Abstract
Several concentrations of theobromine (TB) and (−)-epicatechin (EC) were coadministered to rats, and plasma EC and its metabolites were determined using ultra-high-performance liquid chromatography–tandem mass spectrometry. It has been demonstrated that TB increases the absorption of EC in a dose-dependent manner. Cocoa powder had a similar effect, and the mechanism involved is not thought to depend on tight junctions.
Cacao beans (Theobroma cacao), a dietary ingredient with worldwide popularity since ancient times, are rich in polyphenolic compounds, particularly flavonoids. Its constituents are predominantly flavan-3-ols, including (−)-epicatechin (EC), (+)-catechin, and its oligomers known as procyanidins.Citation1) There are several reports describing the health benefits of cacao, such as prevention of cardiovascular disease,Citation2) diabetes,Citation3) oxidative stress,Citation4) and obesity.Citation5) In particular, EC, which is the largest component of cacao, has received much attention. Moreover, there has been a great deal of research into the absorption and metabolism of flavan-3-ols, and the details of pharmacokinetic properties are becoming clear. Orally ingested flavan-3-ol is metabolized into diverse compounds following sulfate conjugation, glucuronate conjugation, and methylation in the small intestine or liver.Citation6) Although it is still unknown whether flavan-3-ols functions with aglycon or conjugates at the target organs in the body, the absorptivity of flavan-3-ols is nonetheless important. However, the bioavailability of flavan-3-ols is not high, and that of procyanidins with a high degree of polymerization is very low. It has been reported that even EC, which has relatively good absorptivity properties among polyphenols, is absorbed in its aglycon form at a small percentage.Citation7)
Tea and coffee contain a large quantity of caffeine. In contrast, cacao contains more of the methylxanthine, theobromine (TB), than caffeine, and this bitter-tasting substance contributes to the characteristic flavor of cacao. TB is now widely accepted as a food additive, and has various physiological functions such as vessel dilatation. Furthermore, at physiological concentrations, TB has weak awakening effects compared with caffeine. However, it is toxic for some animals, such as dogs, that do not possess appropriate metabolic capabilities.Citation8)
In the combination of major polyphenol and methylxanthine of tea, it was reported that the absorptivity of epigallocatechin-3-gallate (EGCG) was enhanced by changing the mixture ratio of EGCG and caffeine.Citation9) Although there have been numerous reports on the bioavailability of EC,Citation10–12) these have not provided any information about whether TB affects the bioavailability of EC. The aim of the present research was to assess whether TB would raise absorption of EC in rats. Moreover, we studied the underlying mechanism using human colonic cell line Caco-2, a well-accepted model of intestinal absorption for studying transportation of EC.Citation13,14)
Six-week-old male Wistar rats were obtained from Japan SLC (Tokyo, Japan), and housed individually at 23 ± 1 °C in a temperature-controlled room with a 12 h light–dark cycle. After acclimatization with free access to a pellet diet (Oriental Pellet Diet MF, Oriental Yeast, Tokyo, Japan) and water for one week, the rats were divided into four groups (n = six per group). The first experiment was performed by mixing EC (10 mg/kg body weight) and TB (100, 200, and 400 mg/kg) or vehicle (control) in 0.5% methylcellulose solution. In the second experiment, TB was added to a commercially available cocoa powder at different ratios (TB/EC = 1.4, 5, 10, and 20), and the cocoa powder was suspended in 0.5% methylcellulose solution. The main composition of the cocoa powder used is shown in Table .
Table 1. Composition of cocoa powder.
