Abstract
A chemolithoautotrophic arsenite-oxidizing bacterium, designated strain KGO-5, was isolated from arsenic-contaminated industrial soil. Strain KGO-5 was phylogenetically closely related with Sinorhizobium meliloti with 16S rRNA gene similarity of more than 99%, and oxidized 5 mM arsenite under autotrophic condition within 60 h with a doubling time of 3.0 h. Additions of 0.01–0.1% yeast extract enhanced the growth significantly, and the strain still oxidized arsenite efficiently with much lower doubling times of approximately 1.0 h. Arsenite-oxidizing capacities (11.2–54.1 μmol h−1 mg dry cells−1) as well as arsenite oxidase (Aio) activities (1.76–10.0 mU mg protein−1) were found in the cells grown with arsenite, but neither could be detected in the cells grown without arsenite. Strain KGO-5 possessed putative aioA gene, which is closely related with AioA of Ensifer adhaerens. These results suggest that strain KGO-5 is a facultative chemolithoautotrophic arsenite oxidizer, and its Aio is induced by arsenic.
Graphical Abstract
Sinorhizobium sp. KGO-5 is a chemolithoautotrophic arsenite-oxidizing bacterium, and can grow on 5 to 15 mM arsenite as the sole electron donor.

Arsenic is one of toxic metalloids and widely distributed in the environment with a crustal abundance of 0.0001%.Citation1) Although arsenic has four oxidation states (V, III, 0, –III), inorganic arsenate [As(V)] and arsenite [As(III)] predominate in aquatic and soil environments.Citation2) Arsenate exists in anionic forms ( and
) at circumneutral pH, and is strongly adsorbed to the surface of common soil minerals such as Fe and Al (hydr)oxides. In contrast, arsenite occurs mainly as a non-ionic form (H3AsO3, pKa = 9.22), and is therefore less adsorptive and more mobile than arsenate.Citation3,4) While arsenate is stable under oxic conditions, arsenite is stable under reducing conditions and is much more toxic than arsenate by a factor of 100.Citation5) The primary mechanism of arsenite toxicity is its binding to protein sulfhydryl groups. On the other hand, arsenate inhibits the oxidative phosphorylation due to its chemical similarity with phosphate.
Contamination of groundwater occurs in many areas of the world including Bangladesh, India, Vietnam, Thailand, Chile, China, and USA.Citation6) Among these, the most serious groundwater arsenic problem exists in Bangladesh and West Bengal, India, where approximately 60–100 million people are exposed to more than 10 μg L−1 of arsenic in drinking water, a health standard set by the World Health Organization and US Environmental Protection Agency.Citation6,7) In removal of arsenic from contaminated water, chemical oxidants such as chlorine, hypochlorite, ozone, and hydrogen peroxide are commonly used in pre-oxidation steps to convert arsenite to arsenate.Citation8) Subsequent remediation steps are carried out using adsorbents such as activated carbon and aluminum oxide, or co-precipitation with iron oxides.Citation9) However, these chemical oxidants are expensive and may produce harmful by-products such as trihalomethanes. Therefore, microbial transformation of arsenite to arsenate has gained significant attention, and could be a cost-effective alternative to conventional methods.Citation10–12)
Arsenite-oxidizing bacteria distribute widely in nature and are phylogenetically diverse.Citation13–15) To date, numerous heterotrophic and chemolithoautotrophic arsenite oxidizers have been isolated from arsenic-rich and pristine environments.Citation16) Heterotrophic arsenite oxidation is generally considered a detoxification mechanism that converts arsenite into less toxic arsenate. In contrast, chemolithoautotrophic arsenite oxidizers utilize arsenite as the sole electron donor for growth coupled with reduction of oxygen or nitrate.Citation17,18) Both of these bacteria possess arsenite oxidase (Aio), which consists of two subunits: a large subunit (AioA) containing a molybdopterin center and a [3Fe-4S] cluster and a small subunit (AioB) containing a Rieske [2Fe-2S] cluster.Citation19,20) AioA is similar to the molybdenum-containing subunits in the DMSO reductase family and distantly related to the catalytic subunit of respiratory arsenate reductase (ArrA).
The aim of this study was to isolate a new chemolithoautotrophic arsenite-oxidizing bacterium from arsenic-contaminated industrial soil in Japan, and to characterize its arsenite-oxidizing ability. The isolated strain, strain KGO-5, was phylogenetically closely related with Sinorhizobium meliloti in the class Alphaproteobacteria, and oxidized arsenite either in the presence or absence of organic matter. In addition, its arsenite-oxidizing ability as well as arsenite oxidase activity was found to be inducible. Due to its rapid growth in the presence of arsenite, strain KGO-5 could be one of promising micro-organisms applicable to biological oxidation of arsenite in mitigation of arsenic contamination in drinking water.
