Abstract
Obese adipose tissue is characterized by enhanced macrophage infiltration. A loop involving monocyte chemoattractant protein-1 (MCP-1) and tumor necrosis factor-α (TNFα) between adipocytes and macrophages establishes a vicious cycle that augments inflammatory changes and insulin resistance in obese adipose tissue. Tomatoes, one of the most popular crops worldwide, contain many beneficial phytochemicals that improve obesity-related diseases such as diabetes. Some of them have also been reported to have anti-inflammatory properties. In this study, we focused on the potential protective effects of phytochemicals in tomatoes on inflammation. We screened fractions of tomato extract using nitric oxide (NO) assay in lipopolysaccharide (LPS)-stimulated RAW264 macrophages. One fraction, RF52, significantly inhibited NO production in LPS-stimulated RAW264 macrophages. Furthermore, RF52 significantly decreased MCP-1 and TNFα productions. The coculture of 3T3-L1 adipocytes and RAW264 macrophages markedly enhanced MCP-1, TNFα, and NO productions compared with the control cultures; however, the treatment with RF52 inhibited the production of these proinflammatory mediators. These results suggest that RF52 from tomatoes may have the potential to suppress inflammation by inhibiting the production of NO or proinflammatory cytokines during the interaction between adipocytes and macrophages.
Graphical Abstract
Tomato extract suppresses the production of proinflammatory mediators induced by interaction between adipocytes and macrophages.
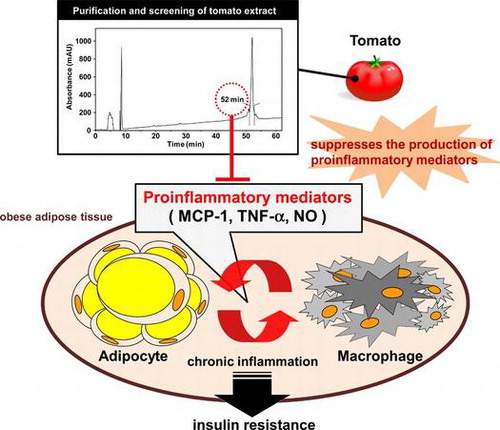
Obesity is a major risk factor for chronic diseases such as cardiovascular diseases, insulin resistance, and type 2 diabetes.Citation1,2) Recent studies have indicated that obesity is characterized by chronic and low-grade inflammation, which can cause increased insulin resistance.Citation3–5) Adipose tissue is a key site for this chronic inflammatory response and obese adipose tissue is characterized by an enhanced infiltration of macrophages.Citation6,7) These macrophages produce various inflammatory cytokines including tumor necrosis factor-α (TNFα) and nitric oxide (NO), which can directly cause insulin resistance.Citation8–10) Furthermore, monocyte chemoattractant protein-1 (MCP-1) is also upregulated in obese adipose tissue,Citation11) suggesting that MCP-1 plays a crucial role in adipose tissue inflammatory responses by inducing the infiltration of macrophages into adipose tissue and their activation. It has been indicated that a paracrine loop involving adipocyte-derived free fatty acid (FFA), MCP-1, and macrophage-derived TNFα establishes a vicious cycle that aggravates inflammatory changes and insulin resistance in obese adipose tissue.Citation12) Therefore, to prevent obesity-related diseases, it is important to suppress the expression of these proinflammatory factors in adipose tissue.
Tomatoes, one of the most popular and extensively consumed crops in the world, contain many beneficial nutrients and phytochemicals suppressing chronic diseases. Many previous studies have shown that the dietary intake of tomatoes is associated with the reduction in the risk of chronic diseases such as cardiovascular diseases, cancer, and type-2 diabetes.Citation13–16) Thus, tomatoes may prevent and ameliorate the obese-related inflammation.
In this study, we investigated the inhibitory effect of tomato extract on the production of proinflammatory mediators including NO, TNFα, and MCP-1 in lipopolysaccharide (LPS)-stimulated macrophages and on the interaction between adipocytes and macrophages.
