Abstract
α-1,4-Glucan lyases [glycoside hydrolase family (GH) 31] catalyze an elimination reaction to form 1,5-anhydro-d-fructose (AF), while GH31 α-glucosidases normally catalyze a hydrolytic reaction. We determined that a small amount of AF was produced by GH31 Aspergillus niger α-glucosidase from maltooligosaccharides by elimination reaction, likely via an oxocarbenium ion intermediate.
1,5-Anhydro-d-fructose (AF) is found in micro-organisms,Citation1) red algae,Citation2−Citation5) and rat liver tissue.Citation6) AF is a unique ketomonosaccharide that can exist in equilibrium as the tautomers of its ketone, enol, enediol, and hydrated forms (Fig. (A)). α-1,4-Glucan lyase (GLase; EC 4.2.2.13) produces AF by the elimination-mediated degradation of starch, glycogen, or maltooligosaccharides.Citation2,7) Glucoside hydrolase family (GH) 31 enzymes include those with the following activities: α-xylosidase, α-glucosidase (AGase; EC 3.2.1.20), α-1,3-glucosidase, sucrase, isomaltase, isomaltosyltransferase, and GLase.Citation8−Citation11) GH31 proteins share the same catalytic domain containing a (β/α)8 barrel fold in which two Asp residues on the β4 and β6 strands are the catalytic nucleophile and general acid/base catalyst, respectively.Citation12−Citation14) Most GH31 members are glycosylases that catalyze a retaining hydrolytic reaction, while only GLase catalyzes an elimination reaction. AGase (a major enzyme of GH31) and GLase share about 25% sequence similarity, where the seven well-conserved regions are essential for catalytic activity.Citation15) An inhibition study using acarbose, 1-deoxynojirimycin, carbodiimides, and 5-fluoro-β-l-idopyranosyl fluoride also demonstrated that AGase and GLase share the same mechanism for accepting inhibitors.Citation7,15,16) Furthermore, both enzymes recognize the α-1,4-glucosidic linkage with high specificity.Citation7,17) These observations suggest that AGase could also generate AF as a product by catalyzing an elimination reaction. In this study, we investigated whether Aspergillus niger AGase (ANGase; a GH31 AGase) could produce AF using maltooligosaccharides (maltose to maltohexaose; G2 to G6) as substrates.
GLase was kindly supplied by Dr. Shukan Yu (Danisco A/S, Copenhagen, Denmark). Recombinant ANGase was prepared as reported previously.Citation18) G2 was purchased from Nacalai Tesque (Kyoto, Japan); G3 to G6 and O-ethylhydroxylamine/HCl were from Wako Pure Chemical Industries, Ltd. (Osaka, Japan). Preparation of [12C]AF or [13C]AF was done according to the following procedure. A reaction mixture (10 mL) containing 2.5 mL of 0.2 M sodium acetate buffer (pH 4.0), 5.0 mL of 3% algae starch labeled with [12C] or [13C] (Shoko Co. Ltd., Tokyo, Japan) and 2.5 mL of GLase (0.66 mg/mL) was incubated at 37 °C for 4 h. The reaction was stopped by adding 20 mL of 99% ethanol, followed by incubation on ice for more than 1 h to allow the starch to precipitate. The precipitate was discarded by centrifugation at 9,000 × g for 10 min at 4 °C. The supernatant containing [12C]AF or [13C]AF was evaporated to dryness, dissolved in 3 mL of 0.05 mM HCl (pH 4.35), and desalted with Amberlite MB-4 (Organo, Tokyo, Japan; for more than 2–3 h, two times). The sample was purified with gel filtration using Bio-Gel P-2 (Bio-Rad, Hercules, CA, USA; 2 × 80 cm; 0.05 mM HCl, pH 4.35; 0.5 mL/min), and the detection of [12C]AF or [13C]AF in each fraction was done by the method of Yu et al.Citation19) The fraction containing [12C]AF or [13C]AF was concentrated, and its purity was checked by thin-layer chromatography (TLC).Citation20) The concentrated sample was subjected to reverse-phase HPLC equipped with a COSMOSIL Sugar-D column (Nacalai Tesque; 4.6 × 250 mm; isocratic elution