Abstract
Iron is one of the essential trace elements for humans. In this study, the iron contents in fresh, dried, and toasted nori (Pyropia yezoensis) were analyzed. The mean iron content of fresh, dried, and toasted nori were 19.0, 22.6, and 26.2 mg/100 g (dry weight), respectively. These values were superior to other food of plant origin. Furthermore, most of the iron in nori was maintained during processing, such as washing, drying, and toasting. Then, the form of iron in fresh, dried, and toasted nori was analyzed. As a result, an iron storage protein ferritin contributed to iron storage in raw and dried nori, although the precise rate of its contribution is yet to be determined, while ferritin protein cage was degraded in the toasted nori. It is the first report that verified the ferritin contribution to iron storage in such edible macroalgae with commercial importance.
Graphical Abstract
Nori, which is a traditional Japanese food made from a marine alga, was proved to be rich in iron. Further, ferritin contributed to the iron content in nori.
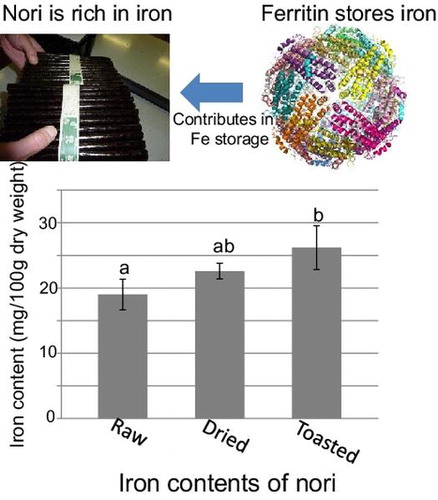
Key words:
Iron is an essential element in almost all living kingdoms. It is recommended that approximately 10–20 mg (9–12.5 mg for a male and 20 mg for a female) iron is taken for an adult from foods everyday (data from Food and Agriculture Organization of the United Nations (FAO); http://www.fao.org/docrep/004/y2809e/y2809e0j.htm), although the values range largely depending on the availabilities of iron in various food matrices. In general, iron deficiency is one of the most serious nutritional problems that affect huge amounts of people worldwide. A well-known iron source with good availability for human is heme iron included in animal foodstuffs. In contrast, plant foodstuffs contain little amount of heme iron. However, they show various iron contents and some can be a candidate for nutritional iron source, which can rescue people suffering from an iron deficiency all over the world. One of the iron-rich plant foodstuffs is legume seed, such as soybean, pea, and common bean.Citation1) Soybean seed contains approximately 10 mg iron per 100 g (dry weight). In legume plants, a major part of iron is stored in ferritin, a ubiquitous multimeric iron storage protein.Citation2–5) Ferritin, which forms spherical hollow protein shell composed of 24 subunits, can deposit thousands of iron atoms as non-toxic and bioavailable form in its inner cavity.Citation6) It is suggested that this type of iron, deposited in ferritin, is an iron source with good bioavailability among plant-derived iron.Citation7–9) Therefore, attempts for the biofortification of staple food crops using the ectopically introduced ferritin gene have been performed in the last decade. Citation10–13)
Recently, it is also demonstrated that algae is a good candidate for a bioavailable iron source.Citation14) Garcia-Casal et al. assayed the iron contents in several types of coastal macroalgae such as Ulva sp., Sargassum sp., Porphyla sp., and Gracilariopsis sp., which are classified as a green alga, a brown alga, and red algae (the last two species), respectively.Citation15,16) As a result, they have high iron contents compared with other food materials derived from plants, and all of them showed good iron absorption rates for human.Citation15,16) Traditionally, Japanese and people in other eastern countries have consumed coastal macroalgae as an indispensable ingredient. Among the edible algae, susabinori or simply called nori (Porphyra yezoensis), red macroalga is one of the most important species, which is extensively cultured on the surface of coastal region in Japan, Korea, and China. The thalli of nori are harvested, cut out, dried, pressed to a sheet, and distributed as dried products (http://www.fao.org/fishery/culturedspecies/Porphyra_spp/en). In Japan, dried nori sheets are selected and divided to some grades depending on its quality, and finally most of them are toasted before our consumption. As shown in this FAO statistics, the global amounts of nori production was 691 million tons in 2012, and this value tends to be increasing in recent years, partly because a trend in a health-conscious diet of western countries. Although iron contents in nori were reported,Citation14,17,18) the reported iron contents in nori are highly varied (2–90 mg iron/100 g of dry matter). Therefore, the iron contents of nori should be re-evaluated, e.g. the loss amounts of iron during the food processing and the iron contents in various grades of nori. Furthermore, a form of stored iron in nori remains unclear.
