Abstract
Taxol was originally isolated from the yew Taxus brevifolia. Because taxol inhibits the depolymerization of microtubules, the presence of a self-resistance mechanism in Taxus spp. was hypothesized. The cloning of the cDNA for alpha and beta tubulins from Taxus cuspidata and those from the human embryonic kidney cell line HEK293T revealed that the 26Asp, 359Arg, and 361Leu residues in the human beta tubulin, which are important for taxol binding, were replaced with Glu, Trp, and Met in the beta tubulin of T. cuspidata, respectively. The microtubule assembly of the recombinant alpha and beta tubulins was monitored turbidimetrically, and the results clearly demonstrated that the microtubule from T. cuspidata is less sensitive to taxol than that from HEK293T cells. The Taxus microtubule composed of the wild-type alpha tubulin and the beta tubulin with the E26D mutation restored the sensitivity to taxol. We thus postulated that the mutation identified in the beta tubulin of T. cuspidata plays a role in the self-resistance of this species against taxol.
Graphical Abstract
Monitoring of microtubule assembly in the absence and presence of taxol suggests that the beta tubulin of T. cuspidata is tolerant of taxol.
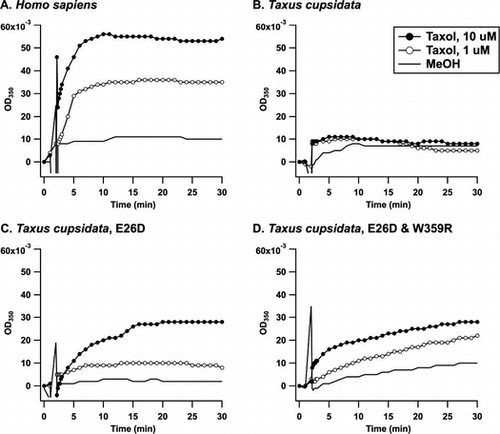
Key words:
Natural products have fascinated research scientists in chemistry and biology due to their complicated structures and diverse biological activities, and these products have often become the lead compounds for clinical application. Due to issues of toxicity and specificity, the elaborative tuning of the structure of a lead compound is required for its approval as a medical agent. Taxol (Fig. ) could be one of the exceptional cases. This compound was isolated from the yew Taxus brevifoliaCitation1) and has significant activity against ovarian, breast, and lung carcinomas.Citation2) Taxol specifically suppresses the depolymerization of microtubulesCitation3) in cancer cells and tumors and inhibits cell proliferation by causing apoptosis.Citation4) As is often the case, however, patients encounter the appearance of taxol-resistant cells.Citation5) This resistance is partly acquired by the expression of P-glycoprotein, which effluxes the taxol from the cell, the overexpression of different tubulin isotypes, or alterations in the structure of tubulins. The last case may be explained by a reduction in the affinity to taxolCitation6,7) or interference in microtubule dynamics and stability.
A microtubule consists of alpha and beta tubulins. Heterodimers of alpha and beta tubulins are accumulated to build a tube-shaped quaternary structure. The interaction of taxol with microtubules has been studied in detail using animal tubulins. Animal brains are rich in microtubules, which enables the simple and rapid purification of microtubules.Citation8) Photolabeling,Citation9,10) H/D exchange coupled to LC/MS,Citation11) and electron crytallographyCitation12) have shown that taxol binds to beta tubulin. Modeling studies based on the crystal structure of the taxol and beta tubulin complex projected the interaction site of taxol.Citation13,14) The 23Val in the H1 helix forms a hydrophobic interaction with both of the N′ and 3′ phenyl rings, and 26Asp is positioned within hydrogen bonding distance from the nitrogen group in the side chain. In the H6–H7 loop, 215Leu and 217Leu form hydrophobic interactions with the 2-phenyl ring, which is located near 227His and 228Leu in helix H7. In the same helix, 231Ala and 234Ser interact with the 3′-phenyl group. The hydrophobic environment of the 3′ phenyl group is also close to 270Phe at the end of the B7 strand. The M-loop, which consists of 272Pro, 273Leu, 274Thr, 275Ser, and 276Arg, is essential for binding of the taxane ring. This pocket is in close proximity to residues in the B9–B10 loop, including 358Pro, 359Arg, 360Gly, and 361Leu.
Taxol was not only isolated from the yew T. brevifoliaCitation1) but also other Taxus spp. and callus prepared from Taxus spp.Citation15) However, the real producer of taxol is considered to be an endophytic fungus.Citation16,17) It is likely that the yew may be tolerant to taxol, as is observed in cancer cell lines and tumors. Although Expósito reported that the viability of Taxus cells is suppressed in the presence of a high concentration of exogenous taxol,Citation18) the tolerance of Taxus spp. against taxol has not been comprehended at a molecular level. In fact, the investigation of the interaction of the natural products with plant microtubules has not exhibited comparable progress to that of the interaction of taxol with animal tubulins. Due to the extremely low concentration of microtubules in plants, the same purification methodCitation8) was not applicable. Some references have demonstrated the purification of plant tubulins by a combination of chromatography.Citation19–21) Using this combined method, however, the Taxus tubulins could not be purified in our laboratory (data not shown). Alternatively, recombinant plant tubulins were demonstrated to be required for taxol-assisted microtubule formation,Citation22,23) although Taxus tubulins have not yet been expressed in vitro.
In this study, we cloned and expressed in vitro the alpha and beta tubulins from Taxus cuspidata and human embryonic kidney cells (HEK293T) and identified specific variations in the beta tubulin from T. cuspidata. We subsequently investigated the effect of taxol on microtubule assembly and proposed that the identified variations may have reduced the sensitivity to taxol.
