Abstract
Membrane proteins of amino acid–polyamine–organocation (APC) superfamily transport amino acids and amines across membranes and play important roles in the regulation of cellular processes. The alanine or glycine: cation symporter (AGCS) family belongs to APC superfamily and is found in prokaryotes, but its substrate specificity remains to be clarified. In this study, we found that a halotolerant cyanobacterium, Aphanothece halophytica has two putative ApagcS genes. The deduced amino acid sequence of one of genes, ApagcS1, exhibited high homology to Pseudomonas AgcS. The ApagcS1 gene was expressed in Escherichia coli JW4166 which is deficient in glycine uptake. Kinetics studies in JW4166 revealed that ApAgcS1 is a sodium-dependent glycine transporter. Competition experiments showed the significant inhibition by glutamine, asparagine, and glycine. The level of mRNA for ApagcS1 was induced by NaCl and nitrogen-deficient stresses. Uptake of glutamine by ApAgcS1 was also observed. Based on these data, the physiological role of ApAgcS1 was discussed.
Graphical Abstract
Halotolerant cyanobacterium Aphanothece halophytica has a sodium-dependent glycine transporter that was induced by NaCl and nitrogen deficient stresses.
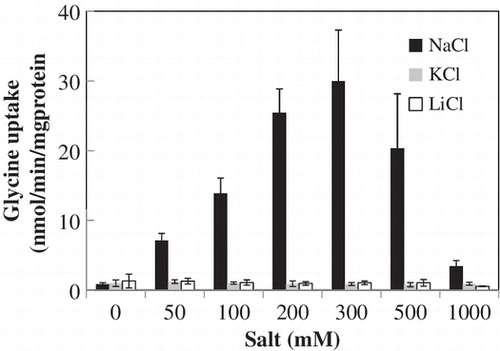
The amino acid–polyamine–organocation (APC) superfamily (TC# 2.A.3) is one of the largest families of secondary active transporters for amino acids.Citation1) These transporters are found in bacteria, archaea, yeast, fungi, unicellular eukaryotes, plants, and animals and hence are ubiquitous in nature.Citation2) The alanine or glycine:cation symporter (AGCS) family (TC# 2.A.25) belongs to APC superfamily and is one of the secondary active amino acid transporters found exclusively in prokaryotes.
Functional characterization of AGCS family remains largely unknown. Only three members of the family have been functionally characterized. These are the cycA gene in Escherichia coli,Citation3,4) the dagA gene in Alteromonas haloplanktis,Citation5) and the acp gene in thermophilic bacterium PS3.Citation6,7) Members of the AGCS family have been reported to transport alanine and/or glycine in symport with Na+ and/or H+. These proteins show limited sequence similarity in the APC family.
Cyanobacteria are oxygenic photosynthetic bacteria and they synthesize all needed organic molecules using simple inorganic compounds.Citation8) Not only the uptake of inorganic nitrogen such as nitrate and ammonium but also the uptake of amino acids plays important roles in nitrogen assimilation.Citation9,10) Amino acid uptake of cyanobacteria seems to be carried out largely by ABC-type transporters with broad substrate specificity.Citation9,10) However, some transporters with specific substrates such as glycine betaine (BetT) and glutamate (GltS) have been reported.Citation11,12)
Aphanothece halophytica (A. halophytica) is a halotolerant cyanobacterium isolated from the Dead Sea which can grow at a wide range of NaCl concentrations (0.2–3.0 M) and pH values (pH 7–11).Citation11,12) A. halophytica accumulates a large amount of glycine betaine (betaine) and mycosporine molecules (mycosporine-2-glycine) under high-salinity conditions.Citation13,14) In A. halophytica, betaine is synthesized by a three-step methylation of glycine.Citation13) Therefore, synthesis and uptake of glycine are important for A. halophytica cells. In this study, we searched the glycine-uptake gene and found that A. halophytica has AGCS family. Kinetics studies revealed that ApAgcS1 is a novel sodium-dependent glycine transporter. RT-PCR and the competition experiments suggested that ApAgcS1 plays an important role in the uptake of glycine and glutamine under stress conditions.