Materials and methods
Enrichment and isolation
Arsenic-contaminated soil was collected from a site formerly used as a chemical plant in Japan.Citation21) It contained approximately 13,000 μmol kg−1 (1,000 mg kg−1) of arsenic. The enrichment culture was prepared by inoculating 0.3 g wet weight of the soil into 30 mL minimal medium. The mediumCitation17) contained the following (per liter): (NH4)2SO4 (1 g), KH2PO4 (0.5 g), KCl (0.05 g), Ca(NO3)2 (0.1 g), NaHCO3 (0.5 g), Na2SO4·10H2O (0.07 g), Na2SeO3·5H2O (0.017 mg), Na2WO4·2H2O (0.03 mg), trace mineral element solution (1 mL), vitamin solution (1 mL), and NaAsO2 (0.65 g). The medium was dispensed into 100 mL Erlenmeyer flasks and autoclaved. Final pH was 7.5–8.0. The enrichment was incubated at 30 °C with shaking at 200 rpm.
The concentrations of arsenate and arsenite in the culture supernatant were determined by high-performance liquid chromatography (HPLC; L-7000; Hitachi, Tokyo, Japan) with an Aminex HPX-87H ion-exclusion column (Bio-Rad Laboratories, Hercules, CA, USA) as described previously.Citation22) After complete oxidation of arsenite was confirmed, 1 mL of the enrichment was transferred to 29 mL fresh liquid medium. After several rounds of subculturing, the enrichment was serially diluted and inoculated on an agar medium prepared with the minimal medium and 2% Bacto Agar (Difco, Sparks, MD, USA). After incubation at 30 °C, a single colony was picked, spread on new agar medium, and the resulting single colony was picked again to insure purity. The pure culture was then grown aerobically with arsenite in liquid medium.
Growth experiments
Strain KGO-5 was grown autotrophically in the minimal medium containing 5 mM arsenite at 30 °C with shaking at 200 rpm. NaHCO3 included in the medium was the sole carbon source under this condition. Effect of organic matter on the growth and arsenite oxidation by strain KGO-5 was tested by adding 0.01–0.1% of yeast extract (Becton Dickinson, Franklin Lakes, NJ, USA) to the minimal medium. Doubling times were calculated during the exponential phase of growth. Rates of arsenite oxidation (μM h−1) were calculated by dividing the arsenite concentrations tested by time required for complete oxidation of arsenite. When potential electron donors were tested, strain KGO-5 was cultured autotrophically, and the electron donors being tested were added to the minimal medium at 5 mM. When potential carbon sources were tested, the strain was cultured without arsenite, and the carbon sources being tested were added to the minimal medium at 5 g L−1.
Arsenite oxidation by washed cell suspension
Cells grown autotrophically for 3 days or heterotrophically with yeast extract for 2 days were collected by centrifugation (7,000 × g at 4 °C for 10 min). After washing twice with 0.8% NaCl, the cells were resuspended in 50 mM Tris–HCl buffer (pH 7.0) to achieve optical density at 600 nm (OD600) of approximately 0.04–0.36 (equivalent to 0.013–0.12 mg [dry weight] mL−1). After arsenite (5 mM) was added to the suspension, the cells were incubated aerobically at 30 °C with shaking at 200 rpm. The cell suspension was removed at various time intervals, and the concentrations of arsenate and arsenite were determined by HPLC. In all cases, arsenite oxidation proceeded linearly with time, and the incubation was completed within 6 h.
Arsenite oxidase assay
For the preparation of the crude extracts, cells grown for 2–3 days (until early stationary phase) were collected, washed, and resuspended in 50 mM Tris–HCl buffer (pH 7.0) to achieve OD600 of 10. They were disrupted by sonication (Ohtake ultrasonic disintegrator 5202) at 150 W and 100 kHz for 2 min followed by centrifugation (10,000 × g, 10 min, 4 °C) to remove cell debris. Arsenite oxidase activity in the crude extracts was assayed spectrophotometrically in a quartz cuvette at 30 °C, by monitoring the reduction of 2,6-dichloroindophenol sodium n-hydrate (DCIP, Wako Pure Chemical Industries) as an electron acceptor.Citation23) The molar extinction coefficient (mM−1 cm−1) for DCIP is 40.1 at 600 nm. The reaction mixture (1.5 mL) contained 50 mM MES [2-(N-morpholino)ethanesulfonic acid] buffer (pH 6.0), 0.06 mM DCIP, 2.5 mM arsenite, and an appropriate amount of enzyme. One unit (U) of arsenite oxidase was defined as the amount of enzyme required to reduce 1 μmol of DCIP per minute. All measurements were corrected for arsenite-independent reduction of DCIP by subtracting the rates observed for control samples in which arsenite was omitted from the reaction mixture. In the absence of enzyme, no DCIP reduction was observed. Protein concentration was determined by the method of BradfordCitation24) with bovine serum albumin as a standard protein.