using 80% acetonitrile at 30 °C; flow rate of 1.0 mL/min; refractive index detector), and then the fraction containing AF was collected and evaporated. The structure of the purified [12C]AF or [13C]AF was analyzed by FD-MS (JEOL JMS-T100GCV, Tokyo, Japan) and NMR (1H-NMR and 13C-NMR; Bruker AMX500, Rheinstetten, Germany). Preparation of the O-ethyloxime-derivative of AF (AF-OX; i.e. [12C]AF-OX or [13C]AF-OX) was done in accordance with the following method.Citation6) A reaction mixture containing AF and O-ethylhydroxylamine/HCl at 1:4 (by weight) was incubated at room temperature for 24 h in the dark. AF-OX was purified by HPLC using a COSMOSIL Cholester Column (Nacalai Tesque; 4.6 × 250 mm) using a gradient system of 0–55% acetonitrile (by volume) at a flow rate of 0.8 mL/min and 30 °C with detection at 207 nm. Purified [12C]AF-OX or [13C]AF-OX was evaporated and its structure was determined using ESI-MS (Waters, Milford, MA, USA) and NMR. ANGase-catalyzed [12C]AF-production was examined by the following procedure. A reaction mixture containing 0.04 M sodium acetate buffer (pH 4.0), 10 mM of G2-G6, and 0.3 μg/mL of ANGase was incubated at 37 °C for 2–120 min, and then a 50 μL aliquot of the mixture was subjected to a glucose test (Glucose C II-Test; Wako Pure Chemical Industries, Ltd.)Citation21), and 500 μL of the mixture was used for the AF assay as follows: to 500 μL of the mixture, 35 μL of 500 mg/mL O-ethylhydroxylamine/HCl, and 10 μL of 0.1 mM [13C]AF (internal standard) were added and then incubated at room temperature in the dark for 24 h, followed by desalting with Amberlite MB-4 (for more than 2–3 h; two times). The AF-OX was purified by HPLC using a Chorestor column and the above-described procedure. The fraction containing AF-OX was concentrated and dissolved in 50 μL of water. The [12C]AF-OX derived from the ANGase-reaction was confirmed by LC-MS-MS (UPLC-MS-MS; Waters) using the following separation conditions for LC: column, UPLC BEH (C18; 2.1 × 100 mm; Waters); mobile phase, a linear gradient of 0–50% acetonitrile (in 0.1% formic acid); and flow rate, 0.25 mL/min at 38 °C. The amount of [12C]AF-OX (molecular mass of 205) in the sample was calculated by comparing the peak area of the internal standard [13C]AF-OX (molecular mass of 211).
Both [12C]AF and [13C]AF were synthesized by a GLase-catalyzed reaction from [12C]starch and [13C]starch, respectively, and purified using gel-filtration chromatography and HPLC. FD-MS analysis of isolated [12C]AF and [13C]AF exhibited positive ions at m/z 163 ([M+H]+) with a molecular formula of C6H10O5 and at m/z 169 ([M+H]+) with a molecular formula of [13C6]H10O5, respectively, indicating that each AF was in the dehydrated form. 1H-NMR and 13C-NMR analysis revealed that both compounds showed identical chemical shifts to the reference data.Citation22,23) The aforementioned structural analysis confirmed that [12C]AF and [13C]AF were successfully produced and purified, even though AF was unstable in the weakly alkaline aqueous solution.Citation24) Analysis of AF was also difficult because of its tautomerization to several isomers (Fig. (A)), and therefore the conversion of AF to AF-OX allowed us to assay AF more conveniently [AF-OX is where the C-2 position (carbonyl group) of AF was modified with O-ethylhydroxylamine (see Fig. (B))]. Structures of [12C]AF-OX and [13C]AF-OX were confirmed by MS and NMR. ESI-MS exhibited positive ions at m/z 206 ([M+H]+) and m/z 228 ([M+Na]+) for [12C]AF-OX with a molecular formula of C8H15O5 N and m/z 212 ([M+H]+) and m/z 234 ([M+Na]+) for [13C]AF-OX with a molecular formula of [13C]C6[12C]C2H15O5 N. NMR analysis (Citation1H NMR and 13C-NMR) also supported the structures of [12C]AF-OX and [13C]AF-OX.