Each rat was fasted for 12 h following oral administration of test sample via a stomach sonde. Blood was collected in heparinized tubes from the caudal vein at 0–5 or 0–6 h after ingestion, and plasma samples were separated by centrifugation at 3000 g for 15 min at 4 °C. Plasma samples were treated as described previously,Citation15,16) however, with slight modifications. In brief, 66 μL plasma was mixed with 6 μL of 0.2 M phosphate buffer (pH 6.8) and 18 μL of 2% ascorbic acid with 0.01% EDTA containing sulfatase type VIII (150 U) from abalone entrails (Sigma Chemical Co., St. Louis), and β-glucuronidase type VII-A (1,250 U) from Escherichia coli (Sigma Chemical Co., St. Louis). The mixture was incubated at 37 °C for 45 min to hydrolyze conjugated metabolites into free form. For ultra-high-performance liquid chromatography–tandem mass spectrometry (UPLC-MS) experiments, 75 μL of the reaction product was added to 675 μL of 0.83 M acetic acid dissolved in methanol. The mixture was vortexed for 30 s, sonicated for 30 s, and finally centrifuged at 5000 g for 5 min at 4 °C. The supernatant obtained was evaporated and dissolved in 750 μL of methanol, and 3 μL was injected into an Acquity UPLC BEH-C18 reversed-phase column (column size 2.1 × 100 mm, particle size 1.7 μm) and a Waters Acquity UPLC system coupled to a Xevo Quadrupole Time-of-Flight (QTOF)-MS system (Waters, Milford, MA). The flow rate was set at 0.1 mL/min. The mobile phase comprised of two solvent solutions that were mixed according to the detection method used: solvent A, water/formic acid (99.9:0.1, v/v); solvent B, acetonitrile/formic acid (99.9:0.1). The buffer gradient consisted of 0.1–30% B for 0–7 min, 30–99.9% B for 7–7.1 min, 99.9% B for 7.1–10.75 min, 99.9–0.1% B for 10.75–11 min, and 0.1% B for 4 min before the next injection. For EC and 3ʹ-O-methyl epicatechin (3ʹOME) metabolites detection, the capillary, sampling cone, and extraction cone voltages were set at 3000, 30, and 3.0 V, respectively. The source and desolvation temperatures were 120 and 450 °C, respectively, while the cone and desolvation gas flow rates were set at 50 and 800 L/h, respectively. The amount of EC and 3ʹOME was estimated from calibration curves obtained using analytical-grade standards compounds. The peak area of m/z [M-H] ± 0.05 Da was divided by the area of the standard. This value was used to generate calibration curves. The metabolites of EC are detectable as aglycon or a methylated EC by considering enzymatic treatment of a sulfatase and β-glucuronidase. When the standard of EC, 3ʹOME, and 4ʹ-O-methyl epicatechin (4′OME) were analyzed using the method described above, the selected masses of [M-H]− and retention times (RT) displayed were EC (m/z 289.07, RT 5.03 min) and 3ʹOME (m/z 303.09, RT 5.96 min).
Therefore, analysis of EC metabolites was possible using the same method. In the preliminary examination, we checked that the methylation metabolite of EC from rat plasma was not 4ʹOME but 3ʹOME, which is in agreement with a previous report.Citation11) EC was suspended in 0.5% methylcellulose solution with TB, and then administered orally to rats that had fasted for 12 h. Depending on the TB concentration, the concentration of EC and 3ʹOME in blood significantly increased. Plasma EC levels of TB (100 and 200 mg/kg) in coadministered rats were higher than with only EC administration at 75 min when the blood concentration of EC served as a peak. Administration of 400 mg/kg TB significantly increased EC concentration in blood from 30 min to 4 h (Fig. (A)). The area under the curve (AUC) of EC in the 400 mg/kg TB group was 1.8 times higher than the control group (Fig. (B)). 3ʹOME also displayed the same tendency; plasma 3ʹOME levels in 400 mg/kg TB group were higher than in the control group at 30 min, 4 h, and 6 h (Fig. (C)). TB doses of 200 and 400 mg/kg increased the AUC of 3ʹOME compared with EC only (Fig. (D)).
Next, the effect of TB addition to commercial cocoa powder and the effect of cacao to absorption of EC were investigated. The original cocoa powder contained 2.59% TB and 1.82% EC at an approximate TB/EC ratio of 1.4. This cocoa powder contained more flavan-3-ols than typical cocoa powder in which the TB/EC ratio was approximately 10, and the composition of other ingredients was equivalent. In order to obtain enough plasma EC to reach detection levels, this powder was selected as the control powder. Considering the typical powder, a TB/EC ratio of 10 is available. TB was added so that the ratio of TB/EC could be adjusted to 5, 10, and 20. The time course change of plasma EC concentration increased depending on TB concentration (Fig. (E)), and the AUC of EC significantly increased at TB/EC ratios of 10 and 20 (Fig. (F)). Similar findings were detected for 3ʹOME (data not shown). Interestingly, compared with coadministration of pure EC and TB (Fig. (A)), a moderate reduction in plasma EC concentration after 2 h was observed, following examination of TB/EC ratios at which the TB concentration of cacao powder increased, particularly TB/EC = 20 (Fig. (E)). This may be due to the matrix of cacao affecting absorption of EC and the effects of TB.