Sequencing and phylogenetic analysis of 16S rRNA gene
Genomic DNA of strain KGO-5 was isolated as described previously.Citation25) The 16S rRNA gene was amplified by PCR using the bacterial consensus primers 8F (5′-AGAGTTTGATCCTGGCTCAG-3′, Escherichia coli positions 8–27) and 1491R (5′-GGTTACCTTGTTACGACTT-3′, Escherichia coli positions 1509–1491). PCR products were purified using a QIAquick PCR Purification kit (Qiagen, Hilden, Germany), and sequenced using a BigDye Terminator Cycle Sequencing kit (Applied Biosystems, Foster City, CA, USA) and an ABI Prism 3100 Genetic Analyzer (Applied Biosystems) using appropriate sequencing primers.Citation26) The obtained 16S rRNA gene sequences were subjected to a blast search (http://www.ncbi.nlm.nih.gov/BLAST/) to determine sequence similarity. The retrieved sequences were aligned using the Clustal X program, version 2.0. The phylogenetic tree was constructed using the neighbor-joining method.Citation27) Bootstrap values were obtained for 1,000 replicates to estimate the confidence of tree topologies.
Amplification of putative aioA gene
A putative arsenite oxidase gene (aioA) was amplified using degenerate primers aoxBM1–2F and aoxBM3–2R according to the PCR protocol described by Quéméneur et al.Citation28) The amplified product was confirmed on 2% agarose gels by electrophoresis, purified with a QIAquick PCR Purification kit (Qiagen), and sequenced. The obtained gene sequence was translated to amino acid sequence, and phylogenetic analysis was carried out as described above.
PCR-DGGE
DNA was extracted from the enrichment using a FastDNA Spin kit (MP Biomedicals, Morgan Irvine, CA, USA) according to the manufacturer’s instructions. PCR-DGGE was performed according to the method reported by Muyzer et al.Citation29) using the primers 341fGC and 534r. The PCR protocol used was as follows: (1) initial denaturation at 94 °C for 10 min; (2) 35 cycles of (a) denaturation at 94 °C for 15 s, (b) annealing at 55 °C for 45 s, and (c) extension at 72 °C for 30 s; and (3) final extension at 72 °C for 30 min. DGGE was performed using a DCode Universal Mutation Detection System (Bio-Rad Laboratories) as described previously.Citation30) The major bands were excised and used for reamplification with the primers 341f and 534r; the products obtained were sequenced as described previously.Citation30)
Nucleotide sequence accession number
The gene sequences identified in this study have been deposited in the DDBJ/EMBL/GenBank databases under accession numbers of AB915360 through AB915365.
Results
Isolation of a chemolithoautotrophic arsenite-oxidizing bacterium strain KGO-5
Arsenic-contaminated soil was inoculated with a minimal medium containing arsenite and bicarbonate as the sole electron donor and carbon source, respectively. When the soil slurry was incubated aerobically with shaking, 5 mM arsenite disappeared completely within 7 days, and approximately 4 mM arsenate was produced (Fig. S1). If the soil slurry was autoclaved at 121 °C for 2 h before the incubation, no arsenite was oxidized. The slurry was then subcultured repeatedly into the fresh medium containing 5 mM arsenite. After three rounds of subculturing, the microbial community in the enrichment was analyzed by PCR-DGGE targeting the 16S rRNA gene. As shown in Fig. S2 and Table S1, bacteria closely related to Lysobacter sp., Bacillus sp., and Sinorhizobium sp. were predominant in the enrichment.
The arsenite-oxidizing enrichment culture was serially diluted and inoculated into the minimal medium solidified with agar. After incubation for a few days, white bacterial colonies appeared, and they were purified by repeated inoculation and picking the single colony from the agar medium. Finally, the resulting colonies were inoculated into aerobic liquid medium containing 5 mM arsenite. After complete oxidation of arsenite was confirmed, one of the micro-organisms with the highest arsenite-oxidizing ability (complete oxidation of 5 mM arsenite within 2–3 days) was designated strain KGO-5. Cells of strain KGO-5 were straight rods, and Gram-stain reaction was negative. Spores formation was not observed.
