Abstract
3-deoxysilybin (3-DS), also known as (–)-isosilandrin A, is a natural flavonoid of Silybum marianum. This study was designed to investigate the anti-inflammatory effect and the underlying molecular mechanisms of 3-DS in lipopolysaccharide (LPS)-stimulated RAW264.7 macrophages. 3-DS dose-dependently inhibited the production of NO and the expression of iNOS in LPS-stimulated RAW264.7 macrophages. 3-DS also inhibited the production of pro-inflammatory cytokines (MCP-1, TNF-α, IL-6, and IL-1β) in LPS-stimulated RAW264.7 macrophages. Moreover, 3-DS decreased the NF-κB DNA binding activity in LPS-stimulated RAW264.7 macrophages. Furthermore, 3-DS suppressed NF-κB activation by inhibiting the degradation of IκBα and nuclear translocation of p65 subunit of NF-κB in LPS-stimulated RAW264.7 macrophages. Taken together, the present study suggests for the first time that 3-DS may exhibit an anti-inflammatory effect through the suppression of NF-κB transcriptional activation in LPS-stimulated RAW264.7 macrophages.
Graphical Abstract
Possible model for anti-inflammatory effect by 3-deoxysilybin in RAW264.7 macrophages.
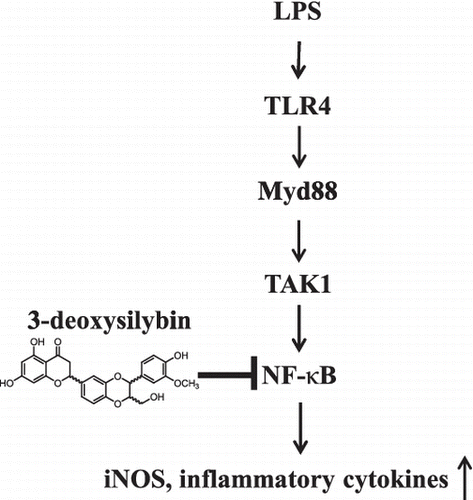
Key words:
Inflammation is the normal protective response against many pathophysiological conditions including trauma, infection, tissue injury, or noxious stimuli.Citation1) Lipopolysaccharide (LPS), a cell wall component of Gram-negative bacteria, induces Toll-like receptor 4 (TLR4)-mediated innate immune response by activating immune cells such as macrophages.Citation2) LPS-stimulated macrophages produce pro-inflammatory cytokines including tumor necrosis factor-α (TNF-α), interleukin-6 (IL-6), interleukin-1β (IL-1β), and other inflammatory mediators such as nitric oxide (NO), inducible nitric oxide synthase (iNOS), cyclooxygenase-2 (COX-2), and prostaglandin E2 (PGE2).Citation3) These inflammatory mediators are activated by nuclear factor-κB (NF-κB)-dependent pathway in LPS-stimulated macrophages.Citation3,4) Thus, many studies have documented that pharmacological inhibition of LPS-induced NF-κB activation is considered as an important target for effective treatment in inflammatory diseases.Citation3,5,6)
Silybum marianum (milk thistle) has been used as a hepatoprotective drug for a long time.Citation7) Various studies indicate that silymarin (milk thistle extract) exhibits anticancer, anti-inflammatory, and antioxidant effects.Citation8) Moreover, silymarin contains a number of biological active compounds including silybin, isosilybin, silandrin, 2,3-dehydrosilybin, and 3-deoxysilybin.Citation7,9,10) Silybin, a major active constituent of silymarin, is used clinically in Europe and Asia as a therapeutic drug due to its antioxidant activity. 2,3-dehydrosilybin has also been reported to exhibit anticancer, antioxidant, and hepatoprotective effects.Citation9,11) In addition, previous study has shown that 3-deoxysilybin, also known as (–)-isosilandrin A, isolated from S. marianum possesses stronger antioxidant activity than silybin.Citation12) However, the effect of 3-deoxysilybin on anti-inflammatory activities has not yet been investigated. Therefore, in the present study, we evaluated the anti-inflammatory effects of 3-deoxysilybin in LPS-stimulated RAW264.7 macrophages.
Materials and methods
Materials
Silybin, LPS, Griess reagent, NP40 cell lysis buffer, protease inhibitor cocktail, and NuCLEAR Extraction Kit were purchased from Sigma-Aldrich (St. Louis, MO, USA). Antibodies for β-Tubulin, IκBα, NF-κB p65, and lamin B were purchased from Santa Cruz Biotechnology (Santa Cruz, CA, USA). Antibody for iNOS was purchased from BD Pharmingen (San Diego, CA, USA). Antibodies for phosphor-IκBα were purchased from Cell Signaling Technology (Danvers, MA, USA). Goat anti-rabbit and mouse IgG HRP-conjugated antibody, Opti-MEM I medium, and Lipofectamine 2000 were purchased from Invitrogen (Carlsbad, CA, USA). The pNF-κB-Luc and pAP-1-Luc reporter vectors were purchased from Stratagene (La Jolla, CA, USA) and Panomics (Fremont, CA, USA), respectively. The pRL-TK internal control vector was purchased from Promega (Madison, WI, USA). Enzyme-linked immunosorbent assay (ELISA) kits for MCP-1, TNF-α, IL-6, and IL-1β were purchased from R&D Systems (Minneapolis, MN, USA). NF-κB transcription factor assay kit was purchased from Abcam (Cambridge, MA, USA).