Materials and methods
Strains and growth conditions
A. halophytica was grown photoautotrophically in BG-11 liquid mediumCitation15) plus 18 mM NaNO3 and Turk Island salt solution containing 0.5 M NaCl at 30 °C with shaking (160 rpm) under continuous fluorescent white light (40 μE m−2 s−1). Synechococcus elongatus PCC 7942 was grown photoautotrophically in BG-11 liquid medium plus 18 mM NaNO3 at 30 °C with shaking (160 rpm) under continuous fluorescent white light (40 μE m−2 s−1). For the deletion mutants of S. elongatus PCC 7942, antibiotics, chloramphenicol (25 μg/mL) or spectinomycin (25 μg/mL), were used.
Escherichia coli (E. coli) DH5α cells were grown at 37 °C in Luria–Bertani (LB) medium which contains 10 mg tryptone, 5 mg yeast extract, and 5 mg NaCl per mL. E. coli strain JW4166 (ΔcycA757::kan) which is deficient for the uptake of glycine was obtained from the Japanese National Institute of Genetics (Mishima, Shizuoka, Japan) and was grown at 37 °C in LB medium containing kanamycin (25 μg/mL).
Construction of expression plasmid in the E. coli cell
The Apagcs1 gene was amplified by PCR using the A. halophytica genomic DNA as template and the primer set BamHI_F_pTrc (5′-GGATCCGGGAAAGTTAAACTCGTGAGCA-3′) and SalI_R_pTrC (5′-GTCGACTCGAAACATACCCGCCTCAA-3′). The PCR reactions were performed using AmpliTaq Gold (Applied Biosystems, Foster City, CA) according to the manufacturer’s protocol. The PCR conditions employed were as follows: denaturation at 95 °C for 5 min; 30 amplification cycles with denaturation for 30 s at 95 °C, annealing for 30 s at 55 °C, and extension for 2 min at 72 °C. The amplified 1948 bp DNA fragment was ligated to the pCR®2.1 (Invitrogen, Carlsbad, CA) vector and sequenced. The inserts were transferred into the BamHI/SalI sites of pTrcHis2C (Invitrogen, Carlsbad, CA). The resulting plasmid, pApagcS1 harboring ApagcS1, was fused in frame to six histidine residues at the C-terminus and was transferred first to E. coli DH5α and then to E. coli JW4166.
Construction of expression plasmid in the cyanobacterium cell
The ApagcS1 was amplified by PCR using pApagcS1 harboring ApagcS1 as template and the primer set BamHI_F_pTrc (5′-GGATCCGGGAAAGTTAAACTCGTGAGCA-3′) and Bam-R (5′-ACGGATCCTCAATGATGATGATGATGATG-3′) containing 6x His-tag sequence. The PCR reactions were the same as those described above except that KOD-Plus-Neo (Toyobo, Japan) was used in place of AmpliTaq Gold. The amplified 1977 bp DNA fragment was ligated to the pMD20 (Simple) T-Vector. Then, the inserts were transferred into the BamHI sites of the pSyn_1 Vector (Invitrogen, Carlsbad, CA). The resulting plasmid, pSyn_1 ApagcS1 harboring ApagcS1, was transferred first to E. coli DH5α and then to S. elongatus PCC 7942. The inactivation of the natG gene (Synpcc7942_1894) in S. elongatus PCC 7942 was carried out by the integration of chloramphenicol resistance gene (0.8 kb) into the middle of natG coding region.Citation13)
Transport assays
The E. coli JW4166 cells transformed with pTrcHis2C or pApagcS1 were grown overnight at 37 °C in LB medium containing kanamycin (25 μg/mL) and ampicillin (100 μg/mL) and were inoculated into the same fresh medium until the OD620 reached 0.4. Then, isopropyl β-D-1thiogalactopyranoside (IPTG) (1 mM, final concentration) was added. After 1 h of incubation, cells were harvested, washed twice, and suspended in the solution containing 100 mM Tris-HCl (pH 7.4), 4 mM MgSO4, and 300 mM NaCl to become 2.0 OD620. After addition of 2 mM Tris-lactate (pH 7.4, final concentration), the cell suspension was incubated for 10 min at 37 °C, and the uptake was initiated by the addition of appropriate concentrations of [U-14C] glycine. Cells were collected on 0.2 μm cellulose nitrate filters (Advantec MFS, Chiba, Japan). Filters were washed with 3 mL of buffer, and the radioactivity trapped in the filters was measured with a liquid scintillation counter (model 3200C; Aloka Instruments Co., Tokyo, Japan). Competitions for glycine uptake were performed in the presence of 100-fold molar excess competitors. Radiolabeled [U-14C] glycine and glutamine were purchased from American Radiolabeled Chemicals, Inc. (St. Louis, USA).