Phylogenetic analysis of the 16S rRNA gene
blast and similarity analysis of the 16S rRNA gene sequence showed that strain KGO-5 was most similar to known strains of Sinorhizobium spp. in the class Alphaproteobacteria with sequence similarities of more than 99%. Phylogenetic comparison of the 16S rRNA gene sequence of strain KGO-5 with those of selected representatives was performed using approximately 1,450 bases (Fig. ). The result indicated that strain KGO-5 is most closely related to Sinorhizobium meliloti, and is distinct from known alphaproteobacterial arsenite oxidizers. The 16S rRNA gene sequence of the band d (Fig. S2) determined in PCR-DGGE analysis showed 100% identity with that of strain KGO-5.
Fig. 1. Phylogenetic tree showing the relationship between strain KGO-5 and related bacteria within the Alphaproteobacteria on the basis of 16S rRNA gene sequences.
Notes: The tree was constructed using the neighbor-joining method. Circles and triangles at the branch nodes represent bootstrap percentages (1,000 replicates): filled circles, 90–100%; open circles, 70–89%; open triangles, 50–69%. Values < 50% are not shown. The scale bar represents the estimated number of substitutions per site. The GenBank accession number for each reference strain is shown in parentheses. An asterisk represents the bacterium is a chemolithoautotrophic arsenite oxidizer, including a facultative chemolithoautotrophic arsenite oxidizer. Double asterisks represent the bacterium is a heterotrophic arsenite oxidizer.
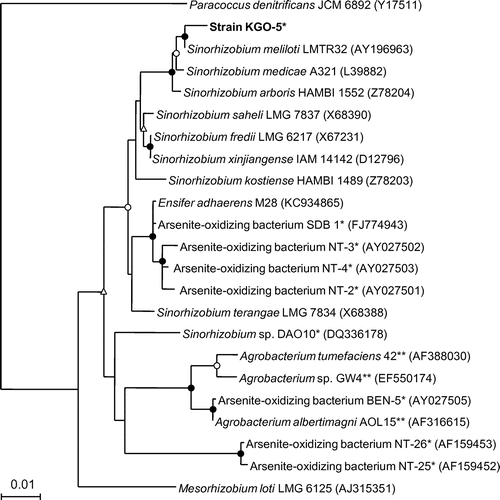
Arsenite oxidation under chemolithoautotrophic conditions
Strain KGO-5 grew by the oxidation of arsenite with the concomitant production of arsenate in the minimal medium (Fig. ). In the presence of 5 mM arsenite, a doubling time of 3.0 h was observed in the logarithmic growth phase (Fig. (A)). In addition, based on the fact that 4.7 mM arsenite was oxidized completely within 60 h, an arsenite oxidation rate of 78 μM h−1 was calculated. The ratios of arsenite consumed to arsenate produced were nearly 1.0 throughout the growth. If arsenite was omitted from the medium, no growth was observed (data not shown). These results indicated that strain KGO-5 is able to grow autotrophically using arsenite as the sole electron donor. In the presence of more than 5 mM of arsenite (10–15 mM), much longer doubling times (7.0–13 h) and much higher oxidation rates (110–113 μM h−1) were observed (Fig. (B) and (C)), but the growth was inhibited completely in the presence of 20 mM arsenite (Fig. (D)). The strain could not utilize 5 mM each of sulfide, elemental sulfur, thiosulfate, ammonium, nitrite, selenite, Fe(II), and Mn(II) as the sole electron donor for autotrophic growth. In addition, the strain did not grow anaerobically in the presence of arsenite and nitrate (5 mM) as the electron donor and the electron acceptor, respectively.
Arsenite oxidation under heterotrophic conditions
Strain KGO-5 was grown in the minimum medium supplemented with various organic matters. The strain utilized 5 g L−1 each of succinate, malate, fumarate, glutamate, and yeast extract as the energy and carbon sources, and the best growth was observed with yeast extract (OD660 of 1.3 at 72 h). On the other hand, the strain could not utilize glucose, fructose, galactose, sucrose, alanine, lactate, citrate, and acetate (OD660 of less than 0.002 at 144 h). In the presence of both arsenite (5 mM) and yeast extract (0.01–0.1%), the strain still oxidized arsenite (Fig. ). Based on the facts that approximately 5 mM of arsenite was oxidized completely within 36 h, the oxidation rates under these conditions were calculated to be 135 μM h−1. In the presence of 0.01 and 0.1% of yeast extract, the doubling times reduced to 0.75 and 1.1 h, respectively. Metabolic energy would not be gained from arsenite oxidation during growth with yeast extract, since the final growth of strain KGO-5 in the presence and absence of arsenite was not different (data not shown). These results indicated that strain KGO-5 is a facultatively chemolithoautotrophic arsenite-oxidizing bacterium, and can oxidize arsenite efficiently even in the presence of organic matter.