Materials and methods
Plant materials and chemicals
In this study, we used the Furikoma tomato cultivar, which is widely used in tomato processing. Furikoma tomatoes, from the 2007 harvest, were provided by the Agriculture and Forestry Research Center (Chiba, Japan). All other chemicals used were from Invitrogen Corp. (CA, U.S.A), Nacalai Tesque (Kyoto, Japan), or Wako (Osaka, Japan), and were guaranteed to be of reagent, HPLC, or tissue-culture grade.
Extract preparation and fractionation of crude extract
The extraction and fractionation of tomatoes were performed as previously described.Citation17) Briefly, lipophilic components were extracted from freeze-dried Furikoma powder using ethanol at room temperature for 24 h. Ethanol in the extract was evaporated under vacuum at 40 °C using a rotary evaporator. The crude Furikoma extract was fractionated by reverse-phase HPLC on a 5C18-AR-2 ODS column (6.0 × 150 mm; Nacalai Tesque) using a mobile phase of water (solvent A) and acetonitrile (solvent B) with 0.1% v/v formic acid added to both solvents. The program began with an isocratic step at 30% solvent B in solvent A followed by a linear elution gradient from 30 to 90% solvent B in solvent A for 60 min. To monitor HPLC elution, a diode array detector was used in the range of 200–700 nm. Flow rate was set at 1.0 mL/min. Eluted fractions were collected at 1 mL/min. To determine active compounds, an active fraction (F37) was further separated by reverse-phase HPLC on another column, a TSK-gel column ODS-100 V (4.6 × 250 mm; TOSOH Corp., Tokyo, Japan). The mobile phase, gradient program, and monitor were the same as those in the method using the 5C18-AR-2 ODS column. Flow rate was set at 0.5 mL/min. Eluted fractions were collected at 0.5 mL/min. Solvents in eluted fractions were evaporated under vacuum at 40 °C using a rotary evaporator, and then we examined the effects of fractions redissolved in ethanol at various concentration on the production of the proinflammatory mediators.
Cell culture
Cell culture was performed as previously described.Citation18) Briefly, the RAW264 macrophage cell line (RIKEN BioResource Center, Tsukuba, Japan) was cultured in Dulbecco’s modified Eagle’s medium (DMEM) (Sigma) with 10% fetal bovine serum (FBS) (JRH Bioscience, Kansas, USA) and 100 U/mL penicillin/100 μg/mL streptomycin (Gibco BRL, NY, USA) at 37 °C under a humidified 5% CO2 atmosphere. To measure MCP-1, TNFα, and NO, RAW264 cells were treated with 5 μg/mL LPS (Sigma) and RF52 in serum-free medium for 24 h. 3T3-L1 preadipocytes (American Type Culture Collection, Manassas, VA, USA) were subcultured in DMEM with 10% FBS (Biological Industries, Kibbutz Beit Haemek, Israel), 100 U/mL penicillin/100 μg/mL streptomycin at 37 °C under a humidified 5% CO2 atmosphere. The differentiation of 3T3-L1 preadipocytes was induced using adipogenic agents [0.5 mM 3-isobutyl-1-methylxanthine (Nacalai Tesque, Kyoto, Japan), 0.25 μM dexamethasone (Sigma), and 10 μg/mL insulin (Sigma)] in DMEM containing 10% FBS for 2 days after the cells reached confluence (day 0). Then, the medium was replaced with DMEM containing 10% FBS and 5 μg/mL insulin, which was replaced with a fresh one every two days. Twenty days after the differentiation induction, the cells that accumulated large lipid droplets were used as hypertrophied 3T3-L1 adipocytes.
Coculture of adipocytes and macrophages
Adipocytes and macrophages were cocultured in a contact system as previously described.Citation12) Briefly, RAW264 cells (1 × 105 cells/mL) were plated onto dishes with serum-starved and hypertrophied 3T3-L1 cells, and the coculture was incubated in serum-free DMEM for 24 h. RAW264 and 3T3-L1 cells of equal numbers to those in the coculture were cultured separately as control cultures. RF52 was added to the coculture at various concentrations, as shown in each figure. After 24 h of treatment, culture supernatants were collected and inflammatory mediators were measured as below.