Reverse transcriptase-PCR (RT-PCR) analysis
Culture of A. halophytica (10 mL, 0.5 OD750) was harvested. The cell pellet was used for RNA extraction by the TRIzol method. The cDNA was synthesized from 1 μg of total RNA by using the cDNA synthesis kit (Takara, Otsu, Japan). Portions of 2-μl synthesized cDNA were subjected to PCR in a reaction volume of 20 μL. Specific primer sets, A_sym_mid_F (5′-GAACCTGACGGCACTTATCGAG-3′), and ApagcS1RT_R (5′-ATGCCACCGGTAATCGCTGAATC-3′) were used for PCR at a final concentration of 0.2 μM in the PCR mixture. The PCR reactions were performed using AmpliTaq Gold (Applied Biosystems, Foster City, CA) according to the manufacturer’s protocol. The PCR conditions employed were as follows: denaturation at 95 °C for 5 min; 28 amplification cycles with denaturation for 30 s at 95 °C, annealing for 30 s at 55 °C, and extension for 30 s at 72 °C. The resultant reaction mixtures were subjected to 1.5% agarose electrophoresis and were visualized by staining with ethidium bromide. The intensities of band were quantified by GelQuant Express software.
Extracellular amino acids
For the determination of extracellular amino acid in the growth medium of S. elongatus PCC 7942, cells grown in BG-11 medium (supplemented with antibiotics in the case of the mutants) were washed, resuspended in BG-11 medium containing 10 mM NH4Cl in the place of 18 mM NO3−, and incubated for 24 h. Samples of the cell suspensions were filtered. The filtrates were analyzed by high-performance liquid chromatography (Prominence, Shimadzu, Kyoto Japan).
Other methods
The nucleotide sequences were determined using an ABI310 genetic analyzer (Applied Biosystems, Foster City, CA). The ion contents were determined with a Shimadzu PIA-1000 personal ion analyzer (Shimadzu, Kyoto, Japan). After the E. coli cells were harvested, lysed by sonication, and centrifuged at 2290× g for 10 min, the supernatant was centrifuged twice at 22,000× g for 30 min at 4 °C. The resulting supernatant and pellet were used as the soluble and membrane fractions, respectively. Protein content was determined by the Lowry’s method, as described previously.Citation13) SDS-PAGE and Western blot analysis were carried out as described previously.Citation16) An antibody raised against the six His-tag was purchased from R&D Systems (Minneapolis, MN). The hydropathy profile of the proteins was predicted by the method of Kyte and Doolittle.Citation17) The transmembrane (TM) structure of ApAgcS1 was predicted using the computer program. TMHMM (http://www.cbs.dtu.dk/services/TMHMM/). The nucleotide sequence data for ApagcS1 and ApagcS2 have been deposited in the DDBJ database under the accession no. AB971927 and AB971928, respectively.