Arsenite-oxidizing ability by washed cells of strain KGO-5
Cells grown with arsenite under the autotrophic condition showed the arsenite-oxidizing ability of 54.1 μmol h−1 mg dry cells−1. Similar result (52.5 μmol h−1 mg dry cells−1) was obtained with the cells grown with arsenite and 0.01% yeast extract. As shown in Fig. , the cells grown with arsenite and 0.1% yeast extract showed the decreased ability of 11.2 μmol h−1 mg dry cells−1, and the cells grown without arsenite (but with 0.1% of yeast extract) did not show significant arsenite-oxidizing ability. Autoclaving of the cells completely inhibited their arsenite-oxidizing ability (Fig. ).
Fig. 4. Arsenite oxidation by washed cells of strain KGO-5.
Notes: Cells were grown with 5 mM arsenite and 0.1% yeast extract (filled symbols), or only with 0.1% yeast extract (cross symbols). Part of the former cells was autoclaved before the incubation (open symbols). Squares; arsenite, circles; arsenate. Approximately 2.3 mg dry cells were used in the experiment.
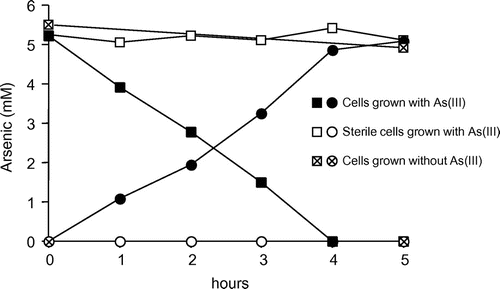
Arsenite oxidase assay
The crude extracts prepared from the cells grown with arsenite under the autotrophic condition showed a low but significant arsenite oxidase activity of 8.77 mU mg protein−1. The cells grown with arsenite and yeast extract were also tested for arsenite oxidase assay, and they were 10.0 (with 0.01% of yeast extract) and 1.76 (with 0.1% of yeast extract) mU mg protein−1. However, cells grown without arsenite (but with 0.1% of yeast extract) did not show significant arsenite oxidase activity.
Analysis of AioA sequence
Putative arsenite oxidase gene (aioA) was amplified from genomic DNA of strain KGO-5. The method of Quéméneur et al.Citation28) yielded a PCR product which shared 91% amino acid sequence similarity with the AioA of Ensifer adhaerens (Fig. ).
Fig. 5. Phylogenetic tree of putative AioA of strain KGO-5.
The tree was constructed based on approximately 300 amino acid sequences using the neighbor-joining method. Circles and triangles at the branch nodes represent bootstrap percentages (1,000 replicates): filled circles, 90–100%; open circles, 70–89%; open triangles, 50–69%. Values < 50% are not shown. The scale bar represents the estimated number of substitutions per site. The GenBank accession number for each reference strain is shown in parentheses.
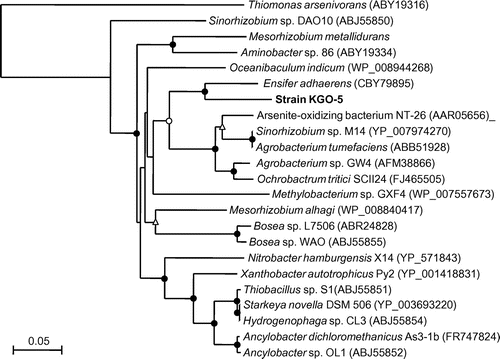
Discussion
To date, a wide variety of arsenite-oxidizing bacteria, including both chemolithoautotrophic and heterotrophic arsenite oxidizers, have been isolated.Citation16) They are phylogenetically diverse and mainly include members of Proteobacteria, Bacteroidetes, Deinococcus-Thermus, Chlorobi, Chloroflexi, and Aquificae. Among these, strains belonging to the Agrobacterium/Rhizobium branch in the class Alphaproteobacteria have frequently been isolated (Fig. ). Most of these strains are facultative chemolithoautotrophic arsenite oxidizers, and were isolated from arsenic-contaminated environments such as gold mine,Citation17,31–33) hot creek,Citation34) and sediment.Citation35) In this study, we have newly isolated a facultative arsenite-oxidizing bacterium strain KGO-5 from arsenic-contaminated industrial soil, and it was phylogenetically closely related with Sinorhizobium meliloti with 16S rRNA gene sequence similarity of 99.3%. Although arsenite-oxidizing bacteria related with Sinorhizobium spp. including strains M14,Citation32) SDB1,Citation33) DAO10,Citation18) NT-2,Citation31) NT-3,Citation31) NT-4,Citation31) and NT-26Citation17) have been reported so far, phylogenetic analysis indicate that strain KGO-5 is distantly related with these strains (Fig. ). Actually, these arsenite-oxidizing strains showed only 94–98% sequence similarities with Sinorhizobium meliloti as well as with strain KGO-5. In addition, strain KGO-5 utilized only limited number of carbon sources under heterotrophic growth conditions, and they were significantly different from those utilized by strains NT-26, DAO10, and SDB1.Citation17,18,33) For example, strain KGO-5 was not capable of utilizing glucose as a sole carbon source, whereas all of other strains can utilize it. Therefore, it is considered that strain KGO-5 is a new arsenite-oxidizing bacterium, which is a member of the genus Sinorhizobium.