It has been reported that 3-isobutyl-1-methylxanthine, which inhibits phosphodiesterase, adjusts the function of tight junctions in epithelial cells from dog kidney (MDCK cells),Citation17) and procyanidin oligomers are absorbed by the epithelium via a paracellular route in the Caco-2 cell model.Citation18) Therefore, in order to investigate the mechanism by which TB promotes EC absorption, the effect of TB on tight junction function was examined in a Caco-2 cell line. Caco-2 cells were cultured in Eagle’s Minimum Essential Medium with 10% fetal bovine serum, 1% nonessential amino acids, 100 U/mL penicillin, and 0.1 mg/mL streptomycin. Cells were grown in a humidified atmosphere of 5% CO2 at 37 °C. For transport studies, Caco-2 cells were seeded in Transwell® inserts (polycarbonate membrane, 0.4 μM pore size, 12 mm internal diameter, Corning Costar Corp., NY, USA) in 12-well plates at a density of 105 cells/cm2. Transepithelial electrical resistance (TEER) values across the cell monolayers were measured using a Millicell-ERS Voltohmmeter (Millipore Corp., Billerica, MA, USA). Transport buffer was Hanks’ balanced salt solution containing 25 mM of HEPES (pH 7.4), and inserts with TEER values > 350 Ω cm2 in culture medium were selected for experiments. Although maximum plasma EC concentration was 3 μg/mL (10 μM), the concentration of EC was predominantly adjusted to 50–100 μM as in several previous reports with Caco-2.Citation13,19)
Then, 0.5 mL transport buffer containing 100 μM EC with 100 μM TB or 3 mM lauric acid as positive control was added to the apical side of the inserts. The receiving chamber contained 1.5 mL of transport buffer. TEER values were obtained after incubation for 1 or 3 h at 37 °C. TEER values in the TB group following 3 h incubation were stable, suggesting that the barrier function of tight junctions was sustained (Fig. ). On the other hand, TEER decreased significantly within 1 h of incubation with lauric acid, which proved appropriate as a positive control. This indicated that the increase in EC absorption by TB was not dependent on function of the tight junction.
Fig. 1. Time course changes (A, C, E) and AUC (B, D, F) of plasma EC and 3ʹOME after ingestion of EC (A–D) and cocoa powder (E, F).
Notes: Values are expressed as means with standard error of the mean (n = 6). For time-course data, *p < 0.05 compared with corresponding control values, (Student’s test). For AUC data, means without a common letter differ, p < 0.05 (Tukey’s test).
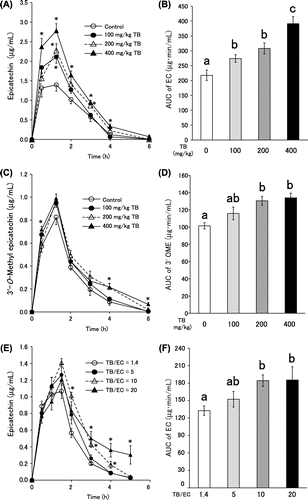
Fig. 2. Effects of TB on TEER in Caco-2 cell monolayers.
Notes: The open circles, open triangles, and solid circles represent control, 100 μM TB, and 3 mM lauric acid groups, respectively. Values are expressed as means with standard error of the mean (n = 6). *p < 0.05 compared with corresponding control values, (Dunnett’s test).