Generation of [12C]AF from the ANGase-mediated reaction from each of G2 to G6 (at 37 °C for 2, 5, 10, 20, 30, 60, and 120 min) was examined by conversion of the product to the O-ethyloxime-derivative which could be measured by LC-MS-MS. EI-MS (the first MS of the LC-MS-MS; positive ion mode) detected both the signals of m/z 206 ([M+H]+) for [12C]AF-OX and m/z 212 ([M+H]+) for [13C]AF-OX from the peak having the same retention time in the LC. During the initial reaction time, [12C]AF-OX was rapidly produced, albeit at a very low level, and then gradually increased. Comparatively, the concentration of glucose (hydrolytic product) in each reaction continuously increased over time to significantly higher concentrations (Fig. ). Based on the results from our previous reportCitation25), which showed that GH31 α-glucosidases (including ANGase) could convert AF to glucose via a hydration reaction, it was likely that the AF produced was quickly converted to glucose before it could significantly accumulate.
Fig. 2. Production of AF (left panels) and glucose (right panels) by ANGase using the substrates G2 to G6.
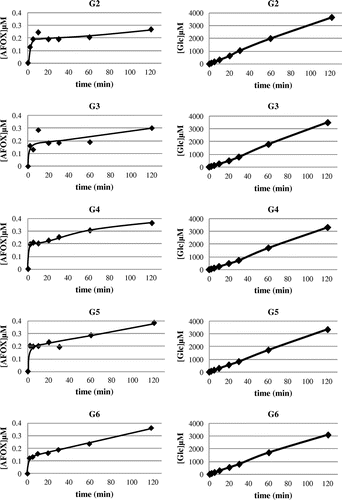
The mechanism by which AF is produced by ANGase is unknown, even though Hirano et al.Citation5) also observed AF-formation by an N-glycan-trimming-type α-1,3-glucosidase (EC 3.2.1.130; GH31 enzyme). Formation of AF is considered to be directly related to the reaction mechanism, where two models are proposed: a nucleophilic displacement mechanism and an oxocarbenium ion intermediate mechanism (Fig. (A) and (B)).Citation26) If ANGase catalyzes the reaction via the oxocarbenium ion intermediate mechanism, AF is rationally produced (see the Scheme in the dashed square of Fig. and panels C and D) since the H-atom of the C2-H is easily extracted by two possible routes [carboxylate ion-associated mechanism (Fig. (C)) and water molecule-associated mechanism (Fig. (D))], when ring oxygen atom is positively charged. However, this extraction is not likely to be observed via a nucleophilic displacement mechanism, and thus AF would not be expected to be formed (Fig. (A)), suggesting that ANGase follows the oxocarbenium ion intermediate mechanism. Further investigations using other GH enzymes are currently in progress in an effort to confirm the reaction mechanism responsible for AF production.
Fig. 3. Reaction models for the hydrolysis of a maltooligosaccharide catalyzed by ANGase via a nucleophilic displacement mechanism (A) and oxocarbenium ion intermediate mechanism (B) together with the possible two routes for production of AF (C, carboxylate ion-associated route; D, water molecule-associated route).