Strain KGO-5 grew rapidly under chemolithoautotrophic condition with a doubling time of 3.0 h (Fig. (A)), and much shorter doubling times (0.75–1.1 h) were observed in the presence of yeast extract (Fig. ). Santini et al.Citation17) reported that doubling times of strain NT-26 under chemolithoautotrophic and heterotrophic conditions were 7.6 h and 2.4–3.4 h, respectively. Similarly, strain M14 showed doubling times of 9.0 h and 2.2–3.5 h under chemolithoautotrophic and heterotrophic conditions, respectively.Citation32) Furthermore, strain SDB1 was reported to have doubling times of 9.8 h under chemolithoautotrophic condition and 2.1–3.4 h under heterotrophic condition.Citation33) Since all of these studies were conducted at an arsenite concentration of 5 mM, it seems likely that growth of strain KGO-5 in the presence of arsenite is much faster than that of other arsenite-oxidizing bacteria. In addition, strain KGO-5 showed relatively high arsenite oxidation rates of 78–135 μM h−1 (Figs. and ) Although certain arsenite-oxidizing bacteria have very high oxidation rates of more than 400 μM h−1, common oxidation rates are between 10 and 50 μM h−1.Citation36) These results, together with the fact that it can tolerate up to 15 mM of arsenite, suggest that strain KGO-5 possesses several physiological characteristics that are suitable for its potential application as a biological oxidant in removal of arsenic from contaminated water.
Arsenite-oxidizing ability as well as arsenite oxidase enzyme activity was inducible in strain KGO-5 (Fig. ). Similar results have been observed in other arsenite-oxidizing bacteria such as Alcaligenes faecalis,Citation37) Agrobacterium albertimagni AOL15,Citation34) Thiomonas sp. 3As,Citation38) and strain M14,Citation32) while constitutive expression of arsenite oxidase was reported in Polaromonas sp. GM1.Citation39) Despite its rapid oxidation rates of arsenite, specific activities of arsenite oxidase in the crude extracts of strain KGO-5 were very low (1.76–10.0 mU mg protein−1). This was probably due to inefficient electron transfer from arsenite to DCIP, as has been suggested in other arsenite oxidizers.Citation40) Further studies are required to understand the localization (membrane-bound or periplasmic) as well as expression mechanism of this enzyme in strain KGO-5.
In conclusion, a newly isolated strain KGO-5 is a facultative chemolithoautotrophic arsenite-oxidizing bacterium, which is closely related with Sinorhizobium meliloti. It can grow rapidly in the presence of arsenite, and efficiently oxidize arsenite not only under autotrophic condition but also under heterotrophic condition. Since arsenate is less toxic and much more easily adsorbed on various solid adsorbents than arsenite, strain KGO-5 could be used in the first step of arsenic bioremediation in contaminated water.
Supplemental material
The supplemental material for this paper is available at http://dx.doi.org/10.1080/09168451.2014.940276.
Supplementary Materials
Download Zip (160.4 KB)Funding
This work was supported by grants from the Ministry of Agriculture, Forestry and Fisheries of Japan (Research Project for Ensuring Food Safety from Farm to Table AC-1122) and from JSPS KAKENHI [grant number 23580103].