Preparation of 3-deoxysilybin
3-deoxysilybin (3-DS) used in the present study was prepared by radiation as described previouslyCitation13) and its structure is shown in Fig. . Briefly, irradiation was carried out at ambient temperature, using a cobalt-60 irradiator (point source AECL, IR-79, MDS Nordion International Co. Ltd, Ottawa, ON, Canada) in the Advanced Radiation Technology Institute, Korea Atomic Energy Research Institute (Jeongup, Korea). The source strength was approximately 320 kCi, with dose rate of 10 kGy/h at the location of the sample. Dosimetry was performed using 5 mm diameter alanine dosimeters (Bruker Instruments, Rheinstetten, Germany). The dosimeters were calibrated against an International Standard Set by the International Atomic Energy Agency (Vienna, Austria). Sample solutions (1 g silybin in 1000 mL MeOH in 10% DMSO) in chapped vials were irradiated with 300 kGy (absorbed dose). After irradiation, sample solution was immediately evaporated to remove the solvent and lyophilized. This gamma ray-irradiated sample solution was performed by MPLC using a reversed-phase silica gel cartridge column [MeOH−water, 4:6 (30 min), 5:5 (40 min), 10:0 (20 min); flow rate: 20 mL/min] to give three fractions (A−C). Fraction B (320 mg) was purified over Sephadex LH-20 (Pharmacia Biotech AB, Uppsala, Sweden) with 80% CH3OH as eluent, yielding 3-deoxysilybin (yellowish powder, 335 mg).
Cell culture
RAW264.7 cells (murine macrophage line) were purchased from American Type Culture Collection (Manassas, VA, USA). The cells were grown in DMEM supplemented with 10% fetal bovine serum (Hyclone, Logan, UT, USA), 100 units/mL of penicillin, and 100 μg/mL of streptomycin (Invitrogen, Carlsbad, CA, USA) in a humidified atmosphere at 37 °C with 5% CO2.
Cytotoxicity assay
Cell viability was measured by an EZ-Cytox cell viability assay kit (DAEIL lab, Seoul, Korea) according to the manufacturer’s instruction. RAW264.7 cells were cultured in a 96-well plate at a density of 2 × 105 cells/mL for 20 h. The cells were subsequently treated with various concentrations of 3-DS (0, 5, 10, 20, 40, 60, 80, and 100 μM). After incubation for 24 h, 10 μL of the kit solution was added to each well and further incubated for 4 h at 37 °C and 5% CO2. The index of cell viability was determined by measuring formazan production with an ELISA reader (Benchmark Plus, Bio-Rad, Hercules, CA, USA) at an absorbance of 480 nm. The reference wavelength was 650 nm. Cell viability was determined relative to untreated control cells.
Measurement of NO, MCP-1, TNF-α, IL-6, and IL-1β production
RAW264.7 cells were cultured in a 96-well plate at a density of 2 × 105 cells/mL for 20 h. After incubation, the cells were pretreated with various concentrations of 3-DS (5, 10, 20, and 40 μM) for 1.5 h and were treated with 1 μg/mL of LPS for an additional 18 h. The culture media were collected at the end of the culture period for the NO and cytokines assay. For a nitrite assay, the culture media (100 μL) was mixed with an equal volume of Griess reagent (Sigma-Aldrich) in a 96-well plate and then incubated for 15 min at room temperature. The absorbance at 540 nm was measured, and the concentration of nitrite was calculated using a calibration standard curve constructed using sodium nitrite dissolved in DMEM. The concentrations of MCP-1, TNF-α, IL-6, and IL-1β in culture media were measured using an ELISA kit (R&D Systems) according to the manufacturer’s instruction. The results are presented as the mean ± SD of three replicates from one representative experiment.