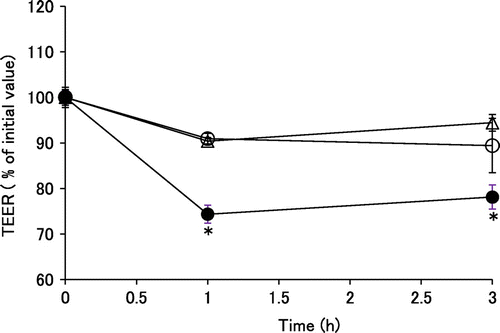
In this report, we demonstrated that TB enhanced the absorption of EC in rats and the mechanism did not involve uncoupling at the tight junction. Nakagawa et al. reported that caffeine suppressed conjugation reactions of EGCG, such as glucuronidation and sulfation, and suggested that as well as absorption from the intestinal tract, regulation by conjugation and excretion were also important.Citation9) Similar changes may have occurred in the case of cacao. Another explanation may be based on the finding that multidrug resistance proteins (MRPs) interact with flavonoids.Citation20) Caffeine and TB are phosphodiesterase inhibitors that can prevent inactivation of cAMP and cGMP, and there is evidence to suggest that the sorting of MRP2 is regulated by cAMP.Citation21) Although more detailed research is necessary, there is a possibility that TB adjusts the absorption of EC through MRPs. Since cacao promotes the bioavailability of polyphenols, modifying cocoa powder to include physiologically relevant concentrations of TB may provide benefit to human health.
Acknowledgments
We thank Dr Kiyotaka Nakagawa and Dr Teruo Miyazawa (Food and Biodynamic Chemistry Laboratory, Graduate School of Agricultural Science, Tohoku University) for their advice in preparing this manuscript, and Miss Manami Sakai for her technical assistance.
Author Contribution
N.T. and T.K. designed research; T.Y., H.T., and T.G. performed research; K·S., A.H., and M.K. discussed data; T.Y. wrote the paper.
Notes
Abbreviations: AUC, area under the curve; EC, (−)-epicatechin; EGCG, epigallocatechin-3-gallate; MRP, multidrug resistance protein; 3′OME, 3′-O-methyl epicatechin; RT, retention time; TB, theobromine; TEER, transepithelial electrical resistance; UPLC-MS, ultra-high-performance liquid chromatography–tandem mass spectrometry.
References
- Sánchez-Rabaneda F, Jáuregui O, Casals I, Andrés-Lacueva C, Izquierdo-Pulido M, Lamuela-Raventós RM. Liquid chromatographic/electrospray ionization tandem mass spectrometric study of the phenolic composition of cocoa (Theobroma cacao). J. Mass Spectrom. 2003;38:35–42.10.1002/(ISSN)1096-9888
- Zomer E, Owen A, Magliano DJ, Liew D, Reid CM. The effectiveness and cost effectiveness of dark chocolate consumption as prevention therapy in people at high risk of cardiovascular disease: best case scenario analysis using a Markov model. BMJ. 2012;344:e3657.10.1136/bmj.e3657
- Yamashita Y, Okabe M, Natsume M, Ashida H. Prevention mechanisms of glucose intolerance and obesity by cacao liquor procyanidin extract in high-fat diet-fed C57BL/6 mice. Arch. Biochem. Biophys. 2012;527:95–104.10.1016/j.abb.2012.03.018
- Suzuki K, Nakagawa K, Miyazawa T, Kato S, Kimura F, Kamei M, Miyazawa T. Oxidative stress during development of alcoholic fatty liver: therapeutic potential of cacao polyphenol. Biosci. Biotechnol., Biochem. 2013;77:1792–1794.10.1271/bbb.130380
- Matsui N, Ito R, Nishimura E, Yoshikawa M, Kato M, Kamei M., Shibata H., Matsumoto I., Abe K., Hashizume S. Ingested cocoa can prevent high-fat diet-induced obesity by regulating the expression of genes for fatty acid metabolism. Nutrition. 2005;21:594–601.10.1016/j.nut.2004.10.008
- Donovan JL, Crespy V, Manach C, Morand C, Besson C, Scalbert A, Rémésy C. Catechin is metabolized by both the small intestine and liver of rats. J. Nutr. 2001;131:1753–1757.
- Manach C, Scalbert A, Morand C, Rémésy C, Jiménez L. Polyphenols: food sources and bioavailability. Am. J. Clin. Nutr. 2004;79:727–747.