Materials and methods
Alignment of plant tubulin sequences to deduce the consensus sequences
The amino acid sequences of plant alpha or alpha-1 tubulins from Homo sapiens (NP_006073.2), T. cuspidata (cloned in this study), Taxus baccata (WWSSA2),Citation24) Arabidopsis thaliana (NP_176654.1), Camellia sinensis (ABC59068.2), Daucus carota (AAG02564.1), Eleusine indica (CAA06619.1), Glycine max (NP_001237301), Hirschfeldia incana (ADW66593.1), Hordeum vulgare subsp. vulgare (AAB08791.1), Medicago truncatula (XP_003624633), Miscanthus sinensis (AFC88823.1), Oryza sativa (ADM86870.1), Populus tremuloides (AAO63781.1), and Triticum aestivum (AAD10486.1) were aligned using Clustal X (Fig. S1). The consensus amino acid sequences were determined to be 20C-W-E-L-Y-C-L-27E and 413M-E-E-G-E-F-S-420E.
The amino acid sequences of plant beta or beta-1 tubulins from H. sapiens (NP_821133.1), T. cuspidata (cloned in this study), T. baccata (WWSSB1),Citation24) A. thaliana (BAB10059.1), Eucalyptus grandis (ABS50665.1), G. max (NP_001239638), Gossypium hirsutum (AF487511_1), Lupinus albus (CAA49736), O. sativa (AAA66495.1), T. aestivum (AAD10487.1), and Vanilla planifolia (ADZ76155.1) were aligned using Clustal X (Fig. S2). The consensus amino acid sequences were determined to be 19K-F-W-E-V-(V/I)-25C and 403M-D-E-M-E-F-T-410E.
Extraction of total RNA from T. cuspidata
Unless otherwise noted, the following procedure was performed at 4 °C. Twenty-five leaves of T. cuspidata were smashed in liquid nitrogen and treated with 5.0 mL of Isogen (Nippon Gene Co., Ltd). The suspension was incubated at 50 °C and cooled to room temperature for 5 min, and 1.0 mL of CHCl3 was then added to the mixture. After vortexing, the mixture was maintained at room temperature for 2 min and partitioned by centrifugation at 10,000 g for 15 min. The aqueous layer (2.4 mL) was combined with 4.0 M LiCl, and the mixture was vortexed for 30 s, stored at −80 °C for 1 h, and centrifuged at 12,000 g for 15 min to yield a white pellet. The pellet was dissolved in 400 μL of sterile H2O, and 400 μL of isopropanol was then added to the suspension. The mixture was vortexed for 15 s, cooled on ice for 30 min, and centrifuged at 12,000 g for 15 min. The pellet was washed with 70% EtOH and dissolved in 400 μL of sterile H2O. The solution was treated with 40 μL of 3.0 M NaOAc and 1.0 mL of EtOH and then stored at −80 °C for 1 h. After centrifugation at 12,000 g for 15 min, a white pellet was obtained. The pellet was washed with 1.0 mL of 70% EtOH, dissolved in TE buffer, and stored at −80 °C until use. The OD260 and the ratio of OD260 to OD280 were measured to determine the quantity and purity, respectively.
Molecular cloning of alpha and beta tubulin cDNAs from T. cuspidata
The total RNA was extracted using Isogen (Nippon Gene Co., Ltd) and was reverse transcripted with an oligo dT primer CDS III/3′ PCR Primer (att cta gag gcc gag gcg gcc gac atg ttt ttt ttt ttt ttt ttt ttt ttt ttt ttt vn) using SMARTScribe™ Reverse Transcriptase (Takara Bio Inc.), and 1.0 μM aag cag tgg tat caa cgc aga gtg gcc att acg gcc ggg was included in the ligation reaction as the adaptor sequence to the 3′ end of the cDNA sequence. The first strand cDNA was amplified by a PCR reaction using the CDS III/3′ PCR Primer and 5′ PCR Primer (aag cag tgg tat caa cgc aga gt) as the primers and EmeraldAmp PCR Master Mix (Takara Bio Inc.) as the enzyme.
The T. cuspidata specific cDNA was amplified by PCR with the degenerated primers, which cover the consensus DNA sequences identified in the plant tubulin DNA. For the alpha tubulin of T. cuspidata, tgy tgg gar cty tay tgy ctc gar and ytc nga raa ytc hcc ytc ytc cat were used as the forward and reverse primers, respectively. The PCR conditions were 94 °C for 2 min followed by 35 cycles of 98 °C for 10 s, 55 °C for 30 s, and 72 °C for 90 s.
For the beta tubulin of T. cuspidata, aar ttc tgg gar gtk rts tgy and ytc ngt gaa ctc cat ytc rtc cat were used as the forward and reverse primers, respectively. The PCR conditions were 98 °C for 2 min followed by 35 cycles of 98 °C for 10 s, 58 °C for 30 s, and 72 °C for 90 s. The gel-purified PCR fragments were directly ligated to the pMD20-T vector for TA cloning using the Mighty TA-Cloning Kit (Takara Bio Inc.). Competent DH5α cells (Takara Bio Inc.) were transformed with the ligation reaction mixture and incubated overnight at 37 °C on a LB agar plate containing ampicillin (50 μg/mL). Blue–white selection using X-gal (25 μg/mL) enabled the identification of the positive clone, which was inoculated in LB medium containing ampicillin (50 μg/mL). The Pure YieldTM Plasmid MiniPrep System (Promega KK) was used to extract the plasmid, which was subjected to on-demand sequencing analysis at Fasmac Co., Ltd.
For 5′ RACE, step-down PCR was performed using KOD-Plus-Neo (Toyobo Co., Ltd) and the 5′ PCR Primer and gca gtt rtc tgc aag ctt cct gat acg atc as the primers for alpha tubulin and the 5′ PCR Primer and aag tgt cct gaa gca gat atc ata cag tgc as the primers for beta tubulin. For the alpha and beta tubulins of T. cuspidata, the PCR conditions were 94 °C for 2 min, five cycles of 98 °C for 10 s and 74 °C for 20 s, five cycles of 98 °C for 10 s and 72 °C for 20 s, five cycles of 98 °C for 10 s and 70 °C for 20 s, five cycles of 98 °C for 10 s and 68 °C for 20 s, and 68 °C for 7 min.