Measurement of inflammatory mediators
The concentrations of MCP-1 and TNFα in the culture supernatants were determined by enzyme-linked immunosorbent assay (ELISA), conducted using a READY-SET-GO mouse MCP-1 and TNFα (eBioscience, CA, USA) according to the manufacturers’ protocol.
The amount of NO in the cell-free culture supernatants was measured using Griess reagent.Citation19) Briefly, 100 μL of supernatant was mixed with an equivalent volume of Griess reagent [1:1 (v/v) of 0.1% N-1-naphthyl-ethylenediamine in distilled water and 1% sulfanilamide in 5% phosphoric acid] on a 96-well flat-bottom plate. After 10 min, absorbance at 550 nm was measured, and the amount of NO was calculated from the NaNO2 standard curve.
Western blotting
RAW264 cells were carefully washed with ice-cold phosphate buffered saline (PBS) and placed immediately in lysis buffer containing 20 mM Tris HCl (pH 7.5), 15 mM NaCl, 1% Triton X100, and protease inhibitor cocktail (Nacalai Tesque). The lysates were centrifuged at 15,000 rpm for 5 min, and supernatants were stored for subsequent analysis. The protein concentration of the cell lysate was determined using DC protein assay reagents (BioRad Laboratories, CA, USA) on the basis of the method of Lowry et al.Citation20) Fifty micrograms of protein/lane was subjected to 10% SDS-PAGE, and separated products were transferred to an Immobilon-P membrane (Millipore, MA, USA). After blocking with 5% skim milk in PBS/0.1% Tween 20, the membrane was incubated with anti-phosphorylated extracellular-signaling-regulated kinase (anti-pERK), anti-ERK, anti-βactin (Cell Signaling, MA, USA) or anti-IκB-α antibody (Santa Cruz Biotechnology, CA, USA) overnight, and then with a secondary antibody conjugated to horseradish peroxidase (anti-rabbit or anti-mouse IgG (Santa Cruz Biotechnology)). The secondary antibody was visualized using chemiluminescence with an ECL Western blotting detection reagent (Amersham Biosciences, NJ, USA).
Cell viability assay
Cells were seeded in 96-well plates (1 × 105 cells/well) and treated with tomato fractions for 24 h. The viability of RAW264 macrophages was measured by CellTiter 96 Aqueous One-Solution Cell Proliferation Assay (Promega).
Statistical analysis
The data are presented as means ± SEM. Data were assessed by one-way ANOVA and Dunnett’s multiple comparison tests. Differences were considered significant when p was <0.05.
Results
Screening of tomato fraction in NO assay
An ethanol extract of Furikoma tomatoes was fractionated by HPLC using an analytical ODS column, and then the inhibitory effects of these fractions on NO production by LPS-stimulated RAW264 macrophages were examined. The NO assay revealed that one fraction, which eluted at 32 and 37 min (F32 and F37, respectively), significantly decreased NO production in LPS-stimulated RAW264 macrophages (Fig. (A) and (B)). F37 had no effect on the viability of RAW264 cells, but F32 decreased cell viability (Fig. (C)). To purify the active compound in F37, we further separated F37 by HPLC using another analytical ODS column and obtained a single peak at 52 min (RF52, Fig. (A)). RF52 significantly inhibited the LPS-induced NO production in RAW264 macrophages (Fig. (B)). Thus, we used RF52 derived from tomato extract to elucidate the potential to suppress the production of proinflammatory mediators.
Fig. 1. Screening of tomato fraction in NO assay. (A) HPLC profile (276 nm) during fractionation of tomato extract. (B) NO production by RAW264 cells stimulated with LPS (5 μg/mL) and incubated with tomato fractions. (C) Viability of RAW264 cells stimulated with LPS (5 μg/mL) and incubated with tomato fractions. Data are presented as means ±SEM (n = 4–5). *p < 0.05 vs. culture treated with LPS alone.