Preparation of total proteins
RAW264.7 cells were cultured in a 100 mm dish at a density of 2 × 105 cells/mL for 24 h. After incubation, the cells were pretreated with various concentrations of 3-DS (10, 20, and 40 μM) for 1.5 h and were treated with 1 μg/mL of LPS for 18 h or 20 min. The cells were harvested and lysed with NP40 cell lysis buffer in the presence of a protease inhibitor cocktail (Sigma-Aldrich) and PMSF (Sigma-Aldrich). The samples were incubated for 30 min on ice and then centrifuged at 12,000 rpm for 15 min at 4 °C. The cell extracts were collected and stored at –80 °C until used for further studies. The protein contents were determined by the Bio-Rad Protein Assay (Bio-Rad)
Preparation of nuclear proteins
RAW264.7 cells were cultured in a 100 mm dish at a density of 2 × 105 cells/mL for 20 h. After incubation, the cells were pretreated with various concentrations of 3-DS (10, 20, and 40 μM) for 1.5 h and were treated with 1 μg/mL of LPS for 20 min. The cells were harvested and nuclear and cytosolic fractions were prepared using a NuCLEAR Extraction Kit (Sigma-Aldrich) according to the manufacturer’s instruction.
Western blotting
After quantification of protein contents, equal amounts of protein were resolved on SDS-polyacrylamide gels and then transferred to nitrocellulose membranes (Hybond ECL Nitrocellulose; Amersham Biosciences, Bucks, UK). The membranes were blocked with 5% skim milk and incubated with the primary antibodies in 10 mL of buffer (Tris-buffered saline and 0.1% Tween 20 with 5% skim milk) with gentle shaking at 4 °C overnight. After incubation, the membranes were washed, incubated with HRP conjugated secondary antibody for 2 h at room temperature, and then washed again. The blotted proteins were detected using an enhanced chemiluminescence detection system (GE Healthcare, Bucks, UK).
Luciferase assay
RAW264.7 cells were cultured in a six-well plate at a density of 4 × 105 cells/mL for 20 h. The cells were transfected with the pNF-κB-Luc, the pAP-1-Luc reporter vector, and the pRL-TK internal control vector using Lipofectamine 2000 (Invitrogen) according to the manufacturer’s instruction. After transfection, the cells were pretreated with 20 and 40 μM of 3-DS for 1.5 h and were treated with 1 μg/mL of LPS. After 24 h of stimulation, the cells were then collected, lysed, and luciferase activity was measured using the Dual-luciferase Reporter Assay System (Promega) and luminometer (Berthold Technologies, Bad Wildbad, Germany).
NF-κB ELISA assay
After nuclear extraction, NF-κB DNA binding activity was determined using NF-κB transcription factor assay kit (Abcam) according to the manufacturer’s instruction. Briefly, nuclear extracts (10 μg) and complete transcription factor binding assay buffer were added in plate wells coated with a NF-κB consensus dsDNA sequence and incubated at 4 °C overnight without agitation. Each well was washed with wash buffer five times and incubated with the NF-κB primary antibody (1:100) at room temperature for 1 h without agitation. The wells were washed, incubated with HRP conjugated secondary antibody (1:100) for 1 h at room temperature without agitation, and then washed again. After incubation, developing solution was added to each well and incubated for 15 min at room temperature with gentle agitation protected from light, and then the developing reaction was stopped by adding stop solution. The absorbance at 450 nm was measured, and the NF-κB DNA binding activity was determined relative to untreated control cells.
Immunofluorescence assay
RAW264.7 cells were cultured in sterile cover slides and pretreated with 40 μM of 3-DS for 1.5 h and treated with 1 μg/mL of LPS for 20 min. The cells were then rinsed with PBS and fixed in methanol for 15 min at room temperature. The fixed cells were permeabilized with 0.3% Triton X-100 in PBS for 15 min and blocked with 0.5% BSA in PBS for 1 h at room temperature. After washing with PBS, the cells were incubated overnight at 4 °C with a rabbit anti-p65 antibody (1:100). Next, Alexa Fluor 488-conjugated secondary antibody (1:200; Molecular Probes, Eugene, OR, USA) was applied for 1 h at room temperature. The cells were washed with PBS, and then stained with 1 μg/mL of bis-benzimide staining solution for 10 min at room temperature. After washing with PBS, the cover slides were finally mounted and observed by confocal microscopy (LSM 510 META; Carl Zeiss, Jena, Germany).
Statistical analysis
All data are presented as the mean ± SD. The data analysis was performed using the SAS program (Statistical analysis system V9.3, SAS Institute Inc., Cary, NC, USA). Differences between the variables were reported using one-way analysis of variance. Duncan’s multiple range test (p value < 0.05) was used to compare the mean values.