With respect to the mechanism for iron storage in algae, researches have been performed mainly on prokaryotic and eukaryotic unicellular microalgae. For example, ferritin has a critical role in iron storage and in the proliferation of cyanobacteria.Citation19) Similarly, ferritin plays an important role in iron storage in bloom-forming marine pennate diatoms.Citation20) As a unicellular Chlorophyceae, Chlamydomonas has multiple genes encoding plant-type ferritins, and these gene expression are differently regulated against iron deficiency and sufficiency.Citation21) Ferritin is also detected in a unicellular red alga, Cyanidium caldarium, Citation22)whereas it is suggested that another iron storage mechanism does exist in unicellular brown alga, Ectocarpus siliculosus, which has no orthologue of ferritin in its genome.Citation23) On the other hand, knowledge of iron storage in macroalgae is still very limited. Recently, we showed that a green macroalga, Ulva pertusa, contains high amount of iron, and ferritin protein was detected in thalli of this alga.Citation24) It was the first report that demonstrates ferritin contribution for macroalgal iron storage. In the present study, we focused on iron in various forms and grades of nori, P. yezoensis. At the same time, we provide the first evidence that ferritin functions as an iron storage device in red macroalga, nori.
Materials and methods
Algae
Nori (P. yezoensis) were cultured and harvested on the coastal region in Suma (Kobe, Japan), approximately 100 m offshore from the beach in December 2012 and 2013. After harvest, nori fronds were washed, cut out, pressed to sheets, and dried at 40 °C for 3 h using automatic nori manufacturing machine. Dried nori sheets were further heated and dried at 70 °C for 3 h. The resultant nori sheets were designated as “dried nori.” Dried nori were selected and divided to some classes, such as the first, second, third, fourth grade, and out of the grade. These are the general procedures in the processing of nori. Subsequently, dried nori sheets were toasted at 300 °C for 3 s to generate “toasted nori” sheets. The samples harvested in December 2012 were all judged as the first grade, and those samples were mainly used in this study unless specially mentioned.
Metal content measurement
A few kilograms of the harvested thalli of nori (P. yezoensis) were used as a non-processed sample, designated as “raw.” Raw nori thalli were washed with distilled water and lyophilize for three days, followed by air dried at 120 °C for 5 h. Dried and toasted nori sheets were also air dried at 120 °C similarly. Each sample of 0.5 g (dry weight) was wet-ashed with a solution of 14 ml of HNO3 (60%, Nacalai tesque, Kyoto, Japan), and 0.5 ml of H2O2 (Wako, Tokyo, Japan) for 5 h at 250 °C using a graphite block acid digestion system “Ecopre” (Actac, Tokyo, Japan). The resulting digested solution of each sample was diluted to 40 ml with distilled water (HPLC grade, Nacalai tesque). Then, each sample was diluted 200 folds, followed by the measurement of iron concentration using an atomic absorption spectrophotometer (AAS) AA-6800 (Shimadzu, Kyoto, Japan) equipped with graphite furnace atomizer. The iron concentration of each sample was calculated by standard addition method and/or calibration curve method. To ensure the results of iron concentration analyses, the iron contents of dried nori samples harvested in 2012 winter were analyzed by UV–visual (UV/Vis) spectrophotometric analysis described below. Each 40 ml-sample was mixed with equal volume of 0.5% potassium ferrocyanide followed by the incubation at room temperature for 35 min in the dark place.Citation25) Then, the absorbance at 690 nm was measured using a UV/Vis spectrophotometer (UV-2550, Shimadzu). The concentration of each sample was determined from an average of 3–10 independent samples. ANOVA with Tukey–Kramer multiple comparison test was used to compare iron contents among various forms and grades of nori. Mean differences were considered significant at p < 0.01.
Cloning of P. yezoensis ferritin cDNA(PyFer)and similarity analysis
Total RNA was extracted using the Sepasol reagent (Nacalai) according to the manufacturer’s instructions. Gene specific primer set, (5′-cgtccttaccatgacgatg-3′) and (5′-ccagaaactgacatgggag-3′), was designed according to the sequence deposited in GenBank (Accession No. JX293834). The similarity analysis of the nucleotide sequence was carried out using BLAST2.0 at DDBJ. Theoretical isoelectric point (pI) and molecular weight (MW) of protein was calculated from deduced amino acid sequence of PyFer by pI/MW tool (http://au.expasy.org/tools/). Multiple alignment of the ferritin sequences were performed by ClustalW (http://clustalw.ddbj.nig.ac.jp/top-j.html) program. The phylogenetic tree was created by ClustalW and viewed by tree view program.