Results and discussion
Cloning of the putative sodium alanine/glycine cation symporter (agcS) gene from A. halophytica
Since a sodium-dependent agcS gene was isolated from a marine bacterium A. haloplanktis (dagA gene in A. haloplanktis),Citation5) we searched the homologous genes in A. halophytica. Based on shotgun sequencing of A. halophytica, we found two candidate genes in the A. halophytica genome. One gene encoded 480 amino acid residues and was designated as ApagcS1 (accession No. AB971927). The other gene encoded 517 amino acid residues and was designated as ApagcS2 (accession No. AB971928). The putative protein ApAgcS1 exhibited 33% identity with Acp in thermophilic bacterium PS3Citation6) and 32% with DagA in A. haloplanktis.Citation5) The encoded protein ApAgcS2 exhibited 32% identity with DagA in A. haloplanktis and 23% identity with Acp in PS3. Interestingly, ApAgcS1 exhibited high homology (58% identity) with AgcS from Pseudomonas pseudoalcaligenes (accession No. WP_003457884). ApAgcS2 exhibited high homology with the proteins from several marine cyanobacteria. The phylogenetic tree among the above-mentioned proteins indicated that ApAgcS1 exhibits the closest homology to P. pseudoalcaligenes AgcS, whereas ApAgcS2 exhibits the closest homology to DagA from A. haloplanktis (Fig. (A)). Hydropathy profiling of ApAgcS1 (Fig. (B)) suggested that the transporter has 10 TM segments. Since we could not detect the activity of ApAgcS2 (data not shown), thereafter, we focused on ApAgcS1.
Fig. 1. Phylogenetic tree and TM topological model of AGCS family proteins.
Notes: (A) Phylogenetic tree of ApAgcS1, ApAgcS2, Acp from thermophilic bacterium PS3, Pseudomonas AgcS, and DagA from A. haloplanktis. The tree was constructed from alignments of full-length amino acid sequences using the Clustal W and TreeView programs. (B) TM topology model of ApAgcS1. TM topology model of ApAgcS1 was calculated by an algorithm TMHMM.
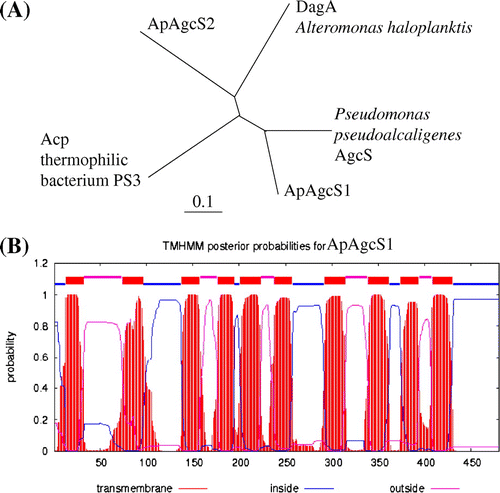
Transport properties of ApAgcS1 expression in E. coli mutant cell
Next, we examined the kinetic properties of ApAgcS1. We expressed ApAgcS1 in E coli JW4166 cells. The expression of ApagcS1 was confirmed by RT-PCR, but we could not detect the protein band of ApAgcS1 by SDS-PAGE or by immunoblotting using anti-His-tag antibodies under various conditions (data not shown). No measurable uptake of [U-14C] glycine was observed for the JW4166 cells transformed with the only vector, pTrcHis2C (Fig. (A)). The JW4166 cells transformed with pApagcS1 took up glycine immediately and saturated within 10 min (Fig. (A)). Exogenous addition of NaCl to the assay medium significantly increased the uptake of glycine, with maximum uptake at 0.3 M NaCl (Fig. (B)). However, the addition of KCl and LiCl was ineffective. These data indicate that ApAgcS1 is a Na+-dependent glycine transporter.
Fig. 2. Uptake of glycine by ApAgcS1.
Notes: (A) Time course of glycine uptake by ApAgcS1. B) Effects of NaCl, KCl, and LiCl on glycine uptake by ApAgcS1. ApAgcS1 expressing E. coli JW4166 cells were grown at 37 °C as described in “Materials and Methods.” Glycine uptake was measured after 10 μM [U-14C] glycine was added. In (B), duration of uptake was 1 min. Each value represents the average of three independent measurements and error bars represent standard deviations.