Results
Effect of 3-DS on NO production and iNOS expression levels in LPS-stimulated RAW264.7 cells
First, we determined cell cytotoxicity of 3-DS against RAW264.7 cells. The cells were treated with various concentrations of 3-DS (0, 5, 10, 20, 40, 60, 80, and 100 μM) for 24 h, and cell cytotoxicity was determined using the EZ-Cytox cell viability assay kit. As shown in Fig. (A), 3-DS did not exhibit cytotoxicity to RAW264.7 cells up to 40 μM but did inhibit cell viability at the doses of 60–100 μM. Therefore, treatments in all experiments used doses of 3-DS up to 40 μM. To investigate the potential anti-inflammatory effects of 3-DS, RAW264.7 cells were pretreated with 3-DS (5, 10, 20, and 40 μM) to detect NO production. LPS treatment significantly increased the production of NO by approximately fourfold over basal levels in RAW264.7 cells. However, the LPS-induced NO production was significantly reduced by 3-DS in a dose-dependent manner (Fig. (B)). Western blot was performed to determine whether the inhibitory effects of 3-DS on NO production were related to the expression of iNOS (Fig. (C)). Pretreatment with 3-DS (10, 20, and 40 μM) remarkably decreased the expression of iNOS protein in RAW264.7 cells. These results suggested that 3-DS decreased NO production by inhibiting the expression of iNOS.
Fig. 2. Effect of 3-DS on cell viability (A), NO production (B), and iNOS expression levels (C) in LPS-stimulated RAW264.7 macrophages.
Notes: (A) RAW264.7 cells were treated with 3-DS (0, 5, 10, 20, 40, 60, 80, and 100 μM) for 24 h, and the relative cell viability was assessed by EZ-Cytox cell viability assay. (B) RAW264.7 cells were pretreated with 3-DS (5, 10, 20, and 40 μM) for 1.5 h and then incubated for 18 h with LPS (1 μg/mL). The culture supernatant was subjected to a nitrite assay. Data were expressed as mean ± SD. Means with same alphabet (a–f) are not significantly different at p < 0.05. (C) The iNOS expression levels were determined by western blotting.
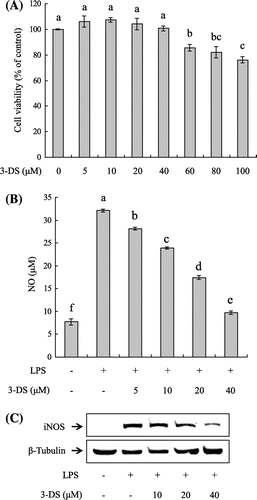
Effect of 3-DS on MCP-1, TNF-α, IL-6, and IL-1β production in LPS-stimulated RAW264.7 cells
To determine the effects of 3-DS on the production of the pro-inflammatory chemokine MCP-1 and cytokines (TNF-α, IL-6, and IL-1β), RAW264.7 cells were pretreated with various concentrations of 3-DS (10, 20, and 40 μM) and stimulated with 1 μg/mL of LPS for 18 h. The pro-inflammatory chemokine MCP-1 and cytokines (TNF-α, IL-6, and IL-1β) in the culture media were measured by ELISA kits. As shown in Fig. , the levels of MCP-1, TNF-α, IL-6, and IL-1β were significantly increased in the LPS-stimulated RAW264.7 cells when compared with the untreated cells. However, pretreatment of 3-DS markedly decreased the levels of MCP-1, TNF-α, IL-6, and IL-1β in a dose-dependent manner.
Fig. 3. 3-DS inhibits LPS-induced MCP-1 (A), TNF-α (B), IL-6 (C), and IL-1β (D) production in RAW264.7 macrophages.
Notes: RAW264.7 cells were pretreated with 3-DS (10, 20, and 40 μM) for 1.5 h and then incubated for 18 h with LPS (1 μg/mL). The culture supernatant was subjected to ELISA. Data were expressed as mean ± SD. Means with same alphabet (a–d) are not significantly different at p < 0.05.
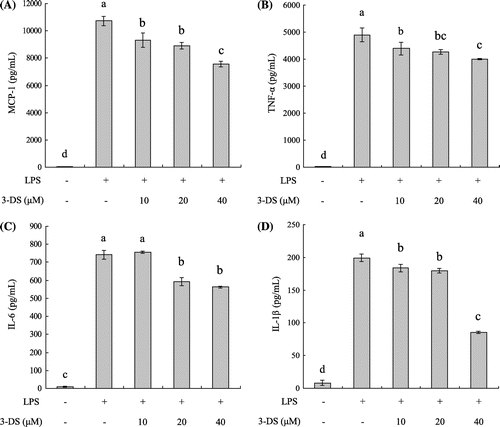
Effect of 3-DS on NF-κB transcriptional and DNA binding activity in LPS-stimulated RAW264.7 cells
To analyze the molecular mechanism underlying the anti-inflammatory effects of 3-DS, we examined the NF-κB activation using a luciferase reporter gene assay and ELISA assay. To investigate the effect of 3-DS on NF-κB transcriptional activity, RAW264.7 cells were transiently transfected with the pNF-κB-Luc plasmid (containing repeats of NF-κB recognition sequences) and pRL-TK plasmid (containing cDNA encoding Renilla luciferase). As shown in Fig. (A), NF-κB reporter activity was significantly increased in the LPS-treated cells when compared with the untreated cells. However, NF-κB reporter activity was significantly decreased by 3-DS in a dose-dependent manner. Moreover, ELISA assay showed that 3-DS significantly decreased the LPS-induced elevation of NF-κB DNA binding activity in a dose-dependent manner (Fig. (B)). These results suggested that 3-DS might suppress the production of pro-inflammatory mediators such as NO, iNOS, MCP-1, TNF-α, IL-6, and IL-1β through the attenuation of NF-κB activation.