Preparation of recombinant PyFer (rPyFer)
To construct the pET vector-based expression plasmid, cDNA fragment of PyFer was amplified by the primer set (5′-gactaccatggcgcgtatgacgttttcg-3′) and (5′-gactaggatccattacgcctgggcatcctc-3′). The resulting PCR fragment was digested by NcoI and BamHI, and inserted to the NcoI/BamHI site of pET21d (Novagen, San Diego, CA). Protein expression was performed at 30 °C after isopropyl-β-D-thiogalactopyranoside induction. The cells were harvested and disrupted by sonication, followed by protein extraction with phosphate buffered saline containing protease inhibitor cocktail (Nacalai tesque). PyFer was expressed as soluble protein, and further purified by ammonium sulfate precipitation (50% saturation), anion exchange chromatography by Q-Sepharose (GE-healthcare, Piscataway, NJ), and size exclusion chromatography by Superdex 200 pg 16/60 column (GE healthcare).
Detection of ferritin in various forms of nori
To enable specific detection of ferritin in nori, a rabbit polyclonal antibody was raised against the recombinant PyFer (rPyFer), and used for the western blot analysis. Prior to western blot analysis, SDS-PAGE was performed with or without a reducing reagent that enables the detection of ferritin monomer and oligomer (24-mer) form, respectively. Generally, oligomeric ferritin can be detected by non-reducing PAGE without heat denaturation, because ferritin oligomer has high stability against the treatment of heat and denaturant.Citation26,27) Protein samples were prepared by extraction from 0.1 g of raw, dried, and toasted nori using 5 ml of PBS. So, each loaded sample contained soluble protein extracted from 200 μg of each form of nori. The horse-radish peroxidase labeled antirabbit IgG (Promega) was used as the secondary antibody. Signal was visualized by using Chemilumi-One (Nacalai) and laser imager (LAS-4000, GE-healthcare).
Iron-containing proteins were detected by non-reducing SDS-PAGE followed by Prussian blue staining of the gel. The gel separated above mentioned protein extracts and was dipped in the mixture of 2% (w/v) potassium ferrocyanide and 2% (w/v) hydrochloride.
Results and discussion
Iron contents of nori and their variation depending on the forms, grades, and years
The iron contents of raw, dried, and toasted nori, which were harvested in December, 2012 and judged as the first grade, are shown in Fig. (A). The mean values of iron contents of raw, dried, and toasted nori were 19.0, 22.6, and 26.2 mg/100 g dry weight, respectively (Fig. (A)). These values were measured by AAS analysis combined with standard addition method. According to the results, the iron contents of edible forms, dried, and toasted nori are quite high among all plant-derived food stuffs such as legumes, cereals, vegetables, and fruits (http://fooddb.mext.go.jp/). In addition, iron content of raw nori was comparable with that of U. pertusa, which had highest iron content among coastal green, red, and brown macroalgae as we analyzed in the previous study.Citation24) Together with the good bioavailability of iron in red alga Porphyra sp. (a native species of Venezuela),Citation15,16) nori can be considered as a good nutritional iron source. Since the dried and toasted nori samples were made from the raw thalli used in this study, these results reflect the true transition of iron concentration during the food processing.
Fig. 1. Iron contents of various forms and grades of nori (P. yezoensis)
(A) Iron contents (mg/100 g dry weight) of raw, dried, and toasted nori. Iron contents of these nori samples harvested in 2012 winter were analyzed by AAS with standard addition method. (B) Evaluation of the iron content values measured by AAS with standard addition (AAS_1), AAS with calibration curve method (AAS_2), and UV/Vis spectrometry. (C) Iron contents of various grades of dried nori were assayed by AAS combined with calibration curve method. Iron concentrations were determined from 3 to 8 independent experiments. Each data point represents the average of replicates and bars indicate standard deviations. The differences in means were compared with ANOVA with Tukey–Kramer multiple comparison test. Means not sharing identical letters are significantly different (p < 0.01).