The nested PCR was performed using aga gtg gcc att acg gcc ggg and aac agt ggg ctc cag atc tac aaa tac agc for alpha tubulin and aga gtg gcc att acg gcc ggg and gga tac tcc tcc ctg atc ttc gat atc arc for beta tubulin. For the alpha and beta tubulins of T. cuspidata, the PCR conditions were 94 °C for 2 min followed by 35 cycles of 98 °C for 10 s, 65 °C for 5 s, and 72 °C for 20 s.
For 3′ RACE, step-down PCR was performed using KOD-Plus-Neo (Toyobo Co., Ltd) with the CDS III/3′ PCR and tgc cca agg atg tga atg as the primers for alpha tubulin and the 3′ PCR Primer (gcc gag gcg gcc gac atg) and tgt cca gaa caa gaa ttc as the primers for beta tubulin. For the alpha and beta tubulins of T. cuspidata, the PCR conditions were 94 °C for 2 min, five cycles of 98 °C for 10 s and 74 °C for 20 s, five cycles of 98 °C for 10 s and 72 °C for 20 s, five cycles of 98 °C for 10 s and 70 °C for 20 s, five cycles of 98 °C for 10 s and 68 °C for 20 s, and 68 °C for 7 min.
The first nested PCR was performed with the CDS III/3′ PCR Primer and tca aag ggc cgt gtg cat gat atc aaa ttc for alpha tubulin and act ttg ttg aat gga tcc and gcc gag gcg gcc gac atg for beta tubulin. For the alpha tubulin of T. cuspidata, the PCR conditions were 94 °C for 2 min followed by 25 cycles of 98 °C for 10 s, 65 °C for 5 s, and 72 °C for 20 s. For the beta tubulin of T. cuspidata, the PCR conditions were 94 °C for 2 min followed by 25 cycles of 98 °C for 10 s, 60 °C for 30 s, and 72 °C for 20 s.
The second nested PCR was performed with the CDS III/3′ PCR Primer and tca aag ggc cgt gtg cat gat atc aaa ttc for alpha tubulin and act ttg ttg aat gga tcc and gcc gag gcg gcc gac atg for beta tubulin. For the alpha tubulin of T. cuspidata, the PCR conditions were 94 °C for 2 min followed by 35 cycles of 98 °C for 10 s, 5 °C for 5 s, and 72 °C for 20 s. For the beta tubulin of T. cuspidata, the PCR conditions were 94 °C for 2 min followed by 25 cycles of 98 °C for 10 s, 60 °C for 30 s, and 72 °C for 20 s.
TA cloning was performed as follows. The gel-purified PCR fragments from 5′ RACE and 3′ RACE were incubated with 10X A-attachment (Toyobo Co., Ltd), dNTP mixture, MgSO4, and 10X PCR buffer for KOD-Plus-Neo (Toyobo Co., Ltd) at 60 °C for 30 min. The reaction product was ligated to the pMD20-T vector (Takara Bio Inc.) using Ligation Mighty Mix (Takara Bio Inc.) at 16 °C for 150 min. Competent DH5α cells were transformed with the ligation mixture at 4 °C for 30 min and 42 °C for 45 s. After the mixture was cooled to 4 °C, it was poured onto an LB agar plate containing ampicillin. After incubation at 37 °C overnight, the white-colored colonies were inoculated overnight in 2.0 mL of LB medium containing ampicillin. The following day, the plasmid was extracted using the PureYield™ Plasmid Miniprep System (Promega KK), and subjected to on-demand sequence analysis (Fasmac Co., Ltd).
For amplification of the whole cDNA sequences of the alpha and beta tubulins from T. cuspidata, PCR was performed with gct gga tcc atg aga gag tgc ata tcg and ttg cga ctc gag tca gta ttc gtc ccc ctc atc for the alpha tubulin of T. cuspidata and cgc gaa gct ttt atg cgt gaa atc ttg cac gtt and cgc ctc gag tta cat gcc tac atc tcc tga aac for the beta tubulin of T. cuspidata. The conditions were 94 °C for 2 min followed by 35 cycles of 98 °C for 10 s and 68 °C for 50 s. The gel-purified PCR fragments for alpha tubulin were digested at 37 °C for 3 h with BamH I and Xho I, and those for beta tubulin were digested with Hind III and Xho I; the resulting fragments were then ligated to the pBluescript II KS (+) vector (pBS) (Agilent Technologies, Inc.), which was previously cut using the same pair of the restriction enzymes, and the resulting vector was subjected to on-demand sequence analysis (Fasmac Co., Ltd). The pBS Taxus alpha tubulin and the pBS Taxus beta tubulin were digested with the same pair of the restriction enzyme and ligated to the pET28a vector.
Extraction of total RNA from HEK293T cells
Unless otherwise noted, the following procedure was performed at 4 °C. The human embryonic kidney cell line HEK293T (RCB2202, Cell Bank, Riken Bioresource Center) was maintained in RPMI1640 medium containing 10% FBS, 100 units/mL penicillin, and 100 μg/mL streptomycin at 37 °C under a 5% CO2/air atmosphere. The cells (6.5 × 106 cells) were harvested and suspended in 1.2 mL of Isogen (Nippon Gene Co., Ltd). The suspension was maintained at room temperature for 5 min and then at −80 °C for 30 min, and thawed at room temperature. Then, 0.2 mL of CHCl3 was added to the mixture and the suspension was mixed vigorously. After 3 min at room temperature, the mixture was centrifuged at 12,000 g for 15 min. The aqueous layer was mixed with 0.5 mL of 2-propanol for 5 min at room temperature and centrifuged at 12,000 g for 10 min. The pellet was washed with 70% EtOH, dried in air for 10 min, dissolved in 100 μL of TE buffer, and stored at −80 °C until use. The OD260 and the ratio of OD260 to OD280 were measured to determine quantity and purity of the total RNA, respectively.