Fig. 4. 3-DS inhibits LPS-induced NF-κB activation in RAW264.7 macrophages.
Notes: (A) RAW264.7 cells were transiently transfected with pNF-κB-Luc and pRL-TK vector. The cells were pretreated with 3-DS (20 and 40 μM) for 1.5 h and then incubated for 24 h with LPS (1 μg/mL). The NF-κB luciferase activity was determined by dual-luciferase reporter assay. (B) RAW264.7 cells were pretreated with 3-DS (10, 20, and 40 μM) for 1.5 h and then incubated for 20 min with LPS (1 μg/mL). The nuclear proteins were prepared and the NF-κB DNA binding activity were analyzed by ELISA assay. Data were expressed as mean ± SD. Means with same alphabet (a–d) are not significantly different at p < 0.05.
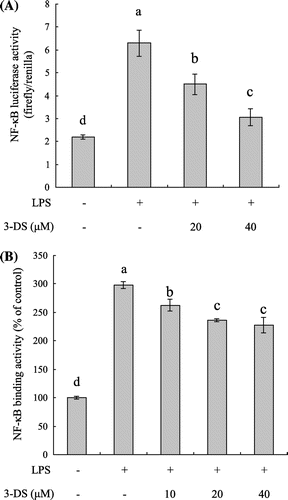
Effect of 3-DS on degradation of IκB-α and nuclear translocation of p65 in LPS-stimulated RAW264.7 cells
To further investigate the molecular mechanisms of 3-DS on anti-inflammatory effect, we investigated nuclear translocation of p65 subunit of NF-κB and degradation of IκB-α. As shown in Fig. (A) and (B), the nuclear translocation of p65 was increased by LPS treatment in RAW264.7 cells. However, these translocations were inhibited by pretreatment of 3-DS, as evidenced by immunofluorescence and western blot analysis. In addition, LPS treatment markedly induced the degradation and phosphorylation of cytosolic IκB-α in RAW264.7 cells. However, these effects were inhibited by pretreatment of 3-DS in a dose-dependent manner (Fig. (C)). These results strongly suggested that 3-DS prevented the nuclear translocation of NF-κB p65 by blocking the degradation and phosphorylation of IκB-α.
Fig. 5. 3-DS inhibits LPS-induced nuclear translocation of NF-κB p65 and degradation of IκB-α in RAW264.7 macrophages.
Notes: (A) RAW264.7 cells were pretreated with 40 μM of 3-DS for 1.5 h and then incubated for 20 min with LPS (1 μg/mL). Translocation of p65 was determined using a NF-κB p65 antibody and an Alexa Fluor 488-conjugated anti-rabbit IgG antibody. Nuclei were counterstained by Hoechst 33258. Scale bar = 10 μm. (B and C) RAW264.7 cells were pretreated with 3-DS (10, 20, and 40 μM) for 1.5 h and then incubated for 20 min with LPS (1 μg/mL). The nuclear and cytosolic proteins were prepared, and then the p65 nuclear translocation and IκB-α degradation levels were determined by western blotting.
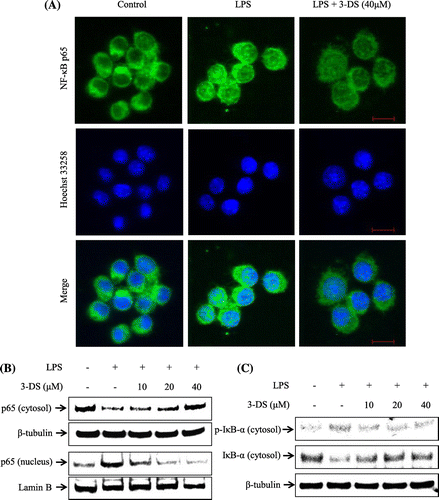
Discussion
The objective of the present study was to evaluate the anti-inflammatory effects of 3-deoxysilybin in LPS-stimulated RAW264.7 macrophages. In this study, we showed that 3-deoxysilybin inhibited the induction of the pro-inflammatory mediators such as NO, iNOS, MCP-1, TNF-α, IL-6, and IL-1β through suppression of NF-κB activation.