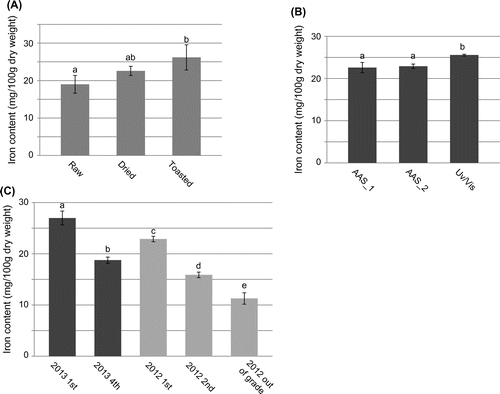
To ensure these values in Fig. (A), iron concentrations were further measured by two different methods, AAS combined with calibration curve method and colorimetric analysis using UV/Vis spectrophotometer. The iron contents of dried nori were calculated to 22.6 ± 1.21, 22.9 ± 0.520, and 25.6 ± 0.205 mg/100 g dry weight by AAS with standard addition method, AAS with calibration curve method, and UV/Vis spectrophotometric method, respectively (Fig. (B)). These values indicated that AAS with calibration curve method, which is simpler than the standard addition method, can be adoptable for the measurements of iron contents of dried nori samples. Colorimetric analysis with UV/Vis spectrophotometric method may slightly overestimate the values. Accordingly, the following iron content measurements were performed by AAS data with standard curve method using dried nori as samples. Dried nori sheets are usually selected and divided to different grades depending on their appearance, such as colors and glaze, which reflect the quality of nori. The iron concentrations in various grades of dried nori are shown in Fig. (C). The samples were harvested in winter of 2012 and 2013. The mean iron contents of the first grade nori harvested in 2012 and 2013 were 22.9 and 27.0 mg/100 g dry weight, respectively (Fig. (C)). The value of 2013 was significantly higher than that of 2012, suggesting that the iron content of nori product varies among the harvested years. Since the variation of the concentration of iron and other minerals among culture locations were pointed out by Yoshie et al.,Citation17) the values may vary among the years of harvest to some extent. The variation in iron content among different grades can be evaluated by the comparison between the first and fourth grade of 2013, and among the first, second, and out of the grade of 2012 (Fig. (C)). The comparisons in iron contents of different grades of nori are significant only when compared among grades harvested in a same year, because the grade is judged relatively every year. In both cases of 2012 and 2013 samples, the iron contents of the samples in the grade of good quality are higher in general. For example, in 2012 samples, the mean iron content of “out of the grade,” which is the worse quality and not to be sold in the market, is 11.3 mg/100 g dry weight, while that of the first and second grade were 22.9 and 15.9 mg/100 g dry weight, respectively (Fig. (C)). Similarly, in 2013 samples, mean iron contents of the first and fourth grade were 27.0 and 18.7 mg/100 g dry weight, respectively (Fig. (C)). These results indicate that there is a clear correlation between iron contents and grades of nori, although previous study suggested no such tendency was observed.Citation17) The reason for the data confliction is not clear. However, the iron content values of preceding studiesCitation17,18) tend to be lower (2–12 mg/100 g dry weight)Citation17) than that of the present results, available database, and another study measuring the iron contents in various algae, including red macroalga (Porphyra sp.).Citation15) To clarify the relationship between the grades of nori and iron contents, further encompassing study is required.
cDNA cloning of ferritin from P. yezoensis (PyFer)
The open reading frame of PyFer cDNA composed of 795 bp, which encoded 264 amino acid residues (Fig. (A)). The calculated pI and MW were 4.52 and 28,135, respectively. The cDNA sequence has been submitted to the NCBI database (GenBank ID: AB918149). The amino acid sequence of PyFer shows 38% and 35% sequence identity with ferritin, respectively, from U. pertusaCitation24,26) (green macroalga) and soybean ferritin subunit No.4.Citation28) Further, PyFer and CmFer, ferritin cDNA of unicellular red alga Cyanidioschyzon merolae,Citation29) share 38.9% identity in their amino acid sequences. The phylogenic tree of various ferritin cDNA indicates that PyFer belongs to the same cluster as the plant-type ferritins, including higher plants (soybean), green algae (Ulva sp., Chlamydomonas reinhardtii, and Volvox carteri), and red algae (P. yezoensis and C. merolae). This tree further supports the close relationship between PyFer and CmFer of a unicellular microred alga C. merolae, (Fig. (B)).
Fig. 2. Sequence description of PyFer.
(A) Multiple alignment of PyFer (GenBank accession number AB918149) with other ferritins: U. pertusa (UpFer, AB691549), Glycine max (GmFer1, M64337), and G. max (GmFer4, AB062756). The amino acid residues forming the ferroxidase site are black hilighted. Putative transit peptide (TP) and extension peptide (EP), and helix A–E of PyFer are indicated by bars on the sequences. Strictly conserved amino acid residues among 6 members are indicated by asterisks, while similar residues are by colons. (B) Unrooted phylogenetic tree of various ferritins generated using the neighbor-joining (NJ) method. Bar indicates p-distances. Ferritins are PyFer, UpFer, GmFer1, GmFer4 (Genbank IDs of them are shown above), C. merolae (CmFer, XP_005537881), Ulva fasciata (UfFer, EF437243), C. reinhardtii (CrFer1, AF503338), V. carteri f. Nagariensis (VaFer, XP_002951031), Pseudo-nitzschia australis (PaFer, ACI30661), Pseudo-nitzschia multiseries (PmFer, ACI30660), Synechocystis sp. PCC6803 (CyanobacFer, AGF53187), Escherichia coli FTN (EcFTN, X53513), E. coli Bfr (EcBfr, ABJ02814), Homo sapiens (HuHF, M11146), and H. sapiens (HuLF, M11147).