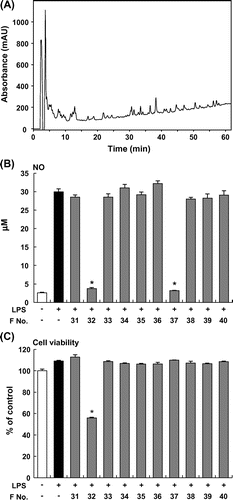
Fig. 2. Purification of tomato fraction in HPLC. (A) HPLC profile (276 nm) during the fractionation of tomato extract. (B) NO production by RAW264 cells stimulated with LPS (5 μg/mL) and incubated with tomato fractions. (C) Viability of RAW264 cells stimulated with LPS (5 μg/mL) and incubated with tomato fractions. Data are presented as means ±SEM (n = 4–5). *p < 0.05 vs. culture treated with LPS alone.
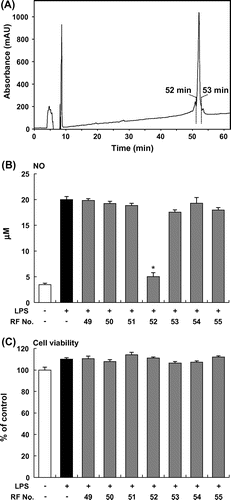
Effects of RF52 on MCP-1 and TNFα production in LPS-stimulated RAW264 macrophages
To determine whether RF52 suppresses MCP-1 and TNFα productions in activated macrophages, RAW264 macrophages were stimulated with LPS in the presence or absence of RF52. The tested RF52 had no effect on the viability of RAW264 cells. RF52 significantly inhibited LPS-induced MCP-1 production by RAW264 cells in a dose-dependent manner (Fig. (A)). RF52 also inhibited LPS-induced TNFα and NO productions (Fig. (B) and (C)).
Fig. 3. Effects of RF52 on secretion of inflammatory mediators by LPS-stimulated RAW264 macrophages.
Notes: RAW264 cells were stimulated with LPS (5 μg/mL) and incubated with RF52 for 24 h. The levels of MCP-1 (A), TNFα (B), and NO (C) in the culture medium were measured. Data are presented as means ±SEM (n = 4–5). **p < 0.01 vs. culture treated with LPS alone.
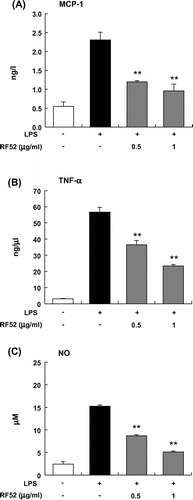
Effects of RF52 on degradation of IκBα and phosphorylation of ERK in macrophages
To clarify the mechanism by which RF52 inhibits LPS-induced inflammation in macrophages, IκBα degradation, which leads to NF-κB activation and ERK phosphorylation were examined in RAW264 macrophages treated with LPS. The LPS treatment for 1 h markedly promoted IκBα degradation in RAW264 macrophages. The degradation was inhibited by RF52 in a dose-dependent manner (Fig. (A)). On the other hand, ERK phosphorylation was enhanced by the LPS treatment. ERK phosphorylation was also inhibited by the RF52 treatment in a dose-dependent manner (Fig. (B)).
Fig. 4. Effects of RF52 on IκBα degradation and ERK phosphorylation in RAW264 macrophages stimulated with LPS.
Notes: RAW264 cells were stimulated with LPS (5 μg/mL) and incubated with RF52 for 1 h. Total cell lysates were extracted from cultured RAW264 cells. Western blotting was conducted using 50 μg of total cell lysate protein/lane, which was reacted with antibodies against (A) IκBα and β-actin, and (B) phosphorylated ERK1/2 and total ERK1/2 (upper band: ERK1, lower band: ERK2). Data are presented as means ±SEM of three replicate experiments. *p < 0.05 vs. culture treated with LPS alone.