NO mediates the numerous physiological responses including blood pressure regulation, renal function, gastrointestinal function, wound healing, and nonspecific immunity. NO is produced by nitric oxide synthase (NOS) which catalyze the 5-electron oxidation of L-arginine to L-citrulline and NO. NOS exists three NOS isoforms, neuronal NOS (nNOS), endothelial NOS (eNOS), and inducible NOS (iNOS). The nNOS and eNOS isoforms are constitutively expressed and continuously release low levels of NO, whereas iNOS isoform is induced by cytokines or LPS and produces high levels of NO.Citation14) Our results indicated that 3-DS inhibited the LPS-induced iNOS expression and NO production in RAW264.7 macrophage cells. Recent studies have found that flavonoids such as 7,8-dihydroxyflavone, avicularin suppressed LPS-induced iNOS expression and NO production in RAW264.7 macrophage cells.Citation6,15) Therefore, we suggest that pretreatment with 3-DS suppress NO production by reducing iNOS expression in LPS-stimulated RAW264.7 macrophage cells.
LPS-stimulated macrophages have been known to secrete pro-inflammatory cytokines and chemokines to induce inflammatory responses. Pro-inflammatory cytokines such as TNF-α, IL-6, and IL-1β cause the iNOS expression and NO production through the NF-κB-dependent pathway.Citation4,16,17) A previous report has documented that silibinin inhibits LPS-induced production of TNF-α and IL-1β in RAW264.7 cells.Citation18) Sargachromanol G isolated from Sargassum siliquastrum has been demonstrated to suppress LPS-induced TNF-α, IL-6, and IL-1β production in RAW264.7 cells.Citation19) It has also been reported that arctigenin inhibits LPS-induced IL-6, IL-1β, and MCP-1 production in RAW264.7 cells.Citation20) Consistent with previous reports, we found that 3-DS suppressed the LPS-induced production of MCP-1, TNF-α, IL-6, and IL-1β in RAW264.7 macrophage cells, suggesting that 3-DS may be used as an effective natural anti-inflammatory agent. Furthermore, our results indicated that 3-DS most strongly inhibited the IL-1β production in LPS-treated RAW264.7 cells. IL-1β has known as a pathogenic mediator in inflammatory disorders including atopic dermatitis. Caspase-1 induces the maturation of IL-1β from inactive precursor to active form in macrophages and activated IL-1β promotes inflammation.Citation21) Thus, the result of Fig. (D) suggests that 3-DS might be useful in the IL-1β/caspase-1 suppression.
NF-κB is activated by a variety of factors such as LPS, TNF-α, double-stranded RNA, viral proteins, ionizing radiation, ultraviolet light, and reactive oxygen species and regulates numerous pathological conditions including inflammation.Citation14) LPS binds to macrophage surface molecule CD14 and induces TLR4 activation to start inflammation-associated signaling pathways.Citation4) TLR4 activation mediates MyD88- and TRIF-dependent signaling pathways and in turn induces IκB degradation and phosphorylation and subsequently results in the translocation of NF-κB into the nucleus. Finally, LPS-mediated NF-κB activation produces the pro-inflammatory mediators such as NO, cytokines, and chemokines.Citation14,22) In the present study, we found that pretreatment with 3-DS significantly decreased LPS-induced IκB phosphorylation and degradation and nuclear translocation of p65 in RAW264.7 cells. In addition, 3-DS significantly suppressed the NF-κB luciferase and DNA binding activities in LPS-stimulated RAW264.7 cells. Thus, the present study suggests that 3-DS inhibited the production of pro-inflammatory cytokines and mediators through the suppression of NF-κB-dependent pathway. In addition to NF-κB activation, LPS-stimulated inflammatory response is involved in a variety of signaling pathways including mitogen-activated protein kinases (MAPKs), Akt, Nrf2/HO-1 pathway. Many studies have reported that the natural chemical compounds such as β-hydroxyisovalerylshikonin, ethyl linoleate, ampelopsin, and pseudoginsenoside-F11 isolated from plants suppress the LPS-induced inflammation through MAPKs, Akt, Nrf2/HO-1 pathway.Citation23–26) Thus, further studies will be need to understand how 3-DS inhibits LPS-induced inflammation.
Previous study reported that silibinin (silybin) at 50 μg/mL (about 104 μM) indicated the anti-inflammatory effect in LPS-stimulated Raw264.7 cells.Citation18) However, our results showed that 3-DS at 40 μM significantly indicated the anti-inflammatory activity in LPS-stimulated Raw264.7 cells. In addition, it has been known that 3-DS possesses significantly higher antioxidant activity than silybin.Citation12) 3-DS differs structurally from silybin in the fact that 3-DS do not have an hydroxyl group at 3′-position when compared with silybin. This may increase hydrophobic status of 3-DS more than that of silybin and then cause the increase of lipophilicity, which is the ability of a chemical compound to pass through the cell membrane.Citation27,28) Thus, we assume that the enhancement of anti-inflammatory activity of 3-DS may be due to the higher permeation potential than silybin.