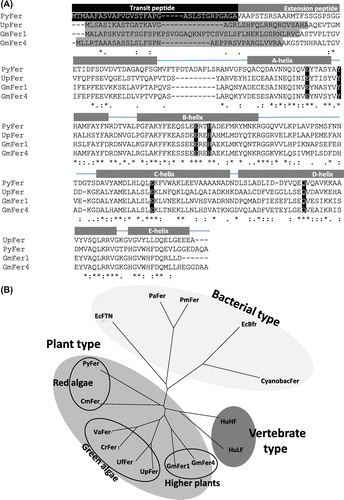
The putative secondary structure deduced from the three-dimensional structure of algalCitation26) and higher-plant ferritinCitation28) is shown in Fig. (A). According to the sequence alignment, the central part of PyFer forms 4-helix bundle, which is the conserved motif among all the identified ferritin from bacteria to mammals.Citation30) The amino acid residues forming the iron oxidation site (Ferroxidase site)Citation31,32) are completely conserved in PyFer sequence (Fig. (A)). Similar to the other plant-derived ferritin, PyFer possesses a putative transit peptide (TP), which is responsible for the targeting to a plastid, although this putative TP has no similarity to other plant ferritin sequences. However, the TP sequences are very highly divergent.Citation33,34) Together with the fact that almost all the identified plant-type ferritins are targeted to a plastid, PyFer also can be considered as a chloroplast protein. The additional N-terminal region, which positions downstream of the TP, is generally designated as the extension peptide (EP) in plant type ferritin.Citation3) This region is the N-terminus of mature plant ferritin and forms an α-helix unique to plant ferritins.Citation26,28) The EP region also presents in PyFer sequence in the downstream of putative TP region. Since the core region forming the 4-helix bundle is highly conserved among PyFer, UpFer, and other plant type ferritins, PyFer is also supposed to forms 4-helix bundle subunit, which assembles to spherical 24-mer.
Detection of PyFer in Thallus and various forms of nori
To clarify whether ferritin (PrFer) contributes to the iron storage in nori, we performed western blotting using specific antibody raised against recombinant PyFer. First, we detected the monomeric PyFer after reduced SDS-PAGE. Fig. (B) shows that monomeric PyFer (approximately 27 kDa) are present in raw, dried, and toasted nori. As described above, ferritin usually functions as an iron storage protein by forming a multimeric protein shell composed of 24 subunits. To detect functional 24-mer of PyFer in each form of nori, we performed another western blotting after SDS-PAGE without heat treatment. Fig. (A) shows that PyFer were detected as the bands whose apparent molecular masses are much larger than 250 kDa marker (Fig. (A)). Subsequently, to detect the iron-containing protein, the gel of non-reducing and non-heating SDS-PAGE was treated by Prussian blue staining (Fig. (B)). In Fig. (B), iron-containing soybean ferritin purified from dry soybean seeds was loaded as positive control of iron-containing plant ferritin (Fig. (B), lane C). Fig. (A) and (B) show that bands of multimeric and iron-containing PyFer are detected in raw and dried nori, whereas not in toasted one. Thus, these results suggest that PyFer functions as multimeric (24-mer) iron storage protein in raw and dried nori as is the case in higher plants. In contrast, there was no PyFer 24-mer in toasted nori, although monomeric one was present in comparable amounts with raw and dried nori (Fig. (B)). Thus, PyFer functioned as a multimeric iron storage protein, which was tolerant of the drying process at 70 °C. However, the precise rate of ferritin contribution in iron content of nori is still unknown. Further characterizations of PyFer in various forms of nori are required. As shown in Fig. (A), few soluble proteins were detected in toasted nori, indicating that almost soluble proteins were denaturated or aggregated during toasting process at 300 °C. In contrast, PyFer was still detectable in toasted nori, though it was not detected as a native 24-mer form. Monomeric or dimeric ferritin may still contribute to iron binding in toasted nori, because the various forms of ferritin oligomer were seen in pea seed,Citation35) and the iron containing dimeric ferritin was detected in soymilk (Masuda T. Unpublished data).
Fig. 3. Reducing and heat-denaturing SDS-PAGE (A) and Western-blot (B) analysis of protein extracted from raw, dried, and toasted nori. Lane 1, raw; lane 2, dried; and lane 3, toasted nori.
(A) Protein extract from 100 μg of raw, dried, and toasted nori were loaded to 12.5% polyacrylamide gel and stained with Coomassie Brilliant Blue R-250. (B) The 50 times diluted above extracts were separated by 12.5% gel, followed by electroblotted on the PVDF membrane. Antirecombinant PyFer antiserum was used as primary antibody. Approximately 1 ng of recombinant PyFer was loaded as a control (lane C).