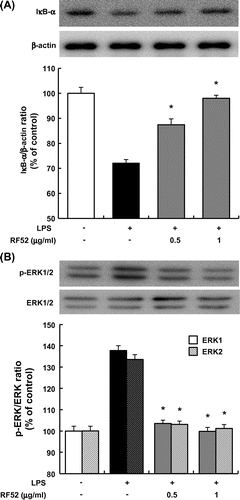
Effects of RF52 on inflammatory changes by coculture of adipocytes and macrophages
MCP-1 secretion by differentiated 3T3-L1 cells or RAW264 cells was very low when the cells were cultured separately; however, the coculture of these cells using a contact system revealed a marked increase in MCP-1 secretion level (Fig. (A)). The RF52 treatment in the coculture significantly decreased MCP-1 level (Fig. (A)). The coculture of differentiated 3T3-L1 adipocytes and RAW264 cells also enhanced TNFα secretion about threefold compared with the control culture (Fig. (B)). The RF52 treatment significantly decreased TNFα secretion level (Fig. (B)). A similar effect of RF52 on NO production was also observed, RF52 dose-dependently suppressed coculture-induced NO production (Fig. (C)).
Fig. 5. Effects of RF52 on inflammatory changes induced by coculture of 3T3-L1 adipocytes and RAW264 macrophages.
Notes: Differentiated 3T3-L1 adipocytes were cocultured with RAW264 macrophages (1 x 105 cells/well) for 24 h. The levels of MCP-1 (A), TNFα (B), and NO (C) in the coculture medium were measured. Data are presented as means ±SEM (n = 4–5). **p < 0.01 vs. nontreated coculture.
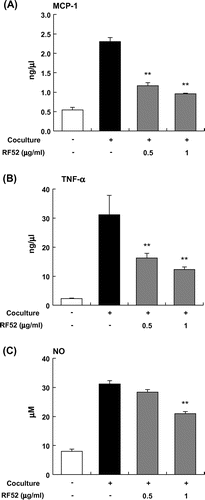
Discussion
In the present study, we demonstrated that RF52 not only suppresses the production of proinflammatory cytokines in activated macrophages, but also inhibits inflammation induced by the interaction between adipocytes and macrophages. This implies that RF52 derived from tomato can inhibit obesity-induced inflammation in adipose tissue. We investigated the structure of compound contained in RF52 using LC-MS. We confirmed that the compound is difficult to ionize under electrospray ionization, suggesting that the compound have little functional group.
Obesity is associated with chronic and low-grade inflammation that can cause insulin resistance in adipose tissue, skeletal muscles, and the liver.Citation20,21) In particular, obese adipose tissue is characterized by an enhanced infiltration of macrophages,Citation6,7) and a paracrine loop involving adipocyte-derived FFA and MCP-1, and macrophage-derived TNFα establishes a vicious cycle that aggravates the inflammation and insulin resistance in adipose tissue.Citation12) Therefore, it is important to inhibit the secretion of these proinflammatory cytokines/chemokines in adipose tissue to prevent the migration of macrophages and the subsequent chronic inflammation. Our data showed that the coculture of hypertrophied 3T3-L1 and RAW264 macrophages resulted in a significant upregulation of the expression of proinflammatory mediators such as MCP-1, TNFα, and NO, which is consistent with the previous report.Citation12) Furthermore, RF52 significantly suppressed the coculture-induced upregulation of MCP-1, TNFα, and NO. These results suggest that RF52 can directly suppress chronic inflammatory responses in obese adipose tissue. However, to determine whether RF52 can inhibit the cytokine paracrine loop in obese adipose tissue, animal experiments using high fat diet-fed mice will be indispensable in the future.