In conclusion, the present study demonstrates that 3-DS suppressed the production of pro-inflammatory mediators (NO, iNOS, MCP-1, TNF-α, IL-6, and IL-1β) in LPS-stimulated RAW264.7 macrophages. Furthermore, our results indicate that these effects of 3-DS may be related to the inhibition of NF-κB activation in LPS-stimulated RAW264.7 macrophages. The present study elucidates for the first time that 3-DS exhibits the anti-inflammatory effect. Therefore, this report suggests that 3-DS should be considered as candidate potential anti-inflammatory agents for the treatment of inflammatory diseases.
Acknowledgments
This research was supported by the Ministry of Science, ICT & Future Planning (MSIFP), Republic of Korea.
References
- Choi WS, Shin PG, Lee JH, Kim GD. The regulatory effect of veratric acid on NO production in LPS-stimulated RAW264.7 macrophage cells. Cell. Immunol. 2012;280:164–170.10.1016/j.cellimm.2012.12.007
- Wang Z, Jiang W, Zhang Z, Qian M, Du B. Nitidine chloride inhibits LPS-induced inflammatory cytokines production via MAPK and NF-kappaB pathway in RAW 264.7 cells. J. Ethnopharmacol. 2012;144:145–150.10.1016/j.jep.2012.08.041
- Bharat Reddy D, Reddanna P Chebulagic acid (CA) attenuates LPS-induced inflammation by suppressing NF-κB and MAPK activation in RAW 264.7 macrophages. Biochem. Biophys. Res. Commun. 2009;381:112–117.10.1016/j.bbrc.2009.02.022
- Wun ZY, Lin CF, Huang WC, Huang YL, Xu PY, Chang WT, Wu SJ, Liou CJ. Anti-inflammatory effect of sophoraflavanone G isolated from Sophora flavescens in lipopolysaccharide-stimulated mouse macrophages. Food Chem. Toxicol. 2013;62:255–261.10.1016/j.fct.2013.08.072
- Shin JS, Park YM, Choi JH, Park HJ, Shin MC, Lee YS, Lee KT. Sulfuretin isolated from heartwood of Rhus verniciflua inhibits LPS-induced inducible nitric oxide synthase, cyclooxygenase-2, and pro-inflammatory cytokines expression via the down-regulation of NF-κB in RAW 264.7 murine macrophage cells. Int. Immunopharmacol. 2010;10:943–950.10.1016/j.intimp.2010.05.007
- Park HY, Kim GY, Hyun JW, Hwang HJ, Kim ND, Kim BW, Choi YH. 7,8-Dihydroxyflavone exhibits anti-inflammatory properties by downregulating the NF-κB and MAPK signaling pathways in lipopolysaccharide-treated RAW264.7 cells. Int. J. Mol. Med. 2012;29:1146–1152.
- Shibano M, Lin AS, Itokawa H, Lee KH. Separation and characterization of active flavonolignans of Silybum marianum by liquid chromatography connected with hybrid ion-trap and time-of-flight mass spectrometry (LC-MS/IT-TOF). J. Nat. Prod. 2007;70:1424–1428.10.1021/np070136b
- Ramasamy K, Agarwal R. Multitargeted therapy of cancer by silymarin. Cancer Lett. 2008;269:352–362.10.1016/j.canlet.2008.03.053
- Huber A, Thongphasuk P, Erben G, Lehmann WD, Tuma S, Stremmel W, Chamulitrat W. Significantly greater antioxidant anticancer activities of 2,3-dehydrosilybin than silybin. Biochim. Biophys. Acta. 2008;1780:837–847.10.1016/j.bbagen.2007.12.012
- Nyiredy S, Samu Z, Szucs Z, Gulacsi K, Kurtan T, Antus S. New insight into the biosynthesis of flavanolignans in the white-flowered variant of silybum marianum. J. Chromatogr. Sci. 2008;46:93–96.10.1093/chromsci/46.2.93
- Cho BO, Ryu HW, So Y, Jin CH, Baek JY, Park KH, Byun EH, Jeong IY. Hepatoprotective effect of 2,3-dehydrosilybin on carbon tetrachloride-induced liver injury in rats. Food Chem. 2013;138:107–115.10.1016/j.foodchem.2012.10.026
- Samu Z, Nyiredy S, Baitz-Gacs E, Varga Z, Kurtan T, Dinya Z, Antus S. Structure elucidation and antioxidant activity of (–)-isosilandrin isolated from Silybum marianum L. Chem. Biodivers. 2004;1:1668–1677.10.1002/(ISSN)1612-1880
- Jin CH, Jeong IY, Ryu HW. Preparation method of chromenone derivatives using the radiation. Korea Patent Number 10-2011-0131687, December 09, 2011.
