Abstract
Photoconvertible water-soluble chlorophyll-binding proteins, called Class I WSCPs, have been detected in Chenopodiaceae, Amaranthaceae and Polygonaceae plant species. To date, Chenopodium album WSCP (CaWSCP) is the only cloned gene encoding a Class I WSCP. In this study, we identified two cDNAs encoding Chenopodium ficifolium Class I WSCPs, CfWSCP1, and CfWSCP2. Sequence analyses revealed that the open reading frames of CfWSCP1 and CfWSCP2 were 585 and 588 bp, respectively. Furthermore, both CfWSCPs contain cystein2 and cystein30, which are essential for the chlorophyll-binding ability of CaWSCP. Recombinant CfWSCP1 and CfWSCP2, expressed in Escherichia coli as hexa-histidine fusion proteins (CfWSCP1-His and CfWSCP2-His), formed inclusion bodies; however, we were able to solubilize these using a buffer containing 8 M urea and then refold them by dialysis. The refolded CfWSCP1-His and CfWSCP2-His could bind chlorophylls and exhibited photoconvertibility, confirming that the cloned CfWSCPs are further examples of Class I WSCPs.
Graphical Abstract
Identification and functional expression of photoconvertible water soluble chlorophyll-binding proteins from Chenopodium ficifolium.
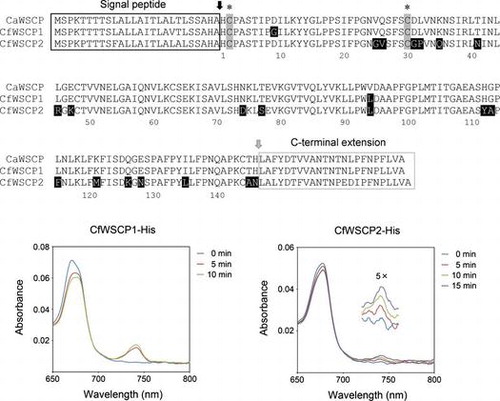
In plants, most chlorophyll proteins that play a role in photosynthesis are hydrophobic and are located in thylakoids. In addition to the photosynthesis-related proteins, non-photosynthetic water-soluble chlorophyll (Chl)-binding proteins (WSCPs) have also been found in plants of the Chenopodiaceae, Amaranthaceae, Polygonaceae, and Brassicaceae families.Citation1) WSCPs can be categorized into two classes; Class I (photoconvertible) and Class II (non-photoconvertible).Citation1) Class I WSCPs have been found in plant species of the Chenopodiaceae, Amaranthaceae, and Polygonaceae families.Citation2,3) Class II WSCPs have so far been extracted only from Brassicaceae plants.Citation4)
Chenopodium album WSCP (CaWSCP) was the first Class I WSCP to be identified. Before and after photoconversion, forms of the protein are called CP668 and CP742, respectively. Biochemical and physicochemical properties of CaWSCP have been well characterized.Citation3,5–17) The molecular size of CP668 is 69 kDa,Citation12) and that of a single subunit is 15,862 Da.Citation15) Therefore, CP668 (i.e. a Chl-CaWSCP complex) is considered a homotetramer. The photoconversion from CP668 to CP742 occurs only in the presence of molecular oxygen (i.e. in aerobic solutions). When Chl in CP668 is excited by light irradiation, it reacts with molecular oxygen, resulting in photoconversion.Citation8,11,13) Although CaWSCP could bind both Chl a and Chl b, only Chl a undergoes photoconversion.Citation7) The photoconversion reaction converts the chlorin skeleton of Chl a into a bacteriochlorin-like skeleton.Citation14,15) Recently, we found that the photoconversion is a three-step reaction and only three subunits of CP668 are photoconverted.Citation17) However, the detailed dynamics of this photoconversion reaction are still unclear.
To date, numerous genes encoding Class II WSCPs have been identified.Citation18–23) Furthermore, various molecular genetic analyses on recombinant Class II WSCPs have been performed.Citation18,20–32) On the other hand, CaWSCP is the only gene encoding a Class I WSCP identified to date and few studies on the molecular genetics of the protein have been performed thus far.Citation33,34)
Identification of novel genes encoding Class I WSCPs is essential for understanding the molecular diversity of the protein. In this study, we cloned two Chenopodium ficifolium genes encoding Class I WSCPs and expressed them in Escherichia coli to produce functional recombinant proteins.