Molecular cloning of alpha and beta tubulin cDNAs from HEK293T cells
The total RNA (5.6 μg) was reverse-transcripted with 1.0 μM CDS III/3′ PCR Primer using SMARTScribe™ Reverse Transcriptase (Takara Bio Inc.), and 1.0 μM aag cag tgg tat caa cgc aga gtg gcc att acg gcc ggg was included in the ligation reaction as the adaptor sequence to the 3′ end of the cDNA sequence.
The cDNA was amplified by PCR using cat gga tcc atg cgt gag tgc atc tcc and aga ccg ctc gag tta gta ttc ctc tcc ttc ttc as the forward and reverse primers for alpha tubulin (NP_006073.2), respectively, and ctg aat tca tga ggg aaa tcg tgc aca tcc agg and atg agt ctc gag tta ggc ctc ctc ttc ggc as the forward and reverse primers for beta tubulin (NP_821133.1), respectively. The PCR conditions were 94 °C for 2 min followed by 35 cycles of 98 °C for 10 s and 68 °C for 50 s.
The gel-purified PCR fragments of apha and beta tubulins were cut with Xho I and BamH and with Xho I and EcoR I, respectively, for 3 h at 37 °C, ligated to the pBluescript II KS (+) vector (pBS), which was previously cut with the same pair of restriction enzymes, and subjected to on-demand sequence analysis (Fasmac Co., Ltd). The pBS human alpha tubulin and pBS human beta tubulin were digested with the same pair of restriction enzymes and ligated to the pET28a vector.
Expression of the recombinant proteins
Competent BL21 (DE3) cells were transformed on an LB agar plate containing 15 μg/mL kanamycin with the pET28a vector carrying the cDNA from the Taxus alpha tubulin, Taxus beta tubulin, human alpha tubulin, or human beta tubulin. The bacterial colony was inoculated in 2.0 mL of LB medium containing 15 μg/mL kanamycin overnight at 37 °C. The bacterial culture was then transferred to a large volume of LB medium and incubated until the OD600 reached 0.4. Then, 0.2 mM IPTG was added to the bacterial culture, and the cells were incubated for 3 h and was centrifuged at 8000 g for 10 min. The pellet was resuspended in a buffer containing 300 mM NaCl and Tris/HCl (pH 8.0) and sonicated for 1 min at 15 s intervals. The suspension was centrifuged at 16,000 g for 10 min. The SDS-PAGE analysis showed that the pellet (insoluble fraction) contained the recombinant protein. The pellet was dissolved in a buffer containing 8.0 M urea, 50 mM NaCl, 1.0 mM CaCl2, 10 mM beta mercaptoethanol, and 50 mM Tris/HCl (pH 8.8) and was incubated at room temperature overnight. The mixture was centrifuged at 20,000 g and 25 °C for 60 min, and the supernatant was diluted 10-fold with a buffer containing 50 mM NaCl, 1.0 mM CaCl2, 10 mM MgSO4, and 50 mM Tris/HCl (pH 8.8). The recombinant alpha and beta tubulins were mixed together and were subjected to SDS-PAGE analysis.
Measurement of microtubule assembly
Because the microtubule formation is apparently facilitated at pH values lower than 7.0, the recombinant protein was passed through the HiTrapTM Desalting Column (5.0 mL, GE) to exchange the buffer for an assay buffer consisting of 1.0 mM GTP, 1.0 mM MgCl2, 1.0 mM EGTA, 5.0% glycerol, and 80 mM PIPES/NaOH (pH 6.8). The protein concentration was adjusted to be 0.8 mg/mL with the assay buffer, and the protein was stored at −80 °C until use. The frozen mixture of recombinant alpha and beta tubulins was thawed and centrifuged at 4 °C for 5 s. The mixture (100 μL) in a disposable ultra-micro cuvette (Biochrom Ltd) was maintained at 37 °C, and the OD350 was measured. Two minutes later, taxol in MeOH was added to the mixture, and the change in OD350 over a period of 30 min was measured.
Results
The total RNA was extracted from leaves of the yew T. cuspidata and was reverse-transcripted to cDNA. The degenerate primers for alpha tubulin were designed based on the sequences of 20C-W-E-L-Y-C-L-27E and 413M-E-E-G-E-F-S-420E, which were the consensus sequences between plants (Fig. S1). Accordingly, the degenerate primers for beta tubulin were designed on the consensus sequences of 19K-F-W-E-V-(V/I)-25C and 403M-D-E-M-E-F-T-410E (Fig. S2). PCR was demonstrated to amplify the internal sequence, and the RACE method was applied to obtain the full length of the cDNA. The oligonucleotide primers for human alpha and beta tubulins were designed based on the complete cDNA sequences in reference to the database (NP_006073.2 and NP_821133.1), and the full length of cDNA was amplified by PCR.
The plant alpha tubulin sequences, including that cloned from T. cuspidata in this study and that cloned from T. baccata by Tuszynski et al.Citation24), were aligned with that of human alpha tubulin cloned from HEK293T embryonic kidney cells in this study (Fig. S1). The alpha tubulins were highly conserved between plants. The amino acid residues specific to the alpha tubulin from the two Taxus spp. were 117Ile and 393Arg, whereas those found in the other species were Leu and His, respectively. The comparison of the alpha tubulin sequence from T. cuspidata with that from HEK293T cells revealed that multiple residues were different between them (Fig. S1). The plant beta tubulin sequences were then aligned with the beta tubulin from HEK293T cells (Fig. S2). The results of the extracted amino acid residues are shown in Fig. (A). The amino acid residues at 23, 26, 215, 217, 227–228, 231, 234, 270, 272–276, and 358–361 were the estimated binding site for taxol.Citation13) Most of those residues were well conserved between the different species. However, 26Glu was only shared by T. cuspidata, T. baccata, and E. grandis. In addition, 359Typ and 361Met were specific to the two Taxus spp. The other amino acid residues in T. cuspidata at positions 32, 37, 39, 84, 115, and 280 should also be analyzed. 32His and 32Thr were unique to T. cuspidata and T. baccata, respectively. 37Thr and 37His were also characteristic of T. cuspidata and T. baccata, respectively. 39Thr was shared with the two Taxus spp. and T. aestivum. 84Val was identified only in T. cuspidata. 115Ala and 280Asn were characteristic of the two Taxus spp. The comparison of the Taxus beta tubulin with that from HEK293T cells revealed a number of amino acid variations. Among these variations, D26E, R359W, and L361M may affect taxol binding.Citation13,24) These results inspired us to investigate the sensitivity of the microtubule of T. cuspidata toward taxol.