![Fig. 2. Uptake of glycine by ApAgcS1.Notes: (A) Time course of glycine uptake by ApAgcS1. B) Effects of NaCl, KCl, and LiCl on glycine uptake by ApAgcS1. ApAgcS1 expressing E. coli JW4166 cells were grown at 37 °C as described in “Materials and Methods.” Glycine uptake was measured after 10 μM [U-14C] glycine was added. In (B), duration of uptake was 1 min. Each value represents the average of three independent measurements and error bars represent standard deviations.](/cms/asset/86b49372-2247-407c-aea2-f6c3991d03ac/tbbb_a_968091_f0002_b.gif)
Kinetic properties of ApAgcS1 in JW4166 cells
Glycine uptake rates increased with an increase in glycine and showed saturation curves (Fig. (A)). Apparent Km and Vmax values were determined by a nonlinear regression software GraphPad Prism version 6.00 as 12.5 μM and 35.7 nmol/min/mg protein, respectively. The apparent Km value for glycine in ApAgcS1 was similar to a novel neutral amino acid transporter (SnatA) from the hyperthermophilic archaeon Thermococcus sp. KS-1 (24 μM),Citation18) but it is up to one order of magnitude higher than those of CycA from E. coli (2.4 μM) and Acp from thermophilic bacterium PS3 (4.0 μM).Citation18) The apparent Km value for glycine in DagA from A. haloplanktis has not been reported, but the apparent Km value for alanine in DagA from A. haloplanktis has been reported as 9 μM.Citation19)
Fig. 3. Kinetics of glycine uptake by ApAgcS1.
Notes: (A) Saturation curve. (B) Effects of pH. Glycine uptake by ApAgcS1 in JW4166 cells was assayed in the presence of 0.3 M NaCl. The pH for saturation curve was 7.4. Duration of uptake was 1 min. Each value represents the average of three independent measurements and error bars represent standard deviations.
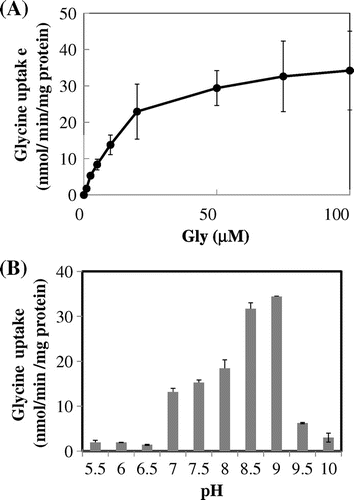
The effect of pH on glycine uptake by ApAgacS1 in JW4166 cells was a typical bell-shaped curve with the optimum pH around 8.5–9.0, suggesting that there was no proton-coupled glycine transport. Both data of Figs. and suggest that ApAgcS1 is a sodium-coupled glycine transporter. Sodium-coupled glycine transporter is also reported for the DagA from A. haloplanktisCitation5), whereas SnatA from the hyperthermophilic archaeon Thermococcus sp. KS-1 has been a proton-coupled glycine transporter.Citation18)
Substrate specificity of ApAgcS1
The substrate specificity of ApAgcS1 was determined by means of competition studies (Fig. ). It was found that glutamine and asparagine were the most potent inhibitors. Glutamine and asparagine inhibited more than 90%, whereas glycine inhibited about 85%. Alanine and methionine inhibited more than 70% and cysteine inhibited about 60%. In the cases of E. coli CycA and Acp from thermophilic bacterium PS3, glycine and alanine were the most potent inhibitors.Citation18) At this point, it is worthwhile to note that a BLAST similarity search of GlnT protein in Bacillus subtilis indicated that the GlnT protein belongs to the sodium:alanine symporter family, but it was biochemically verified as a glutamine transporter.Citation20) The competition experiments of our ApAgcS1 suggest that ApAgcS1 is a glutamine, asparagine, and glycine transporter. Regardless of these facts, it is also true that ApAgcS1 has a relatively broad substrate specificity toward neutral amino acids.
Fig. 4. Competition of glycine uptake by ApAgcS1.
Notes: The reaction mixtures contained 1 μM [U-14C]-glycine and 0.3 M NaCl. The concentration of various competitors was 100 μM. The value of uptake in the control (C) without a competitor is shown as 100%. Each value represents the average of three independent measurements and error bars represent standard deviations.