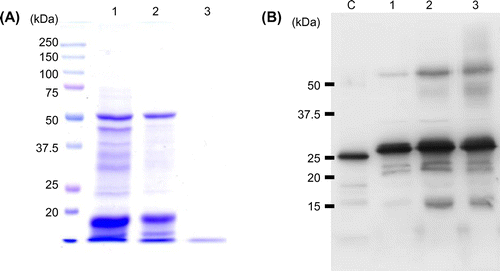
Fig. 4. Non-reducing and non-heat-denaturing SDS-PAGE analysis of oligomeric state of PyFer in the raw, dried, and toasted nori extracts.
Protein extract from 100 μg of raw, dried, and toasted nori were loaded to 7.5% polyacrylamide gel without reducing reagents and heat treatment. Lane 1, raw; lane 2, dried; and lane 3, toasted nori. (A) Western blot analysis of non-reducing nori extracts. Antirecombinant PyFer antiserum was used as primary antibody. (B) The gel was stained by Prussian blue stain. The iron-containing protein on the gel was stained as light blue bands. Approximately, 50 ng of native soybean ferritin purified from dry soybean seeds was loaded as a control (lane C).
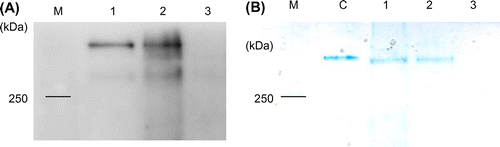
It has been demonstrated that ferritin plays a crucial role in iron storage in higher plantsCitation35–37) and unicellular microalgae.Citation19,38–40) On the other hand, ferritin contribution to iron storage in macroalgae or seaweeds is explored only recently,Citation24) although a ferritin gene is described as a stress-induced gene in a green seaweed Ulva sp.Citation41) Algae have developed sophisticated mechanisms for iron acquisition and storage, because one-third of the ocean is assumed to be deficient in iron due to its extremely low solubility in the oxidized state. Recently, genome project of nori, P. yezoensis, has just been completed.Citation42–44) Hence, nori can be one of the candidates for a model in exploring a mechanism for iron acquisition and storage in macroalgae in addition to its economical and nutritional significance.
Acknowledgments
This work was supported by a research grant from the foundation for Laver Cultivation Promotion and a Grant-in-Aid for Challenging Exploratory Research [grant number 24658287] from JSPS. We thank Mr Akira Morimoto and Mr Yasumasa Miura (Kobe City Fishery Association) for their kind help in harvesting and preparing nori samples.
References
- Welch RM, House WA, Beebe S, Cheng Z. Genetic selection for enhanced bioavailable levels of iron in bean (Phaseolus vulgaris L.) seeds. J. Agric. Food. Chem. 2000;48:3576–3580.10.1021/jf0000981
- Hoppler M, Zeder C, Walczyk T. Quantification of ferritin-bound iron in plant samples by isotope tagging and species-specific isotope dilution mass spectrometry. Anal. Chem. 2009;81:7368–7372.10.1021/ac900885j
- Briat J-F, Duc C, Ravet K, Gaymard F. Ferritins and iron storage in plants. Biochim. Biophys. Acta. 2010;1800:806–814.10.1016/j.bbagen.2009.12.003
- Lukac RJ, Aluru MR, Reddy MB. Quantification of ferritin from staple food crops. J. Agric. Food Chem. 2009;57:2155–2161.10.1021/jf803381d
- Laulhere JP, Lescure AM, Briat JF. Purification and characterization of ferritins from maize, pea, and soya bean seeds. Distribution in various pea organs. J. Biol. Chem. 1988;263:10289–10294.
- Arosio P, Ingrassia R, Cavadini P. Ferritins: A family of molecules for iron storage, antioxidation and more. Biochim. Biophys. Acta. 2009;1790:589–599.10.1016/j.bbagen.2008.09.004
- Zhao G. Phytoferritin and its implications for human health and nutrition. Biochim. Biophys. Acta. 2010;1800:815–823.10.1016/j.bbagen.2010.01.009
- Beard JL, Burton JW, Theil EC. Purified ferritin and soybean meal can be sources of iron for treating iron deficiency in rats. J. Nutr. 1996;126:154–160.
- Lonnerdal B. The importance and bioavailability of phytoferritin-bound iron in cereals and legume foods. Int. J. Vitam. Nutr. Res. 2007;77:152–157.10.1024/0300-9831.77.3.152
- Goto F, Yoshihara T, Shigemoto N, Toki S, Takaiwa F. Iron fortification of rice seed by the soybean ferritin gene. Nat. Biotechnol. 1999;17:282–286.