In LPS-induced inflammation, the toll-like receptor-4 (TLR-4) signaling pathway is very important, as TLR-4 is a receptor for LPS. LPS binds to TLR-4 to induce NF-κB- and activator-protein-1 (AP-1)-dependent transcription through IκB degradation and MAPK activation, respectively.Citation22,23) NF-κB and AP-1 synergistically stimulate the production of proinflammatory cytokines including TNFα and MCP-1. Consistent with these reports, our data revealed that RF52 inhibited both IκB degradation and ERK phosphorylation in the LPS-stimulated macrophages, thereby inhibiting the production of these proinflammatory cytokines. In obese adipose tissue, FFA released from adipocytes by triglyceride lipolysis exerts its proinflammatory effect on macrophages through the TLR-4 activation,Citation22) and the inflammatory interaction between adipocytes and macrophages results in the activation of both NF-κB and ERK.Citation12,24) A previous study demonstrated that inflammatory changes induced by the coculture of adipocytes and macrophages are significantly abolished by inhibiting NF-κB and MAP kinases.Citation12,24) In this study, RF52 suppressed the inflammatory interaction between adipocytes and macrophages. These results suggest that RF52 attenuates coculture-induced inflammatory changes in macrophages via the suppression of both IκB/NF-κB and ERK signaling pathways.
In summary, we found that RF52, derived from tomato extract, suppresses the production of proinflammatory mediators by activated macrophages and/or adipocytes cocultured with activated macrophages. These findings suggest that tomatoes contain a compound that inhibit the secretion of NO or other proinflammatory mediators induced by interaction between adipocytes and macrophages. Consequently, tomatoes may have the potential to suppress the vicious cycle of chronic inflammation in obese adipose tissue.
Acknowledgments
The authors thank Sayoko Shinotoh for secretarial assistance and Manami Sakai for technical assistance.
Notes
Abbreviations: ECL, enhanced chemiluminescence; F37, fraction eluted in 37 min; FBS, fetal bovine serum; LPS, lipopolysaccharide; MCP-1, monocyte chemoattractant protein-1; NO, nitric oxide; ODS, octa decyl silyl; RF52, rechromatographed-fraction eluted in 52 min; TNFα, tumor necrosis factor-α
References
- Reilly MP, Rader DJ. The metabolic syndrome: more than the sum of its parts? Circulation. 2003;108:1546–1551.10.1161/01.CIR.0000088846.10655.E0
- Miranda PJ, DeFronzo RA, Califf RM, Guyto JR. Metabolic syndrome: definition, pathophysiology, and mechanism. Am. Heart J. 2005;149:33–45.10.1016/j.ahj.2004.07.013
- Heibornn LK, Chambell LV. Adipose tissue macrophages, low grade inflammation and insulin resistance in human obesity. Curr. Pharm. Des. 2008;14:1225–1230.
- Schenk S, Saberi M, Olefsky JM. Insulin sensitivity: modulation by nutrients and inflammation. J. Clin. Invest. 2008;118:2992–3002.10.1172/JCI34260
- Shoelson SE, Lee J, Goldfine AB. Inflammation and insulin resistance. J. Clin. Invest. 2006;116:1793–1801.10.1172/JCI29069
- Weisberg SP, McCann D, Desai M, Rosenbaum M, Leibel RL, Ferrante AW Jr. Obesity is associated with macrophage accumulation in adipose tissue. J. Clin. Invest. 2003;112:1796–1808.10.1172/JCI200319246
- Xu H, Barnes GT, Yang Q, Tan G, Yang D, Chou CJ, Sole J, Nichols A, Ross JS, Tartaglia LA, Chen H. Chronic inflammation in fat plays a crucial role in the development of obesity-related insulin resistance. J. Clin. Invest. 2003;112:1821–1830.10.1172/JCI200319451
- Hotamisligil GS, Shargill NS, Spiegelman BM. Adipose expression of tumor necrosis factor-α: direct role in obesity-linked insulin resistance. Science. 1993;259:87–91.10.1126/science.7678183
- Xu H, Uysal KT, Becherer JD, Arner P, Hotamisligil GS. Altered tumor necrosis factor-α processing in adipocytes and increased expression of transmembrane TNFα in obesity. Diabetes. 2002;51:1876–1883.10.2337/diabetes.51.6.1876
- Engeli S, Boschmann M, Adams F, Franke G, Gorzelniak K, Janke J, Luft FC, Jordan J. Dissociation between adipose nitric oxide synthase expression and tissue metabolism. J. Clin. Endocrinol. Metab. 2007;92:2706–2711.10.1210/jc.2007-0234
- Yu R, Kim CS, Kwon BS, Kawada T. Mesenteric adipose tissue-derived monocyte chemoattractant protein-1 plays a crucial role in adipose tissue macrophage migration and activation in obese mice. Obesity. 2006;14:1353–1362.10.1038/oby.2006.153
- Suganami T, Nishida J, Ogawa Y. A paracrine loop between adipocytes and macrophages aggravates inflammatory changes: role of free fatty acids and tumor necrosis factor-α. Arterioscler Thromb Vasc Biol. 2005;25:2062–2068.10.1161/01.ATV.0000183883.72263.13
- Pandey DK, Shekelle R, Selwyn BJ, Tangney C, Stamler J. Dietary vitamin C and β-carotene and risk of death in middle-aged men. Am. J. Epidemiol. 1995;142:1269–1278.