- Billack B. Macrophage activation: role of Toll-like receptors, nitric oxide, and nuclear factor kappa B. Am. J. Pharm. Educ. 2006;70:1–10.
- Vo VA, Lee JW, Chang JE, Kim JY, Kim NH, Lee HJ, Kim SS, Chun W, Kwon YS. Avicularin inhibits lipopolysaccharide-induced inflammatory response by suppressing ERK phosphorylation in RAW 264.7 macrophages. Biomol. Ther. 2012;20:532–537.10.4062/biomolther.2012.20.6.532
- Kim KN, Heo SJ, Yoon WJ, Kang SM, Ahn G, Yi TH, Jeon YJ. Fucoxanthin inhibits the inflammatory response by suppressing the activation of NF-κB and MAPKs in lipopolysaccharide-induced RAW 264.7 macrophages. Eur. J. Pharmacol. 2010;649:369–375.10.1016/j.ejphar.2010.09.032
- Maity B, Yadav SK, Patro BS, Tyagi M, Bandyopadhyay SK, Chattopadhyay S. Molecular mechanism of the anti-inflammatory activity of a natural diarylnonanoid, malabaricone C. Free Radic. Biol. Med. 2012;52:1680–1691.10.1016/j.freeradbiomed.2012.02.013
- Youn CK, Park SJ, Lee MY, Cha MJ, Kim OH, You HJ, Chang IY, Yoon SP, Jeon YJ. Silibinin inhibits LPS-induced macrophage activation by blocking p38 MAPK in RAW 264.7 cells. Biomol. Ther. 2013;21:258–263.10.4062/biomolther.2013.044
- Yoon WJ, Heo SJ, Han SC, Lee HJ, Kang GJ, Kang HK, Hyun JW, Koh YS, Yoo ES. Anti-inflammatory effect of sargachromanol G isolated from Sargassum siliquastrum in RAW 264.7 cells. Arch. Pharm. Res. 2012;35:1421–1430.10.1007/s12272-012-0812-5
- Kou X, Qi S, Dai W, Luo L, Yin Z. Arctigenin inhibits lipopolysaccharide-induced iNOS expression in RAW264.7 cells through suppressing JAK-STAT signal pathway. Int. Immunopharmacol. 2011;11:1095–1102.10.1016/j.intimp.2011.03.005
- Han NR, Kim HM, Jeong HJ. Kanamycin activates caspase-1 in NC/Nga mice. Exp. Dermatol. 2011;20:659–663.10.1111/exd.2011.20.issue-8
- Kawai T, Akira S. TLR signaling. Cell Death Differ. 2006;13:816–825.10.1038/sj.cdd.4401850
- Jayasooriya RGPT, Lee KT, Lee HJ, Choi YH, Jeong JW, Kim GY. Anti-inflammatory effects of β-hydroxyisovalerylshikonin in BV2 microglia are mediated through suppression of the PI3 K/Akt/NF-kB pathway and activation of the Nrf2/HO-1 pathway. Food Chem. Toxicol. 2014;65:82–89.10.1016/j.fct.2013.12.011
- Park SY, Seetharaman R, Ko MJ, Kim DY, Kim TH, Yoon MK, Kwak JH, Lee SJ, Bae YS, Choi YW. Ethyl linoleate from garlic attenuates lipopolysaccharide-induced pro-inflammatory cytokine production by inducing heme oxygenase-1 in RAW264.7 cells. Int. Immunopharmacol. 2014;19:253–261.10.1016/j.intimp.2014.01.017
- Qi S, Xin Y, Guo Y, Diao Y, Kou X, Luo L, Yin Z. Ampelopsin reduces endotoxic inflammation via repressing ROS-mediated activation of PI3 K/Akt/NF-κB signaling pathways. Int. Immunopharmacol. 2012;12:278–287.10.1016/j.intimp.2011.12.001
- Wang X, Wang C, Wang J, Zhao S, Zhang K, Wang J, Zhang W, Wu C, Yang J. Pseudoginsenoside-F11 (PF11) exerts anti-neuroinflammatory effects on LPS-activated microglial cells by inhibiting TLR4-mediated TAK1/IKK/NF-κB, MAPKs and Akt signaling pathways. Neuropharmacol. 2014;79:642–656.
- Youdim KA, Dobbie MS, Kuhnle G, Proteggente AR, Abbott NJ, Rice-Evans C. Interaction between flavonoids and the blood-brain barrier: in vitro studies. J. Neurochem. 2003;85:180–192.10.1046/j.1471-4159.2003.01652.x
- Pavlica S, Gebhardt R. Protective effects of flavonoids and two metabolites against oxidative stress in neuronal PC12 cells. Life Sci. 2010;86:79–86.10.1016/j.lfs.2009.10.017