![Fig. 4. Competition of glycine uptake by ApAgcS1.Notes: The reaction mixtures contained 1 μM [U-14C]-glycine and 0.3 M NaCl. The concentration of various competitors was 100 μM. The value of uptake in the control (C) without a competitor is shown as 100%. Each value represents the average of three independent measurements and error bars represent standard deviations.](/cms/asset/e28162fe-809c-4cb6-a81a-39f814e90ef3/tbbb_a_968091_f0004_b.gif)
Expression of ApagcS1 was up-regulated under high-salinity and nitrogen-deficient conditions
Next, we examined the effect of nitrogen deficiency and salt stress on the gene expression of ApagcS1 in A. halophytica cells. RT-PCR analysis revealed that the expression of ApagcS1 was increased by three-fold after 1 h of depletion of nitrate (Fig. (A)). The expression of ApagcS1 was increased by 2.1-fold after 3 h of exposure of A. halophytica to a high-salinity medium containing 2.5 M NaCl (Fig. (B)). These results indicate that the expression of ApagcS1 is induced upon nitrogen deficiency as well as salt stress in A. halophytica cells.
Fig. 5. Expression of mRNA for ApagcS1.
Notes: (A) Nitrate-deficient stress. (B) Salt stress (2.5 M NaCl). A. halophytica cells were collected after 0, 1, 3, 6, 12, and 24 h growth. RT-PCR analysis was performed as described in “Materials and Methods.” The ribonuclease P(rnpB) gene was used as the control. The values at time 0 for each gene were set to 1.0. Asterisk indicates significant difference (p < 0.05) from the values at time 0. Each value represents the average of three independent measurements and error bars represent standard deviations.
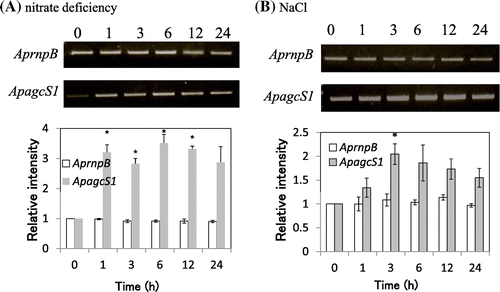
Glutamine is the best nitrogen source for cyanobacteria. It serves as an amino acid for protein synthesis and as a nitrogen donor for the synthesis of various nitrogenous molecules in the cell.Citation5) Therefore, the uptake of glutamine from the medium as well as de novo synthesis by glutamine synthetase is important under nitrogen-deficient conditions. Data on the induction of the ApagcS1 gene under nitrogen-deficient conditions are consistent with the view point that ApAgcS1 is involved in glycine/glutamine uptake.
Recapture of leaked neutral amino acids by ApAgcS1
It has been shown that the cyanobacteria mutant lacking a permease responsible for the uptake of neutral amino acids accumulate hydrophobic amino acids in the extracellular medium.Citation9) Therefore, we tested whether ApAgcS1 is involved in the recapture of leaked amino acids using S. elongatus PCC 7942 because the transformation of A. halophytica is not possible. Hitherto, the genes involved in the amino acid transport in S. elongatus PCC 7942 have not been reported. We searched the genome sequence of S. elongatus PCC 7942 and found that S. elongatus PCC 7942 has ABC-type neutral amino acid permeases, N-I and N-II.Citation9,10) We inactivated the natG gene (Synpcc7942_1894) encoding the permease subunit of N-II. The complete segregation was confirmed by the PCR amplification. As shown in Fig. , negligible amounts of amino acids were detected in the extracellular medium of wild-type S. elongatus PCC 7942. By contrast, the natG-deleted mutant accumulated considerable amounts of amino acids such as alanine, serine, glutamine, and tyrosine in the extracellular medium (Fig. ). The natG mutant overexpressing ApAgcS1 decreased the extracellular amino acids serine, glutamine, and isoleucine. The extracellular glycine was decreased, but it was not statistically significant. Fig. shows the results of the 24-h incubation. When the incubation time was 12 h, glycine was the only amino acid that was significantly recaptured by ApAgcS1 (data not shown). In Fig. , alanine which considerably competed for the glycine uptake in Fig. was not recaptured by ApAgcS1. Some inconsistency between Figs. and was observed. Fig. shows the results of competition of glycine uptake for a short term (1 min) in E. coli cells, whereas Fig. shows the results of amino acids after 24 h in the growth medium of S. elongatus PCC 7942 cells. Although further studies are required to clarify the reason, the data in Fig. suggest that ApAgcS1 is involved in the recapture of leaked amino acids, especially glutamine and serine.