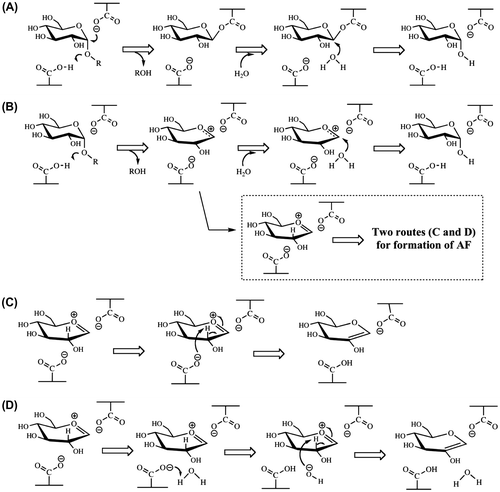
Acknowledgments
We used the LC-MS-MS system of the Research Faculty of Agriculture, Hokkaido University.
References
- Yu S. α-1,4-Glucan lyase, a new starch processing enzyme for production of 1,5-anhydro-d-fructose. Zuckerindustrie. 2004;129:26–30.
- Yu S, Kenne L, Pederśen M. α-1,4-Glucan lyase, a new class of starch/glycogen degrading enzyme. I. Efficient purification and characterization from red seaweeds. Biochim. Biophys. Acta. 1993;1156:313–320.10.1016/0304-4165(93)90049-E
- Yu S, Pederśen M. α-1,4-Glucan lyase, a new class of starch/glycogen-degrading enzyme. II. Subcellular localization and partial amino acid sequence. Planta. 1993;191:137–142.
- Yoshinaga K, Fujisue M, Abe J, Hanashiro I, Takeda Y, Muroya K, Hizukuri S. Characterization of exo-(1,4)-α glucan lyase from red alga Gracilaria chorda. Activation, inactivation and the kinetic properties of the enzyme. Biochim. Biophys. Acta. 1999;1472:447–454.10.1016/S0304-4165(99)00147-6
- Hirano K, Ziak M, Kamoshita K, Sukenaga Y, Kametani S, Shiga Y, Roth J, Akanuma H. N-Linked oligosaccharide processing enzyme glucosidase II produces 1,5-anhydrofructose as a side product. Glycobiology. 2000;10:1283–1289.10.1093/glycob/10.12.1283
- Kametani S, Shiga Y, Akanuma H. Hepatic production of 1,5-anhydrofructose and 1,5-anhydroglucitol in rat by the third glycogenolytic pathway. Eur. J. Biochem. 1996;242:832–838.10.1111/ejb.1996.242.issue-3
- Yu S, Christensen TMIE, Kragh KM, Bojsen K, Marcussen J. Efficient purification, characterization and partial amino acid sequencing of two α-1,4-glucan lyases from fungi. Biochim. Biophys. Acta. 1997;1339:311–320.10.1016/S0167-4838(97)00014-9
- Henrissat B. A classification of glycosyl hydrolases based on amino acid sequence similarities. Biochem. J. 1991;280:309–316.
- Henrissat B, Bairoch A. New families in the classification of glycosyl hydrolases based on amino acid sequence similarities. Biochem. J. 1993;293:781–788.
- Henrissat B, Bairoch A. Updating the sequence-based classification of glycosyl hydrolases. Biochem. J. 1996;316:695–696.
- Kang MS, Okuyama M, Mori H, Kimura A. The first α-1,3-glucosidase from bacterial origin belonging to glycoside hydrolase family 31. Biochimie. 2009;91:1434–1442.10.1016/j.biochi.2009.07.018
- Ernst HA, Lo Leggio L, Willemoës M, Leonard G, Blum P, Larsen S. Structure of the Sulfolobus solfataricus α-glucosidase: Implications for domain conservation and substrate recognition in GH31. J. Mol. Biol. 2006;358:1106–1124.10.1016/j.jmb.2006.02.056
- Lovering AL, Lee SS, Kim YW, Withers SG, Strynadka NC. Mechanistic and structural analysis of a family 31 α-glycosidase and its glycosyl-enzyme intermediate. J. Biol. Chem. 2005;280:2105–2115.