- Drakakaki G, Christou P, Stoger E. Constitutive expression of soybean ferritin cDNA in transgenic wheat and rice results in increased iron levels in vegetative tissues but not in seeds. Transgenic Res. 2000;9:445–452.10.1023/A:1026534009483
- Drakakaki G, Marcel S, Glahn RP, Lund EK, Pariagh S, Fischer R, Christou P, Stoger E. Endosperm-specific co-expression of recombinant soybean ferritin and Aspergillus Phytase in maize results in significant increases in the levels of bioavailable iron. Plant Mol. Biol. 2005;59:869–880.10.1007/s11103-005-1537-3
- Masuda H, Kobayashi T, Ishimaru Y, Takahashi M, Aung MS, Nakanishi H, Mori S, Nishizawa NK. Iron-biofortification in rice by the introduction of three barley genes participated in mugineic acid biosynthesis with soybean ferritin gene. Front. Plant Sci. 2013;4:132:1–12.
- Shaw NS, Liu YH. Bioavailability of iron from purple laver (Porphyra spp.) estimated in a rat hemoglobin regeneration bioassay. J. Agric. Food. Chem. 2000;48:1734–1737.10.1021/jf990759y
- Garcia-Casal MN, Pereira AC, Leets I, Ramirez J, Quiroga MF. High iron content and bioavailability in humans from four species of marine algae. J. Nutr. 2007;137:2691–2695.
- Garcia-Casal MN, Ramirez J, Leets I, Pereira AC, Quiroga MF. Antioxidant capacity, polyphenol content and iron bioavailability from algae (Ulva sp., Sargassum sp. and Porphyra sp.) in human subjects. Br. J. Nutr. 2009;101:79–85.10.1017/S0007114508994757
- Yoshie Y, Suzuki T, Shirai T, Hirano T. Dietary fiber and minerals in dried nori of various culture locations and prices. Nippon Suisan Gakkaishi. 1993;59:1763–1767.10.2331/suisan.59.1763
- Yoshie Y, Suzuki T, Shirai T, Hirano T. Changes in the contents of dietary fibers, minerals, free amino acids, and fatty acids during processing of dried nori. Nippon Suisan Gakkaishi. 1994;60:117–123.10.2331/suisan.60.117
- Keren N, Aurora R, Pakrasi HB. Critical roles of bacterioferritins in iron storage and proliferation of cyanobacteria. Plant Physiol. 2004;135:1666–1673.10.1104/pp.104.042770
- Marchetti A, Parker MS, Moccia LP, Lin EO, Arrieta AL, Ribalet F, Murphy MEP, Maldonado MT, Armbrust EV. Ferritin is used for iron storage in bloom-forming marine pennate diatoms. Nature. 2009;457:467–470.10.1038/nature07539
- Long JC, Sommer F, Allen MD, Lu S-F, Merchant SS. FER1 and FER2 encoding two ferritin complexes in Chlamydomonas reinhardtii chloroplasts are regulated by iron. Genetics. 2008;179:137–147.10.1534/genetics.107.083824
- Nagasaka S, Nishizawa NK, Negishi T, Satake K, Mori S, Yoshimura E. Novel iron-storage particles may play a role in aluminum tolerance of Cyanidimin caldarium. Planta. 2002;215:399–404.10.1007/s00425-002-0764-y
- Boettger LH, Miller EP, Andresen C, Matzanke BF, Kuepper FC, Carrano CJ. Atypical iron storage in marine brown algae: a multidisciplinary study of iron transport and storage in Ectocarpus siliculosus. J. Exp. Bot. 2012;63:5763–5772.10.1093/jxb/ers225
- Morimoto S, Masuda T, Sugihara I, Toyohara H. Identification and characterization of a ferritin gene and its product from the Multicellular green alga Ulva pertusa. Biosci. Biotechnol. Biochem. 2012;76:1913–1919.10.1271/bbb.120400
- Rad AM, Janic B, Iskander AS, Soltanian-Zadeh, Arbab AS. Measurement of quantity of iron in magnetically labeled cells: comparison among different UV/VIS spectrometric methods. BioTechniques. 2007;43:627–628, 630, 632 passim.10.2144/000112599
- Masuda T, Morimoto SI, Mikami B, Toyohara H. The extension peptide of plant ferritin from sea lettuce contributes to shell stability and surface hydrophobicity. Protein Sci. 2012;21:786–796.
