting the glycolytic pathway and the TCA cycle to extract chemical energy from glucose as well as to provide carbon skeletons required for synthesizing diverse metabolites. We reasoned that the removal of pyruvate to cope with the unfavorable equilibrium could be implemented by exploiting the native cellular metabolism, instead of using the auxiliary enzymes. To test this idea, we examined how intracellular pyruvate surplus affected the amination reaction and verified whether the concept of the metabolically driven equilibrium shift enabled to achieve amination conversion above a thermodynamic limit.
Pyruvate is an end product of the glycolytic pathway and enters the TCA cycle under aerobic conditions after being transformed into acetyl CoA. We envisioned that pyruvate, generated from the ω-TA reaction between ketone and alanine, could be removed by the native metabolic pathway and thereby the whole cell reaction could afford equilibrium shift towards the synthesis of a chiral amine from a ketone precursor (Fig. ). However, to facilitate the effective pyruvate removal, cells should be carbon starved. As a proof-of-concept, we examined how intracellular pyruvate surplus, resulting from the glycolysis of glucose, affected the amination of acetophenone by L-alanine (Fig. ). The reactions were catalyzed by Escherichia coli BL21(DE3) cells overexpressing (S)-selective ω-TA cloned from Ochrobactrum anthropi (OATA).Citation13) The cells, carrying the pET28a(+) expression vectors harboring the ω-TA gene, were cultivated in LB medium (typically 300 mL) containing 50 μg kanamycin/mL at 37 °C. Protein expression was induced by IPTG (final concentration = 0.1 mM) around 0.5 OD600 and then the cells were allowed to grow further for 12 h. Cells were spun down at 10,000 g, washed twice with potassium phosphate buffer (50 mM, pH 7) and then resuspended in the same buffer. Reaction volume was 1 mL and the reactions were started by the addition of the cell suspension. The reaction mixtures were incubated at 37 °C under magnetic stirring. Aliquots of the reaction mixture were taken at predetermined reaction times and the produced (S)-α-methylbenzylamine ((S)-α-MBA) was analyzed by HPLC analysis using a Crownpak CR(−) column (Diacel Co., Japan) as described elsewhere.Citation14) Compared with the amination reaction in the absence of glucose where the concentration of (S)-α-MBA reached 1.29 mM after 6 h, the synthesis of (S)-α-MBA was dramatically slowed down (i.e. 0.04 mM at 6 h) in the reaction medium supplemented with glucose. This result suggests that the amination reaction was adversely influenced by increase in the intracellular pyruvate level presumably caused by pyruvate influx from the glycolytic pathway. We also found that the metabolic removal of pyruvate led to even higher conversion than the one obtained using isopropylamine. Substitution of L-alanine with the same concentration of isopropylamine in the absence of glucose resulted in 0.88 mM (S)-α-MBA at 6 h. This result indicates that the pyruvate removal strategy by the native metabolism might be exploited as effectively as the spontaneous acetone removal strategy using isopropylamine.
Fig. 1. Schematics of the metabolically driven amination of ketones using alanine as an amino donor.
Notes: Under aerobic conditions, pyruvate enclosed in the box is directed to the TCA cycle.
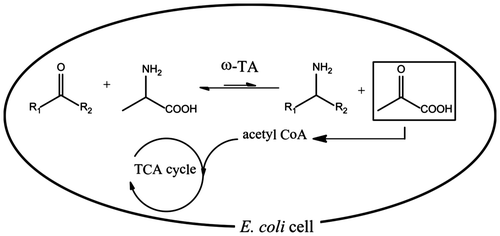
Fig. 2. Effect of metabolic supply of pyruvate to amination of ketone.
Notes: Reaction conditions were acetophenone (20 mM), L-alanine (200 mM), and whole cells expressing OATA (18 mg dry cell weight/mL). To provide the carbon-rich conditions, the reaction mixture was supplemented with glucose (100 mM).
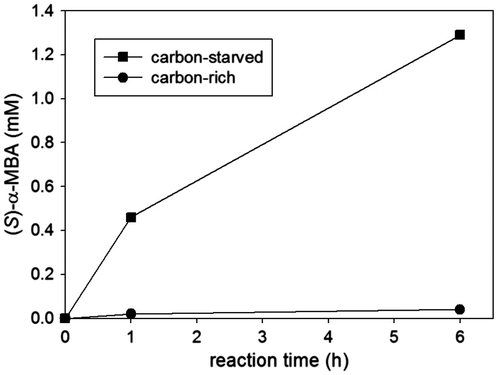
To demonstrate the asymmetric synthesis of chiral amine above a thermodynamic limit using the metabolic removal of pyruvate, we chose 2-butanone as a model ketone substrate. In the previous report, we could calculate the free energy change of the transamination between 2-butanone and alanine (ΔG = −12.89 kJ/mol), which led to Keq = 6.8 × 10−3.Citation14) Under the reaction conditions where 50 mM 2-butanone and 100 mM L-alanine were used, the equilibrium concentration of sec-butylamine was 5.4 mM. Quantitative chiral analyses of sec-butylamine and L-alanine were carried out using a Symmetry C18 column (Waters, USA) after pre-column derivatization with 2,3,4,6-tetra-O-acetyl-β-D-glucopyranosyl isothiocyanate as described elsewhere.Citation14) After 12 h, the amination reaction catalyzed by E. coli cells expressing OATA yielded 37.3 mM (S)-sec-butylamine (i.e. 75% conversion) that was far beyond the thermodynamic limit (Fig. ). We found that the concentration sum of (S)-sec-butylamine and L-alanine during the reaction was kept constant around the initial concentration of L-alanine, indicative of stoichiometric transfer of an amino group from L-alanine to 2-butanone.