References
- Cullen WR, Reimer KJ. Arsenic speciation in the environment. Chem. Rev. 1989;89:713–764.10.1021/cr00094a002
- Smedley PL, Kinniburgh DG. A review of the source, behaviour and distribution of arsenic in natural waters. Appl. Geochem. 2002;17:517–568.10.1016/S0883-2927(02)00018-5
- Dixit S, Hering JG. Comparison of arsenic(V) and arsenic(III) sorption onto Iron oxide minerals: implications for arsenic mobility. Environ. Sci. Technol. 2003;37:4182–4189.10.1021/es030309t
- Goldberg S. Competitive adsorption of arsenate and arsenite on oxides and clay minerals. Soil Sci. Soc. Am. J. 2002;66:413–421.10.2136/sssaj2002.0413
- Rosen BP. Biochemistry of arsenic detoxification. FEBS Lett. 2002;529:86–92.10.1016/S0014-5793(02)03186-1
- Ng JC, Wang J, Shraim A. A global health problem caused by arsenic from natural sources. Chemosphere. 2003;52:1353–1359.10.1016/S0045-6535(03)00470-3
- Fendorf S, Michael HA, van Geen A. Spatial and temporal variations of groundwater arsenic in South and Southeast Asia. Science. 2010;328:1123–1127.10.1126/science.1172974
- Bissen M, Frimmel FH. Arsenic−a review. Part II: oxidation of arsenic and its removal in water treatment. Acta Hydrochim. Hydrobiol. 2003;31:97–107.10.1002/(ISSN)1521-401X
- Mondal P, Majumder CB, Mohanty B. Laboratory based approaches for arsenic remediation from contaminated water: recent developments. J. Hazard. Mater. 2006;137:464–479.10.1016/j.jhazmat.2006.02.023
- Bahar MM, Megharaj M, Naidu R. Arsenic bioremediation potential of a new arsenite-oxidizing bacterium Stenotrophomonas sp. MM-7 isolated from soil. Biodegradation. 2012;23:803–812.10.1007/s10532-012-9567-4
- Ike M, Miyazaki T, Yamamoto N, Sei K, Soda S. Removal of arsenic from groundwater by arsenite-oxidizing bacteria. Wat. Sci. Technol. 2008;58:1095–1100.10.2166/wst.2008.462
- Andrianisa HA, Ito A, Sasaki A, Aizawa J, Umita T. Biotransformation of arsenic species by activated sludge and removal of bio-oxidised arsenate from wastewater by coagulation with ferric chloride. Wat. Res. 2008;42:4809–4817.10.1016/j.watres.2008.08.027
- Oremland RS, Stolz JF. The ecology of arsenic. Science. 2003;300:939–944.10.1126/science.1081903
- Silver S, Phung LT. Genes and enzymes involved in bacterial oxidation and reduction of inorganic arsenic. Appl. Environ. Microbiol. 2005;71:599–608.10.1128/AEM.71.2.599-608.2005
- Stolz JF, Basu P, Oremland RS. Microbial arsenic metabolism: new twists on an old poison. Microbe. 2010;5:53–59.
- Yamamura S, Amachi S. Microbiology of inorganic arsenic: from metabolism to bioremediation. J. Biosci. Bioeng. 2014;118:1–9, http://dx.doi.org/10.1016/j.jbiosc.2013.12.011
- Santini JM, Sly LI, Schnagl RD, Macy JM, Wulf-Durand P, Macy JM. A new chemolithoautotrophic arsenite-oxidizing bacterium isolated from a gold mine: phylogenetic, physiological, and preliminary biochemical studies. Appl. Environ. Microbiol. 2000;66:92–97.10.1128/AEM.66.1.92-97.2000
- Rhine ED, Phelps CD, Young LY. Anaerobic arsenite oxidation by novel denitrifying isolates. Environ. Microbiol. 2006;8:899–908.10.1111/emi.2006.8.issue-5
- Ellis PJ, Conrads T, Hille R, Kuhn P. Crystal structure of the 100 kDa arsenite oxidase from alcaligenes faecalis in two crystal forms at 1.64 and 2.03 Å. Structure. 2001;9:125–132.10.1016/S0969-2126(01)00566-4
- JMSantini, Molybdenum-containing arsenite oxidase of the chemolithoautotrophic arsenite oxidizer NT-26. J. Bacteriol. 2004; 186: 1614–1619.10.1128/JB.186.6.1614-1619.2004
- Kudo K, Yamaguchi N, Makino T, Ohtsuka T, Kimura K, Dong DT, Amachi S. Release of arsenic from soil by a novel dissimilatory arsenate-reducing bacterium Anaeromyxobacter sp. strain PSR-1. Appl. Environ. Microbiol. 2013;79:4635–4642.10.1128/AEM.00693-13
- Ohtsuka T, Yamaguchi N, Makino T, Sakurai K, Kimura K, Kudo K, Homma E, Dong DT, Amachi S. Arsenic dissolution from Japanese paddy soil by a dissimilatory arsenate-reducing bacterium Geobacter sp. OR-1. Environ. Sci. Technol. 2013;47:6263–6271.