Fig. 2. Cloning and expression of tubulins from T. cuspidata.
Notes: (A) The extracted amino acid sequences of the beta tubulins from HEK293T cells and plants were aligned. The numbering of the amino acid residues was assigned either to the amino acid residues uniquely identified in T. cuspidata (plain letter) or to those important for taxol binding (white letter with black background). The boxed amino acid residues were conserved between species. The highlighted amino acid residues were relatively unique in T. cuspidata or the two Taxus spp. (B) 10% polyacrylamide gel electrophoresis (SDS-PAGE). The recombinant microtubules from T. cuspidata (Lane 1) and that from H. sapiens (Lane 2) were run on a 10% SDS-PAGE gel at 30 mV for 1 h and stained with Coomassie Brilliant Blue G250. The lower and upper bands present the recombinant alpha and the beta tubulins, respectively. The numbers placed in the left side of the protein marker (Lane M) indicate the marker size (kDa).
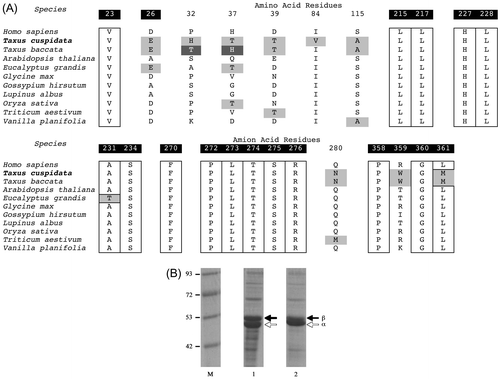
The cDNAs were subcloned into the protein expression vector pET28a, and the recombinant proteins were expressed robustly in Escherichia coli BL21 (DE3) cells, although all of the constructs were found in the inclusion body when expressed as recombinant proteins. Solubilization and refolding were necessary to obtain the soluble form of the recombinant proteins (Fig. (B)).Citation25–27) Lanes 1 and 2 present the mixture of the alpha and beta tubulins from T. cuspidata and HEK293T cells, respectively. The sizes of the two bands are ca. 50 kDa, which confirms the presence of the recombinant proteins and suggests that the solubilization and refolding were successfully conducted.
The microtubule assembly was monitored turbidimetrically by the increase in optical density (OD) at 350 nm (Fig. ). The application of 1.0 μM taxol to the recombinant alpha and beta tubulins from HEK293T cells caused a time-dependent increase in the OD350 (Fig. (A), solid line with open circles). The microtubule assembly was increased in the presence of 10 μM taxol (Fig. (A), solid line with closed circles), whereas the control (MeOH) exhibited little change in the OD350 (Fig. (A), solid line). The recombinant alpha and beta tubulins from the T. cuspidata were then investigated to assess the significance of the discovered variation. As shown in Fig. (B), taxol at a concentration of 1.0 μM (solid line with open circles) or 10 μM (solid line with closed circles) did not cause an increase in OD350 compared with the control (MeOH, solid line), clearly indicating that the microtubule assembly was suppressed. The mutant carrying 26Asp instead of Glu (E26D) exhibited higher sensitivity toward taxol than the wild type (Fig. (C)). The addition of taxol at a concentration of 1.0 μM resulted in an increase in OD350 against the mutant microtubule. We then attempted to express W359R mutant because 359Typ is not only important for taxol binding but also uniquely identified in T. cuspidata; however, we were unable to express it in E. coli. Instead, the double mutant E26D/W359R was expressed at a moderate level as a recombinant protein and was tested to investigate its ability to form microtubules in the presence of taxol. The double mutant provided a similar dose response to E26D (Fig. (D)), suggesting that the mutation at W359 may not affect taxol binding. Thus, the reduced binding affinity to taxol of the Taxus microtubule was achieved by the variation at 26Glu in the beta tubulin, which would infer a self-defense mechanism for T. cuspidata.
Fig. 3. Time course of taxol-induced microtubule assembly monitored by turbidity at 350 nm.
Notes: The microtubule formation in a buffer consisting of 80 mM PIPES/NaOH (pH 6.8), 5.0% glycerol, 1.0 mM GTP, 1.0 mM MgCl2, and 1.0 mM EGTA was observed at 37 °C for a period of 30 min. MeOH (solid line), 1.0 μM of taxol (solid line and open circles), or 10 μM taxol (solid line and closed circles) were added at 2 min, and the change in OD350 was recorded. The recombinant tubulins were from (A) HEK293T cells, (B) T. cuspidata, (C) T. cuspidata with the E26D mutation in the beta tubulin, and (D) T. cuspidata with the E26D and W359R mutations in the beta tubulin.