Fig. 6. Extracellular amino acid accumulation in the culture medium.
Notes: The extracellular amino acids in the growth medium after 24 h incubation of S. elongatus PCC 7942 were measured. Wild-type, gray bar; ΔnatG mutant harbored empty vector, black bar; ΔnatG mutant harbored ApagcS1, white bar. Asterisk indicates significant difference (p < 0.05) between S. elongatus PCC 7942 cells expressing natG_empty vector_24 h and natG_pSyn1_ApagcS1_24 h. Each value represents the average of three independent measurements and error bars represent standard deviations.
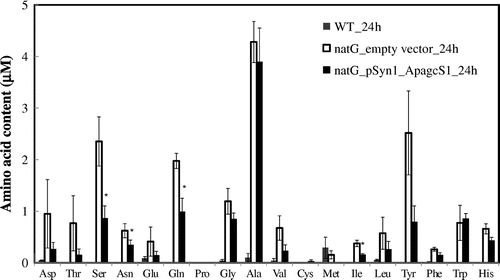
The strong competition of glycine uptake by glutamine (Fig. ) and the induction of the ApagcS1 gene under nitrogen-deficient conditions (Fig. ) suggest the uptake of glutamine by ApAgcS1. To confirm this, we examined the direct uptake of [U-14C] glutamine using the natG mutant. The natG mutant overexpressing ApAgcS1 up took [U-14C] glutamine, whereas, the natG mutant expressing only the vector did not uptake glutamine (data not shown). These facts indicate that ApAgcS1 functions as a glutamine transporter.
Conclusion
Based on the above data, we concluded that A. halophytica contains a unique sodium-dependent neutral amino acid transporter that exhibited high homology to AGCS family. Biochemical, RT-PCR, and deletion experiments showed that ApagcS1 is a sodium-dependent transporter and is involved in the uptake of glycine and glutamine.
Acknowledgments
This study was supported in part by Grants-in-Aid for Scientific Research from the Ministry of Education, Culture, Sports, Science, and Technology of Japan and the International Research Center for Natural Environmental Science of Meijo University (TT). It was also supported by the Office of the Higher Education Commission of Thailand, through a grant in the program “Strategic Scholarships for Frontier Research Network for the Ph.D. Program, Thai Doctoral Degree” and the 90th Anniversary of Chulalongkorn University Ratchadaphiseksomphot Endowment Fund for a Ph.D. Scholarship (to AB).
Notes
Abbreviations: Ap, Aphanothece halophytica; ApAgcS, Aphanothee alanine or glycine: cation symporter; Ec, Escherichia coli; LB medium, Luria-Bertani medium.
References
- Reizer J, Reizer A, Saier MH Jr. A functional superfamily of sodium/solute symporters. Biochim. Biophys. Acta. 1994;1197:133–166.10.1016/0304-4157(94)90003-5
- Jack DL, Paulsen IT, Saier MH. The amino acid/polyamine/organocation (APC) superfamily of transporters specific for amino acids, polyamines and organocations. Microbiology. 2000;146:1797–1814.
- Robbins JC, Oxender DL. Transport systems for alanine, serine, and glycine in Escherichia coli K-12. J. Bacteriol. 1973;116:12–18.
- Ghrist AC, Stauffer GV. The Escherichia coli glycine transport system and its role in the regulation of the glycine cleavage enzyme system. Microbiology. 1995;141:133–140.10.1099/00221287-141-1-133
- MacLeod PR, MacLeod RA. Identification and sequence of a Na+-linked gene from the marine bacterium Alteromonas haloplanktis which functionaily compiements the dagA gene of Escherichia coli. Mol. Microbiol. 1992;6:2673–2681.10.1111/mmi.1992.6.issue-18
- Kamata H, Akiyama S, Morosawa H, Ohota T, Toshiro Hamamoto T, Kambe T, Kagawa Y, Hirata H. Primary structure of the alanine carrier protein of thermophilic bacterium PS3. J. Biol. Chem. 1992;267:21650–21655.