Fig. 3. Metabolically driven asymmetric synthesis of (S)-sec-butylamine from 2-butanone.
Notes: Reaction conditions were 2-butanone (50 mM), L-alanine (100 mM), and whole cells expressing OATA (100 mg dry cell weight/mL). Dotted line represents a theoretical equilibrium limit of (S)-sec-butylamine production.
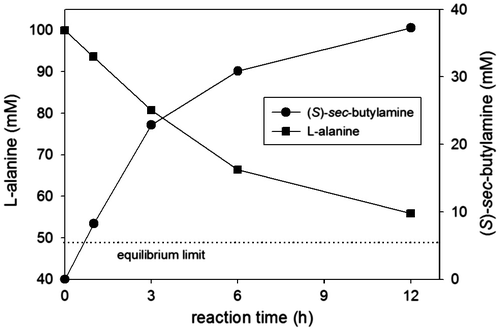
In conclusion, we demonstrated that the metabolic removal of pyruvate could be exploited to shift the unfavorable equilibrium of the ω-TA-catalyzed amination of ketones using alanine as an amino donor. In this study, the whole cell reactions were carried out without aeration that was required to elevate pyruvate efflux into the TCA cycle. However, vigorous aeration should lead to substantial loss of a volatile ketone substrate without a condenser incorporated into a reactor set-up. Therefore, to bring this concept into practical applications, a reactor design to support aerobic conditions without undesirable loss of a ketone substrate should be considered.
Funding
This work was supported by the Advanced Biomass R&D Center [grant number ABC-2011-0031358] through the National Research Foundation of Korea funded by the Ministry of Education, Science and Technology.
References
- Nugent TC. Chiral amine synthesis: methods, developments and applications. Wiley-VCH Verlag GmbH & Co. KGaA, Weinheim, Germany; 2010.
- Breuer M, Ditrich K, Habicher T, Hauer B, Kesseler M, Sturmer R, Zelinski T. Industrial methods for the production of optically active intermediates. Angew. Chem. Int. Ed. 2004;43:788–824.10.1002/(ISSN)1521-3773
- Savile CK, Janey JM, Mundorff EC, Moore JC, Tam S, Jarvis WR, Colbeck JC, Krebber A, Fleitz FJ, Brands J, Devine PN, Huisman GW, Hughes GJ. Biocatalytic asymmetric synthesis of chiral amines from ketones applied to sitagliptin manufacture. Science. 2010;329:305–309.10.1126/science.1188934
- Desai AA. Sitagliptin manufacture: a compelling tale of green chemistry, process intensification, and industrial asymmetric catalysis. Angew. Chem. Int. Ed. 2011;50:1974–1976.10.1002/anie.v50.9
- Malik MS, Park ES, Shin JS. Features and technical applications of w-transaminases. Appl. Microbiol. Biotechnol. 2012;94:1163–1171.10.1007/s00253-012-4103-3
- Höhne M, Bornscheuer UT. Biocatalytic routes to optically active amines. ChemCatChem. 2009;1:42–51.10.1002/cctc.v1:1
- Shin JS, Kim BG. Kinetic modeling of ω-transamination for enzymatic kinetic resolution of a-methylbenzylamine. Biotechnol. Bioeng. 1998;60:534–540.10.1002/(ISSN)1097-0290
- Koszelewski D, Tauber K, Faber K, Kroutil W. ω-Transaminases for the synthesis of non-racemic α-chiral primary amines. Trends Biotechnol. 2010;28:324–332.10.1016/j.tibtech.2010.03.003
- Mutti FG, Fuchs CS, Pressnitz D, Sattler JH, Kroutil W. Stereoselectivity of four (R)-selective transaminases for the asymmetric amination of ketones. Adv. Synth. Catal. 2011;353:3227–3233.10.1002/adsc.v353.17
- Koszelewski D, Lavandera I, Clay D, Rozzell D, Kroutil W. Asymmetric synthesis of optically pure pharmacologically relevant amines employing ω-transaminases. Adv. Synth. Catal. 2008;350:2761–2766.10.1002/adsc.200800496
- Höhne M, Kuhl S, Karen R, Bornscheuer UT. Efficient asymmetric synthesis of chiral amines by combining transaminase and pyruvate decarboxylase. ChemBioChem. 2008;9:363–365.10.1002/(ISSN)1439-7633
- Koszelewski D, Lavandera I, Clay D, Guebitz GM, Rozzell D, Kroutil W. Formal asymmetric biocatalytic reductive amination. Angew. Chem. Int. Ed. 2008;47:9337–9340.10.1002/anie.v47:48
- Malik MS, Park ES, Shin JS. ω-Transaminase-catalyzed kinetic resolution of chiral amines using L-threonine as an amino acceptor precursor. Green Chem. 2012;14:2137–2140.10.1039/c2gc35615e
- Park E-S, Malik MS, Dong J-Y, Shin J-S. One-pot production of enantiopure alkylamines and arylalkylamines of opposite chirality catalyzed by ω-transaminase. ChemCatChem. 2013;5:1734–1738.10.1002/cctc.v5.7