- Lazarus SA, Bowen K, Garg ML. Tomato juice and platelet aggregation in type 2 diabetes. J. Am. Med. Assoc. 2004;292:805–806.10.1001/jama.292.7.805
- Giovannucci E. Tomatoes, tomato-based products, lycopene, and cancer: review of the epidemiologic literature. J. Nat. Cancer Inst. 1999;91:317–331.10.1093/jnci/91.4.317
- Blum A, Monir M, Wirsansky I, Ben-Arzi S. The beneficial effects of tomatoes. Eur. J. Int. Med. 2005;16:402–404.10.1016/j.ejim.2005.02.017
- Kim YI, Hirai S, Takahashi H, Goto T, Ohyane C, Tsugane T, Konishi C, Fujii T, Inai S, Iijima Y, Aoki K, Shibata D, Takahashi N, Kawada T. 9-oxo-10(E),12(E)-Octadecadienoic acid derived from tomato is a potent PPARα agonist to decrease triglyceride accumulation in mouse primary hepatocytes. Mol. Nutr. Food Res. 2011;55:585–593.10.1002/mnfr.v55.4
- Hirai S, Kim YI, Goto T, Kang MS, Yoshimura M, Obata A, Yu R, Kawada T. Inhibitory effect of naringenin chalcone on inflammatory changes in the interaction between adipocytes and macrophages. Life Sci. 2007;81:1272–1279.10.1016/j.lfs.2007.09.001
- Granger DL, Taintor RR, Boockvar KS, Hibbs JB. Measurement of nitrate and nitrite in biological samples using nitrate reductase and Griess reaction. Methods Enzymol. 1996;268:142–151.10.1016/S0076-6879(96)68016-1
- Hotamisligil GS. The role of TNFα and TNF receptors in obesity and insulin resistance. J. Int. Med. 1999;245:621–625.10.1046/j.1365-2796.1999.00490.x
- Muoio DM, Newgard CB. Obesity-related derangements in metabolic regulation. Annu. Rev. Biochem. 2006;75:367–401.10.1146/annurev.biochem.75.103004.142512
- Lee JY, Sohn KH, Rhee SH, Hwang D. Saturated fatty acids, but not unsaturated fatty acids, induce the expression of cyclooxygenase-2 mediated through toll-like receptor 4. J. Biol. Chem. 2001;276:16683–16689.10.1074/jbc.M011695200
- Shi H, Kokoeva MV, Inouye K, Tzameli I, Yin H, Flier JS. TLR4 links innate immunity and fatty acid-induced insulin resistance. J. Clin. Invest. 2006;116:3015–3025.10.1172/JCI28898
- Suganami T, Tanimoto-Koyama K, Nishida J, Itoh M, Yuan X, Mizuarai S, Kotani H, Yamaoka S, Miyake K, Aoe S, Kamei Y, Ogawa Y. Role of the Toll-like receptor 4/NF-κB pathway in saturated fatty acid-induced inflammatory changes in the interaction between adipocytes and macrophages. Arteriosclerosis, Thrombosis, Vascular Biol. 2007;27:84–91.10.1161/01.ATV.0000251608.09329.9a