- Kanamori M, Kamata H, Yagisawa H, Hirata H. Overexpression of the alanine carrier protein gene from thermophilic bacterium PS3 in Escherichia coli. J. Biochem. 1999;125:454–459.10.1093/oxfordjournals.jbchem.a022308
- Flores E, Herrero A. The cell biology of cyanobacteria. Norfolk: Caister Academic Press; 2014. p. 1–308.
- Pernil R, Picossi S, Mariscal V, Herrero A, Flores E. ABC-type amino acid uptake transporters Bgt and N-II of Anabaena sp. strain PCC 7120 share an ATPase subunit and are expressed in vegetative cells and heterocysts. Mol. Microbiol. 2008;67:1067–1080.10.1111/mmi.2008.67.issue-5
- Quintero MJ, Montesinos ML, Herrero A, Flores E. Identification of genes encoding amino acid permeases by inactivation of selected ORFs from the Synechocystis genomic sequence. Genome Res. 2001;11:2034–2040.10.1101/gr.196301
- Laloknam S, Tanaka K, Buaboocha T, Waditee R, Incharoensakdi A, Hibino T, Tanaka Y, Takabe T. Halotolerant cyanobacterium Aphanothece halophytica contains a betaine transporter active at alkaline pH and high salinity. Appl. Environ. Microbiol. 2006;72:6018–6026.10.1128/AEM.00733-06
- Boonburapong B, Laloknam S, Yamada N, Incharoensakdi A, Takabe T. Sodium-dependent uptake of glutamate by novel ApGltS enhanced growth under salt stress of halotolerant cyanobacterium Aphanothece halophytica. Biosci. Biotechnol. Biochem. 2012;76:1702–1707.10.1271/bbb.120309
- Waditee R, Tanaka Y, Aoki H, Hibino T, Jikuya H, Takano J, Takabe T, Takabe T. Isolation and functional characterization of N-methyltransferases that catalyze betaine synthesis from glycine in a halotolerant photosynthetic organism Aphanothece halophytica. J. Biol. Chem. 2005;278:4932–4942.
- Waditee-Sirisattha R, Kageyama H, Sopun W, Tanaka Y, Takabe T. Identification and upregulation of biosynthetic genes required for accumulation of Mycosporine-2-glycine under salt stress conditions in the halotolerant cyanobacterium Aphanothece halophytica. Appl. Environ. Microbiol. 2014;80:1763–1769.10.1128/AEM.03729-13
- Stanier RY, Kunisawa R, Mandel M, Cohen-Bazire G. Purification and properties of unicellular blue-green algae (Order Chroococcales). Bacteriol. Rev. 1971;35:171–205.
- Waditee R, Bhuiyan MN, Rai V, Aoki K, Tanaka Y, Hibino T, Suzuki S, Takano J, Jagendorf AT, Takabe T, Takabe T. Genes for direct methylation of glycine provide high levels of glycinebetaine and abiotic-stress tolerance in Synechococcus and Arabidopsis. Proc. Nat. Acad. Sci. USA. 2005;102:1318–1323.10.1073/pnas.0409017102
- Kyte J, Doolittle RF. A simple method for displaying the hydropathic character of a protein. J. Mol. Biol. 1982;157:105–132.10.1016/0022-2836(82)90515-0
- Akane S, Kamata H, Yagisawa H, Hirata H. A novel neutral amino acid transporter from the hyperthermophilic archaeon Thermococcus sp. KS-1. J. Biochem. 1999;133:173–180.
- MacLeod PR, MacLeod RA. Cloning in Escherichia coli K-12 of a Na+-dependent transport system from a marine bacterium. J. Bacteriol. 1986;165:825–830.
- Satomura T, Shimura D, Asai K, Sadaie Y, Hirooka K, Fujita Y. Enhancement of glutamine utilization in Bacillus subtilis through the GlnK-GlnL two-component regulatory system. J. Bacteriol. 2005;187:4813–4821.10.1128/JB.187.14.4813-4821.2005