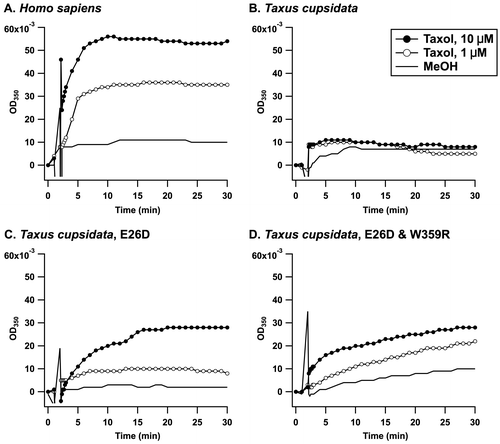
Discussion
A host species that accumulates secondary metabolites acquires tolerance with reduced affinity to the secondary metabolites. In the puffer fish Fugu pardalisCitation28) and the bivalve Mya arenaria,Citation29) one amino acid mutation leads to a reduction in the binding affinity to tetrodotoxin and saxitoxin, respectively, by more than 1000-fold. For the latter case, various species in the area exposed to paralytic shellfish poisoning exhibit different levels of tolerance to saxitoxin, which were thought to be the result of adaptive genetic evolution.Citation30) The garter snake Thamnophis sirtalis, which feeds on the tetrodotoxin-accumulating newt Taricha granulosa,Citation31) has several point mutations in its tetrodotoxin-binding sites, which contribute to its reduced sensitivity to tetrodotoxin. Camptothecin is a plant alkaloid that binds to DNA topoisomerase I and is known as an anti-cancer drug. The enzyme expressed in camptothecin-accumulating plants was mutated to be insensitive to the secondary metabolite.Citation32) With respect to tubulin-targeting natural products, it has been reported that colchicine is not as sensitive to plant microtubules as to animal microtubules,Citation21) although the molecular mechanism underlying the resistance has not yet been elucidated. We hypothesized that this acquisition of resistance would be applicable to taxol-accumulating species. This study revealed the complete amino acid sequences of the alpha and beta tubulins from T. cuspidata and identified significant mutations. Molecular cloning was conducted independently of a precedent study on T. baccata.Citation24) The alpha tubulin was identical to WWSSA2 (T. baccata). The beta tubulin from T. cuspidata was highly homologous to WWSSB1 (T. baccata, Fig. S2) with the following mutations: I7V, A18S, T32H, H37T, T40S, and I84V (Fig. (A)). Tuszynski et al. modeled the taxol and microtubule complex and speculated that the I24V, S25C, D26E, V229I, Q280N, and R359W mutations in the Taxus beta tubulin compared with human beta tubulin (Accession Number: P07437) affect taxol binding. Part of their working hypothesis for D26E and R359 W was validated for the first time in the present study. The recombinant microtubule composed of the alpha and beta tubulins from T. cuspidata was found to be more tolerant to taxol more than the microtubule from HEK293T cells. The treatment of cancer cells with microtubule-associated drugs, including taxol,Citation5) peloruside A,Citation33) and laulimalide,Citation34) facilitates the acquisition of the mutations in the beta tubulin of the resistant cells, although we cloned the alpha and beta tubulin cDNAs from HEK293T cells in the absence of taxol. In addition, the deduced amino acid sequences of alpha and beta human tubulins were identical to those registered in the database (Accession Number: NP_006073.2 and NP_821133.1), suggesting that the recombinant human microtubule is supposedly sensitive to taxol. In this study, the threshold taxol concentration for the accumulation of the human microtubule was found to be less than 1.0 μM (Fig. (A)), even though the threshold taxol concentration for the accumulation of the Taxus microtubule was more than 10 μM (Fig. (B)). This finding clearly suggests the difference in the affinity to taxol between the human microtubule and the Taxus microtubule. Furthermore, the E26D mutation in the Taxus beta tubulin restored its binding affinity to taxol, and microtubule assembly was observed in the presence of 1.0 μM taxol (Fig. (C)). The D26E mutation in the beta tubulin from a taxol-resistant cancer cell lineCitation7) was also identified in the beta tubulins from Eucalyptus pilularis (BAM05543), E. grandis (ABS50665), and Physcomitrella patens (AAQ88114). Another Eucalyptus sp., namely Eucalyptus perriniana, was practically utilized for the structural transformation of taxol.Citation35) P. patens was transfected with the biosynthetic gene for taxol and was confirmed to produce the biosynthetic intermediate.Citation36) Thus, these taxol-accumulating species including T. cuspidata, would be characterized as having the D26E mutation in common.
The other mutant R359W did not clearly affect the binding affinity to taxol. In addition, we could not investigate the effect of the L361M mutation. These are unique mutations identified in the Taxus beta tubulin and may contribute to the self-defense of T. cuspidata via a different mode of action. As in taxol-resistant cancer cells or tumors,Citation5) these mutations in the Taxus tubulin may have increased the microtubule dynamics, indicating that a reduction in the binding affinity toward taxol may not solely account for the self-resistance of T. cuspidata. Other possible speculations for the self-resistance of Taxus species against taxol are the following: (1) the plant tubulins are not as abundant as the mammalian tubulins, and (2) either taxol or the microtubule proteins may be segregated. Taxol was identified in all parts of the yew but was found to be concentrated in the bark and needles.Citation37) However, the subcellular localization of taxol has not yet been clearly understood. The localization of taxol in the cell wall was observed in suspension cultures of Taxus chinensisCitation38) and T. cuspidata.Citation39) In contrast, Fornalè reported that the exogenous addition of taxol results in its accumulation in the vacuoles rather than the cell wall of T. baccata cell suspension. (3) In addition, an export mechanism of taxol may be present in Taxus species,Citation40) and (4) a taxol may undergo a structural modification, which could reduce its toxicity and create conditions in which the microtubule is not affected by the toxin cocktail. All of these mechanisms would proceed in combination with each other and contribute to the self-resistance of T. cuspidata. It is thus acceptable that the direct effect of taxol on microtubule assembly was found to be marginal in this study.
In general, previous research studies have often described recombinant proteins as ‘functional’ even if they did not exhibit an identical level of functionality to that of the wild type and have not discussed the structural homogeneity of the recombinant proteins. In this study, the recombinant yew and human microtubules expressed in E. coli were trapped in inclusion bodies. Solubilization followed by refolding was necessary to obtain the functional proteins. A difference in the affinity to taxol between Taxus microtubules and human microtubules was observed, although this difference could be larger or smaller if the three-dimensional conformations of the recombinant proteins were properly regulated. Some yeast strains carry the chaperons necessary for tubulin expression, and the mutagenesis approach was applicable.Citation41) In the future, we may upgrade this research study by expressing the Taxus microtubule in the yeast.