- Gans JH, Korson R, Cater MR, Ackerly CC. Effects of short-term and long-term theobromine administration to male dogs. Toxicol. Appl. Pharmacol. 1980;53:481–496.10.1016/0041-008X(80)90360-9
- Nakagawa K, Nakayama K, Nakamura M, Sookwong P, Tsuduki T, Niino H, Kimura F, Miyazawa T. Effects of co-administration of tea epigallocatechin-3-gallate (EGCG) and caffeine on absorption and metabolism of EGCG in humans. Biosci. Biotechnol., Biochem. 2009;73:2014–2017.10.1271/bbb.90195
- Ottaviani JI, Momma TY, Kuhnle GK, Keen CL, Schroeter H. Free Radical Biol. Med. 2012;52:1403–1412.10.1016/j.freeradbiomed.2011.12.010
- Natsume M, Osakabe N, Oyama M, Sasaki M, Baba S, Nakamura Y, Osawa T, Terao J. Structures of (−)-epicatechin glucuronide identified from plasma and urine after oral ingestion of (−)-epicatechin: differences between human and rat. Free Radical Biol. Med. 2003;34:840–849.10.1016/S0891-5849(02)01434-X
- Roura E, Andrés-Lacueva C, Jáuregui O, Badia E, Estruch R, Izquierdo-Pulido M, Lamuela-Raventós RM. Rapid liquid chromatography tandem mass spectrometry assay to quantify plasma (−)-epicatechin metabolites after ingestion of a standard portion of cocoa beverage in humans. J. Agric. Food. Chem. 2005;53:6190–6194.10.1021/jf050377u
- Salucci M, Stivala LA, Maiani G, Bugianesi R, Vannini V. Flavonoids uptake and their effect on cell cycle of human colon adenocarcinoma cells (Caco2). Br. J. Cancer. 2002;86:1645–1651.10.1038/sj.bjc.6600295
- Kosińska A, Andlauer W. Cocoa polyphenols are absorbed in Caco-2 cell model of intestinal epithelium. Food Chem. 2012;135:999–1005.10.1016/j.foodchem.2012.05.101
- Piskula MK, Terao J. Accumulation of (−)-epicatechin metabolites in rat plasma after oral administration and distribution of conjugation enzymes in rat tissues. J. Nutr. 1998;128:1172–1178.
- Abd El Mohsen MM, Kuhnle G, Rechner AR, Schroeter H, Rose S, Jenner P, Rice-Evans CA. Uptake and metabolism of epicatechin and its access to the brain after oral ingestion. Free Radical Biol. Med. 2002;33:1693–1702.10.1016/S0891-5849(02)01137-1
- Cereijido M, González-Mariscal L, Contreras RG, Gallardo JM, García-Villegas R, Valdés J. The making of a tight junction. J. Cell Sci. Suppl. 1993;17:127–132.10.1242/jcs.1993.Supplement_17.18
- Deprez S, Mila I, Huneau JF, Tome D, Scalbert A. Transport of proanthocyanidin dimer, trimer, and polymer across monolayers of human intestinal epithelial Caco-2 cells. Antioxid. Redox Signal. 2001;3:957–967.10.1089/152308601317203503
- Kadowaki M, Sugihara N, Tagashira T, Terao K, Furuno K. Presence or absence of a gallate moiety on catechins affects their cellular transport. J. Pharm. Pharmacol. 2008;60:1189–1195.10.1211/jpp.60.9.0011
- Vaidyanathan JB, Walle T. Transport and metabolism of the tea flavonoid (−)-epicatechin by the human intestinal cell line Caco-2. Pharm. Res. 2001;18:1420–1425.10.1023/A:1012200805593
- Roelofsen H, Soroka CJ, Keppler D, Boyer JL. Cyclic AMP stimulates sorting of the canalicular organic anion transporter (Mrp2/cMoat) to the apical domain in hepatocyte couplets. J. Cell Sci. 1998;111:1137–1145.
- Hammerstone JF, Lazarus SA, Mitchell AE, Rucker R, Schmitz HH. Identification of procyanidins in cocoa (Theobroma cacao) and chocolate using high-performance liquid chromatography/mass spectrometry. J. Agric. Food. Chem. 1999;47:490–496.10.1021/jf980760h
- Ortega N, Romero MP, Macià A, Reguant J, Anglès N, Morelló JR, Motilva MJ. Obtention and characterization of phenolic extracts from different cocoa sources. J. Agric. Food. Chem. 2008;56:9621–9627.10.1021/jf8014415
- Craig WJ, Nguyen TT. Caffeine and theobromine levels in cocoa and carob products. J. Food Sci. 1984;49:302–303.10.1111/jfds.1984.49.issue-1