- Stefanini S, Cavallo S, Wang CQ, Tataseo P, Vecchini P, Giartosio A, Chiancone E. Thermal stability of horse spleen apoferritin and human recombinant H apoferritin. Arch. Biochem. Biophys. 1996;325:58–64.10.1006/abbi.1996.0007
- Masuda T, Goto F, Yoshihara T, Mikami B. Crystal structure of plant ferritin reveals a novel metal binding site that functions as a transit site for metal transfer in ferritin. J. Biol. Chem. 2010;285:4049–4059.10.1074/jbc.M109.059790
- Matsuzaki M, Misumi O, Shin IT, Maruyama S, Takahara M, Miyagishima SY, Mori T, Nishida K, Yagisawa F, Nishida K, Yoshida Y, Nishimura Y, Nakao S, Kobayashi T, Momoyama Y, Higashiyama T, Minoda A, Sano M, Nomoto H, Oishi K, Hayashi H, Ohta F, Nishizaka S, Haga S, Miura S, Morishita T, Kabeya Y, Terasawa K, Suzuki Y, Ishii Y, Asakawa S, Takano H, Ohta N, Kuroiwa H, Tanaka K, Shimizu N, Sugano S, Sato N, Nozaki H, Ogasawara N, Kohara Y, Kuroiwa T. Genome sequence of the ultrasmall unicellular red alga Cyanidioschyzon merolae 10D. Nature. 2004;428:653–657.10.1038/nature02398
- Crichton RR, Declercq JP. X-ray structures of ferritins and related proteins. Biochim. Biophys. Acta. 2010;1800:706–718.10.1016/j.bbagen.2010.03.019
- Lawson DM, Treffry A, Artymiuk PJ, Harrison PM, Yewdall SJ, Luzzago A, Cesareni G, Levi S, Arosio P. Identification of the ferroxidase centre in ferritin. FEBS Lett. 1989;254:207–210.10.1016/0014-5793(89)81040-3
- Lawson DM, Artymiuk PJ, Yewdall SJ, Smith JM, Livingstone JC, Treffry A, Luzzago A, Levi S, Arosio P, Cesareni G, Thomas CD, Shaw WV, Harrison PM. Solving the structure of human H ferritin by genetically engineering intermolecular crystal contacts. Nature. 1991;349:541–544.10.1038/349541a0
- Bruce BD. Chloroplast transit peptides: structure, function and evolution. Trends Cell Biol. 2000;10:440–447.10.1016/S0962-8924(00)01833-X
- Bruce BD. The paradox of plastid transit peptides: conservation of function despite divergence in primary structure. Biochim. Biophys. Acta. 2001;1541:2–21.10.1016/S0167-4889(01)00149-5
- Laulhere JP, Laboure AM, Briat JF. Mechanism of the transition from plant ferritin to phytosiderin. J. Biol. Chem. 1989;264:3629–3635.
- Lescure AM, Proudhon D, Pesey H, Ragland M, Theil EC, Briat JF. Ferritin gene transcription is regulated by iron in soybean cell cultures. Proc. Natl. Acad. Sci. U S A. 1991;88:8222–8226.10.1073/pnas.88.18.8222
- Briat JF, Ravet K, Arnaud N, Duc C, Boucherez J, Touraine B, Cellier F, Gaymard F. New insights into ferritin synthesis and function highlight a link between iron homeostasis and oxidative stress in plants. Ann. Bot. 2010;105:811–822.10.1093/aob/mcp128
- Laulhère JP, Labouré AM, Van Wuytswinkel O, Gagnon J, Briat JF. Purification, characterization and function of bacterioferritin from the cyanobacterium Synechocystis P.C.C. 6803. Biochem. J. 1992;281:785–793.
- Kranzler C, Lis H, Shaked Y, Keren N. The role of reduction in iron uptake processes in a unicellular, planktonic cyanobacterium. Environ. Microbiol. 2011;13:2990–2999.10.1111/j.1462-2920.2011.02572.x
- Shcolnick S, Summerfield TC, Reytman L, Sherman LA, Keren N. The mechanism of iron homeostasis in the unicellular cyanobacterium Synechocystis sp. PCC 6803 and its relationship to oxidative stress. Plant Physiol. 2009;150:2045–2056.10.1104/pp.109.141853
- Wu TM, Hsu YT, Sung MS, Hsu YT, Lee TM. Expression of genes involved in redox homeostasis and antioxidant defense in a marine macroalga Ulva fasciata by excess copper. Aquat. Toxicol. 2009;94:275–285.10.1016/j.aquatox.2009.07.010
- Nakamura Y, Sasaki N, Kobayashi M, Ojima N, Yasuike M, Shigenobu Y, Satomi M, Fukuma Y, Shiwaku K, Tsujimoto A, Kobayashi T, Nakayama I, Ito F, Nakajima K, Sano M, Wada T, Kuhara S, Inouye K, Gojobori T, Ikeo K. The first symbiont-free genome sequence of marine red alga, Susabi-nori (Pyropia yezoensis). Plos One. 2013;8; e57122:1–11.
- Kong F, Sun P, Cao M, Wang L, Mao Y. Complete mitochondrial genome of Pyropia yezoensis: reasserting the revision of genus Porphyra. Mitochondrial DNA. 2013.
- Wang L, Mao Y, Kong F, Li G, Ma F, Zhang B, Sun P, Bi G, Zhang F, Xue H, Cao M. Complete sequence and analysis of plastid genomes of two economically important red algae: Pyropia haitanensis and Pyropia yezoensis. PLoS ONE. 2013;8:e65902.10.1371/journal.pone.0065902