Supplemental material
The supplemental material for this paper is available at http://dx.doi.org/10.1080/09168451.2014.940837.
Supplementary Materials
Download MS Word (956.5 KB)Acknowledgments
The authors would like to thank RIKEN BRC through the National Bio-Resource Project of the MEXT, Japan, for providing the human embryonic kidney cell line 293T (RCB2202).
Funding
This research was supported by JSPS KAKENHI [grant number 21603003] and [grant number 24510291] to KK, ERATO Murata Lipid Active Structure Project, Japan Science and Technology Agency (to KK), the SUNBOR grant (to KK), and the JSPS for Funding Program for the Next Generation World-Leading Researchers (LS012 to MYY).
References
- Wani MC, Taylor HL, Wall ME, Coggon P, McPhail AT. Plant antitumor agents VI. Isolation and structure of taxol, a novel antileukemic and antitumor agent from Taxus brevifolia. J. Am. Chem. Soc. 1971;93:2325–2327.10.1021/ja00738a045
- Rowinsky EK, Cazenave LA, Donehower RC. Taxol: a novel investigational antimicrotubule agent. J. Nat. Cancer Inst. 1990;82:1247–1259.10.1093/jnci/82.15.1247
- Schiff PB, Fant J, Horwitz SB. Promotion of microtubule assembly in vitro by taxol. Nature. 1979;277:665–667.10.1038/277665a0
- Bhalla K, Ibrado AM, Tourkina E, Tang C, Mahoney ME, Huang Y. Taxol induces internucleosomal DNA fragmentation associated with programmed cell-death in human myeloid-leukemia cells. Leukemia. 1993;7:563–568.
- Orr GA, Verdier-Pinard P, McDaid H, Horwitz SB. Mechanisms of taxol resistance related to microtubules. Oncogene. 2003;22:7280–7295.10.1038/sj.onc.1206934
- Giannakakou P, Sackett DL, Kang YK, Zhan Z, Buters JT, Fojo T, Poruchynsky MS. Paclitaxel-resistant human ovarian cancer cells have mutant β-tubulins that exhibit impaired paclitaxel-driven polymerization. J. Biol. Chem. 1997;272:17118–17125.10.1074/jbc.272.27.17118
- Hari M, Loganzo F, Annable T, Tan X, Musto S, Morilla DB, Nettles JH, Snyder JP, Greenberger LM. Paclitaxel-resistant cells have a mutation in the paclitaxel-binding region of β-tubulin (Asp26Glu) and less stable microtubules. Mol. Cancer Ther. 2006;5:270–278.10.1158/1535-7163.MCT-05-0190
- Shelanski Ml, Gaskin F, Cantor CR. Microtubule assembly in absence of added nucleotides. Proc. Nat. Acad. Sci. U.S.A. 1973;70:765–768.10.1073/pnas.70.3.765
- Ojima I, Duclos O, Dorman G, Simonot B, Prestwich GD. A new paclitaxel photoaffinity analog with a 3-(4-benzoylphenyl)propanoyl probe for characterization of drug-binding sites on tubulin and P-glycoprotein. J. Med. Chem. 1995;38:3891–3894.10.1021/jm00020a001
- Rao S, He L, Chakravarty S, Ojima I, Orr GA, Horwitz SB. Characterization of the taxol binding site on the microtubule: identification of Arg282 in β-tubulin as the site of photoincorporation of a 7-benzophenone analogue of taxol. J. Biol. Chem. 1999;274:37990–37994.10.1074/jbc.274.53.37990
- Xiao H, Verdier-Pinard P, Fernandez-Fuentes N, Burd B, Angeletti R, Fiser A, Horwitz SB, Orr GA. Insights into the mechanism of microtubule stabilization by taxol. Proc. Nat. Acad. Sci. U.S.A. 2006;103:10166–10173.10.1073/pnas.0603704103
- Nogales E, Wolf SG, Downing KH. Structure of the αβ-tubulin dimer by electron crystallography. Nature. 1998;391:199–203.10.1038/34465
- Löwe J, Li H, Downing KH, Nogales E. Refined structure of αβ-tubulin at 3.5 Å resolution. J. Mol. Biol. 2001;313:1045–1057.10.1006/jmbi.2001.5077
- Snyder JP, Nettles JH, Cornett B, Downing KH, Nogales E. The binding conformation of taxol in β-tubulin: a model based on electron crystallographic density. Proc. Nat. Acad. Sci. U.S.A. 2001;98:5312–5316.10.1073/pnas.051309398
- Wickremesinhe ERM, Arteea RN. Taxus callus cultures: initiation, growth optimization, characterization and taxol production. Plant Cell Tiss. Org. 1993;35:181–193.10.1007/BF00032968
- Stierle A, Strobel G, Stierle D. Taxol and taxane production by Taxomyces andreanae, an edophytic fungus of Pacific yew. Science. 1993;260:214–216.10.1126/science.8097061
- Stierle A, Strobel G, Stierle D, Grothaus P, Bignami G. The search for a taxol-producing microorganism among the endophytic fungi of the pacific yew, Taxus brevifolia. J. Nat. Prod. 1995;58:1315–1324.10.1021/np50123a002
- Expósito O, Bonfill M, Onrubia M, Jané A, Moyano E, Cusidó RM, Palazón J, Piñol MT. Effect of taxol feeding on taxol and related taxane production in Taxus baccata suspension cultures. New Biotechnol. 2009;25:252–259.10.1016/j.nbt.2008.11.001
- Mizuno K, Koyama M, Shibaoka H. Isolation of plant tubulin from azuki bean Epicotyls by ethyl N-phenylcarbamate-Sepharose affinity-chromatography. J. Biochem. 1981;89:329–332.