- Anderson GL, Williams J, Hille R. The purification and characterization of arsenite oxidase from Alcaligenes faecalis, a molybdenum-containing hydroxylase. J. Biol. Chem. 1992;267:23674–23682.
- Bradford MM. A rapid and sensitive method for the quantification of microgram quantities of protein utilizing the principle of protein-dye binding. Anal. Chem. 1976;72:248–254.
- Hiraishi A. Direct automated sequencing of 16S rDNA amplified by polymerase chain reaction from bacterial cultures without DNA purification. Lett. Appl. Microbiol. 1992;15:210–213.10.1111/j.1472-765X.1992.tb00765.x
- Weisburg WG, Barns SM, Pelletier DA, Lane DJ. 16S ribosomal DNA amplification for phylogenetic study. J. Bacteriol. 1991;173:697–703.
- Saito N, Nei M. The neighbor-joining method: a new method for reconstructing phylogenetic trees. Mol. Biol. Evol. 1987;4:406–425.
- Quemeneur M, Heinrich-Salmeron A, Muller D, Lievremont D, Jauzein M, Bertin PN, Garrido F, Joulian C. Diversity surveys and evolutionary relationships of aoxB genes in aerobic arsenite-oxidizing bacteria. Appl. Environ. Microbiol. 2008;74:4567–4573.10.1128/AEM.02851-07
- Muyzer G, de Waal EC, Uitterlinden AG. Profiling of complex microbial populations by denaturing gradient gel electrophoresis analysis of polymerase chain reaction-amplified genes coding for 16S rRNA. Appl. Environ. Microbiol. 1993;59:695–700.
- Suda W, Oto M, Amachi S, Shinoyama H, Shishido M. A direct method to isolate DNA from phyllosphere microbial communities without disrupting leaf tissues. Microbes Environ. 2008;23:248–252.10.1264/jsme2.23.248
- Santini JM, Sly LL, Wen A, Comrie D, Wulf-Durand PD, Macy JM. New arsenite-oxidizing bacteria isolated from Australian gold mining environments–phylogenetic relationships. Geomicrobiol. J. 2002;19:67–76.10.1080/014904502317246174
- Drewniak L, Matlakowska R, Sklodowska A. Arsenite and arsenate metabolism of Sinorhizobium sp. M14 living in the extreme environment of the Zloty Stok gold mine. Geomicrobiol. J. 2008;25:363–370.10.1080/01490450802402836
- Lugtu RT, Choi SC, Oh YS. Arsenite oxidation by a facultative chemolithotrophic bacterium SDB1 isolated from mine tailing. J. Microbiol. 2009;47:686–692.10.1007/s12275-009-0279-3
- Salmassi TM, Venkateswaren K, Satomi M, Newman DK, Hering JG, Hering JG. Oxidation of arsenite by Agrobacterium albertimagni, AOL15, sp. nov., isolated from Hot Creek, California. Geomicrobiol. J. 2002;19:53–66.10.1080/014904502317246165
- Fan H, Su C, Wang Y, Yao J, Zhao K, Wang Y, Wang G. Sedimentary arsenite-oxidizing and arsenate-reducing bacteria associated with high arsenic groundwater from Shanyin, Northwestern China. J. Appl. Microbiol. 2008;105:529–539.10.1111/jam.2008.105.issue-2
- Bachate SP, Khapare RM, Kodam KM. Oxidation of arsenite by two β-proteobacteria isolated from soil. Appl. Microbiol. Biotechnol. 2012;93:2135–2145.10.1007/s00253-011-3606-7
- Philips SE, Taylor ML. Oxidation of arsenite to arsenate by Alcaligenes faecalis. Appl. Environ. Microbiol. 1976;32:392–399.
- Duquesne K, Lieutaud A, Ratouchniak J, Muller D, Lett MC, Bonnefoy V. Arsenite oxidation by a chemoautotrophic moderately acidophilic Thiomonas sp.: from the strain isolation to the gene study. Environ. Microbiol. 2008;10:228–237.
- Osborne TH, Jamieson HE, Hudson-Edwards KA, Nordstrom DK, Walker SR, Ward SA, Santini JM. Microbial oxidation of arsenite in a subarctic environment: diversity of arsenite oxidase genes and identification of a psychrotolerant arsenite oxidiser. BMC Microbiol. 2010;10:205.10.1186/1471-2180-10-205
- vanden Hoven RN, Santini JM. Arsenite oxidation by the heterotroph Hydrogenophaga sp. str. NT-14: the arsenite oxidase and its physiological electron acceptor. Biochim. Biophys. Acta. 2004;1656:148–155.