- Okuyama M, Okuno A, Shimizu N, Mori H, Kimura A, Chiba S. Carboxyl group of residue Asp647 as possible proton donor in catalytic reaction of α-glucosidase from Schizosaccharomyces pombe. Eur. J. Biochem. 2001;268:2270–2280.10.1046/j.1432-1327.2001.02104.x
- Yu S, Bojsen K, Svensson B, Marcussen J. α-1,4-Glucan lyases producing 1,5-anhydro-d-fructose from starch and glycogen have sequence similarity to α-glucosidases. Biochim. Biophys. Acta. 1999;1433:1–15.10.1016/S0167-4838(99)00152-1
- Lee SS, Yu S, Withers SG. Detailed dissection of a new mechanism for glycoside cleavage: α-1,4-glucan lyase. Biochemistry. 2003;42:13081–13090.10.1021/bi035189g
- Kita A, Matsui H, Somoto A, Kimura A, Takata M, Chiba S. Substrate specificity and subsite affinities of crystalline α-glucosidase from Aspergillus niger. Agric. Biol. Chem. 1991;55:2327–2335.10.1271/bbb1961.55.2327
- Tagami T, Okuyama M, Nakai H, Kim YM, Mori H, Taguchi K, Svensson B, Kimura A. Key aromatic residues, at subsites +2 and +3 of glycoside hydrolase family 31 α glucosidase contribute to recognition on long-chain substrates. Biochim. Biophys. Acta. 2013;1834:329–335.10.1016/j.bbapap.2012.08.007
- Yu S, Olsen CE, Marcussen J. Methods for the assay of 1,5-anhydro-d-fructose and α-1,4-glucan lyase. Carbohydr. Res. 1997;305:73–82.10.1016/S0008-6215(97)00226-7
- Yu S, Ahmad T, Kenne L, Pederśen M. α-1,4-Glucan lyase, a new class of starch/glycogen degrading enzyme. III. Substrate specificity, mode of action, and cleavage mechanism. Biochim. Biophys. Acta. 1995;1244:1–9.10.1016/0304-4165(94)00202-9
- Miwa I, Okuda J, Maeda K, Okuda G. Mutarotase effect on colorimetric determination of blood glucose with β-d-glucose oxidase. Clin. Chim. Acta. 1972;37:538–540.10.1016/0009-8981(72)90483-4
- Kametani S, Mizuno H, Shiga Y, Akanuma H. NMR of all-carbon-13 sugars: An application in development of an analytical method for a novel natural sugar, 1,5-anhydrofructose. J. Biochem. 1996;119:180–185.10.1093/oxfordjournals.jbchem.a021206
- Kühn A, Yu S, Giffhorn F. Catabolism of 1,5-anhydro-d-fructose in Sinorhizobium morelense S-30.7.5: Discovery, characterization, and overexpression of a new 1,5-anhydro-d-fructose reductase and its application in sugar analysis and rare sugar synthesis. Appl. Environ. Microbiol. 2006;72:1248–1257.10.1128/AEM.72.2.1248-1257.2006
- Andersen SM, Jensen HM, Yu S, Ascopyrone P. Chemical synthesis from d-glucose. J. Carbohydr. Chem. 2002;21:569–578.10.1081/CAR-120016855
- Kim YM, Saburi W, Yu S, Nakai H, Maneesan J, Kang MS, Chiba S, Kim D, Okuyama M, Mori H, Kimura A. A novel metabolic pathway for glucose production mediated by α-glucosidase-catalyzed conversion of 1,5-anhydrofructose. J. Biol. Chem. 2012;287:22441–22444.10.1074/jbc.C112.360909
- Chiba S. A historical perspective for the catalytic reaction mechanism of glycosidase; so as to bring about breakthrough in confusing situation. Biosci. Biotechnol. Biochem. 2012;76:215–231.10.1271/bbb.110713