- Mizuno K. In vitro assembly of microtubules from tubulins of several higher plants. Cell Biol. Int. Rep. 1985;9:13–21.10.1016/0309-1651(85)90137-7
- Morejohn LC, Bureau TE, Tocchi LP, Fosket DE. Tubulins from different higher plant species are immunologically nonidentical and bind colchicine differentially. Proc. Natl. Acad. Sci. USA. 1984;81:1440–1444.10.1073/pnas.81.5.1440
- Koo BS, Kalme S, Yeo SH, Lee SJ, Yoon MY. Molecular cloning and biochemical characterization of α- and β-tubulin from potato plants (Solanum tuberosum L.). Plant Physiol. Biochem. 2009;47:761–768.10.1016/j.plaphy.2009.04.001
- Koo BS, Jang MH, Park H, Kalme S, Park HY, Han JW, Yeo YS, Yoon SH, Kim SJ, Lee CM, Yoon MY. Characterization of Capsicum annuum recombinant α- and β-tubulin. Appl. Biochem. Biotechnol. 2010;160:122–128.10.1007/s12010-008-8489-4
- Tuszynski JA, Craddock TJA, Mane JY, Barakat K, Tseng CY, Gajewski M, Winter P, Alisaraie L, Patterson J, Carpenter E, Wang WW, Deyholos MK, Li LJ, Sun X, Zhang Y, Wong GKS. Modeling the yew tree tubulin and a comparison of its interaction with paclitaxel to human tubulin. Pharm. Res. 2012;29:3007–3021.10.1007/s11095-012-0829-y
- Wampande EM, Richard McIntosh J, Lubega GW. Classical ligands interact with native and recombinant tubulin from Onchocerca volvulus with similar rank order of magnitude. Protein Expression Purif. 2007;55:236–245.10.1016/j.pep.2007.04.011
- Ding J, Bao WC, Liu Q, Yu Q, Abdille MH, Wei Z. Immunoprotection of chickens against Eimeria acervulina by recombinant α-tubulin protein. Parasitol. Res. 2008;103:1133–1140.10.1007/s00436-008-1106-1
- Giles NL, Armson A, Reid SA. Characterization of trifluralin binding with recombinant tubulin from Trypanosoma brucei. Parasitol. Res. 2009;104:893–903.10.1007/s00436-008-1271-2
- Yotsu-Yamashita M, Nishimori K, Nitanai Y, Isemura M, Sugimoto A, Yasumoto T. Binding properties of ³H-PbTx-3 and ³H-saxitoxin to brain membranes and to skeletal muscle membranes of puffer fish Fugu pardalis and the primary structure of a voltage-gated Na+ channel alpha-subunit (fMNa1) from skeletal muscle of F. pardalis. Biochem. Biophys. Res. Commun. 2000;267:403–412.10.1006/bbrc.1999.1974
- Bricelj VM, Connell L, Konoki K, MacQuarrie SP, Scheuer T, Catterall WA, Trainer VL. Sodium channel mutation leading to saxitoxin resistance in clams increases risk of PSP. Nature. 2005;434:763–767.10.1038/nature03415
- Bricelj VM, Shumway SE. Paralytic shellfish toxins in bivalve molluscs: occurrence, transfer kinetics, and biotransformation. Rev. Fish. Sci. 1998;6:383–383.
- Geffeney SL, Fujimoto E, Brodie ED 3rd, Brodie ED Jr, Ruben PC. Evolutionary diversification of TTX-resistant sodium channels in a predator-prey. Nature. 2005;434:759–763.10.1038/nature03444
- Sirikantaramas S, Yamazaki M, Saito K. Mutations in topoisomerase I as a self-resistance mechanism coevolved with the production of the anticancer alkaloid camptothecin in plants. Proc. Nat. Acad. Sci. U.S.A. 2008;105:6782–6786.10.1073/pnas.0801038105
- Begaye A, Trostel S, Zhao ZM, Taylor RE, Schriemer DC, Sackett DL. Mutations in the β-tubulin binding site for peloruside A confer resistance by targeting a cleft significant in side chain binding. Cell Cycle. 2011;10:3387–3396.10.4161/cc
- Kanakkanthara A, Crawford J, Rawson P, Kivell B, Northcote PT, Miller JH. Resistance to the microtubule-stabilizing agents, peloruside A and laulimalide, is associated with multiple β-tubulin alterations. EJC Suppl. 2010;8:100–100.10.1016/S1359-6349(10)72020-6
- Hamada H, Sanada K, Furuya T, Kawabe S, Jaziri M. Biotransformation of paclitaxel by cell suspension cultures of Eucalyptus perriniana. Nat. Prod. Lett. 1996;9:47–52.10.1080/10575639608043577
- Anterola A, Shanle E, Perroud PF, Quatrano R. Production of taxa-4(5),11(12)-diene by transgenic Physcomitrella patens. Transgenic Res. 2009;18:655–660.10.1007/s11248-009-9252-5
- Vidensek N, Lim P, Campbell A, Carlson C. Taxol content in bark, wood, root, leaf, twig, and seedling from several Taxus species. J. Nat. Prod. 1990;53:1609–1610.10.1021/np50072a039
- Choi HK, Kim SI, Song JY, Son JS, Hong SS, Durzan DJ, Lee HJ. Localization of paclitaxel in suspension culture of Taxus chinensis. J. Microbiol. Biotechnol. 2001;11:458–462.
- Russin WA, Ellis DD, Gottwald JR, Zeldin EL, Brodhagen M, Evert RF. Immunocytochemical localization of taxol in Taxus cuspidata. Int. J. Plant Sci. 1995;156:668–678.10.1086/ijps.1995.156.issue-5
- Fornalè S, Esposti DD, Navia-Osorio A, Cusidò RM, Palazòn J, Teresa Piñol M, Bagni N. Taxol transport in Taxus baccata cell suspension cultures. Plant Physiol. Biochem. 2002;40:81–88.10.1016/S0981-9428(01)01332-8
- Uchimura S, Oguchi Y, Katsuki M, Usui T, Osada H, Nikawa J, Ishiwata S, Muto E. Identification of a strong binding site for kinesin on the microtubule using mutant analysis of tubulin. EMBO J. 2006;25:5932–5941.10.1038/sj.emboj.7601442