Materials and methods
Plant materials
The C. ficifolium used in this study was collected near the Narashino campus of Toho University, Chiba, Japan in June.
Partial purification of CfWSCP
To avoid photoconversion of CfWSCP, we performed all procedures in dark conditions. We homogenized 100 g of fresh leaves and stems of C. ficifolium in 200 mL of 25 mM phosphate buffer (pH 7.0) using an electric blender, and the homogenate was filtered through four-layered gauze. Subsequently, we added ammonium sulfate to the filtrate, and the precipitate that formed between 30 and 70% saturation was collected by centrifugation. The precipitate was dissolved and dialyzed against the same buffer. The dialysate was used as a partially purified CfWSCP.
Evaluation of photoconversion activity
Photoconversion was induced by white light irradiation (2000 μmol photons m−2 S−1). Absorption spectra of the partially purified CfWSCP solution before and after photoconversion were measured using a U-3300 spectrophotometer (Hitachi).
Cloning of cDNAs encoding CfWSCP1 and CfWSCP2
We isolated total RNA of C. ficifolium using an RNeasy Mini Kit (Qiagen) with RNase-Free DNase (Qiagen). We then synthesized single-stranded cDNA from 100 ng of the RNA and an oligo dT(20) primer with a reverse transcriptase, ReverTra Ace (Toyobo). All procedures were performed according to the instructions provided by the manufacturers. The open reading frames of CfWSCP1 and CfWSCP2 were amplified by PCR with the primers 5′-GCAAAGTGTTCCAATAACAAAA-3′ and 5′-TGGAAGCGTGAAGTTGGCTTATTA-3′ and a KOD-Plus-Neo DNA polymerase (Toyobo). The PCR products were ligated into pGEM-T easy (Promega), and the constructs were introduced into E. coli (DH5α). We purified the plasmid DNAs from an overnight culture of the transformed E. coli using a PureLink Quick Plasmid Miniprep Kit (Invitrogen). We then sequenced the cloned fragments.
Sequence analysis
We obtained the open reading frame sequences for all members of the domain unknown function (DUF) 538 superfamily in Arabidopsis thaliana (AtDUF538s; At1g02813, At1g02816, At1g09310, At1g30020, At1g55265, At1g61667, At2g03350, At3g07460, At3g07470, At3g08890, At4g02360, At4g02370, At4g24130, At5g01610, At5g16380, At5g19590, At5g19860, At5g37070, At5g46230, At5g49600, and At5g54530) via TAIR (http://www.arabidopsis.org/). Alignment and phylogenetic analyses of the AtDUF538s, CaWSCP and CfWSCPs were performed with ClustalX.Citation35) The branch lengths shown on the tree are proportional to the genetic distance calculated by the neighbor-joining method.Citation36)
Expression of His-tag fused recombinant CfWSCPs
We amplified the DNA sequences of the deduced mature CfWSCP1 and CfWSCP2 by PCR using the primer sets; CfWSCP1 (5′-CATATGCACTGCCCTGCTTCAACGATCCCT-3′ and 5′-CTCGAGATGGGTACATTTGGGGGCTTGATT-3′); CfWSCP2 (5′-CATATGCACTGCCCTGCTTCAACAATCCCT-3′ and 5′-CTCGAGATTGGCACATTTGGGGGCTTGATT-3′) and ligated the PCR products into pGEM-T easy. After sequence analysis, the DNA sequences corresponding to the mature CfWSCP1 and CfWSCP2 were cut from pGEM-T easy and ligated into NdeI and XhoI sites of pET-24a (Novagen). Subsequently, the constructs were introduced into E. coli BL21 (DE3) codon plus (Novagen).
Two-milliliter aliquots of overnight cultures of the BL21 strains harboring the expression construct CfWSCP1::pET24 or CfWSCP2::pET24 were transferred into 200 mL lysogeny broth (LB) medium containing 50 μg/mL kanamycin. After incubation (3 h at 37 °C), isopropyl β-D-1-thiogalactopyranoside was added to a final concentration of 1 mM to induce expression of CfWSCP1-His or CfWSCP2-His, and the cultures were further incubated for 8 h at 37 °C. The BL21 strains expressing CfWSCP1-His or CfWSCP2-His were then collected and disrupted by sonication. Because both recombinant CfWSCPs formed inclusion bodies in the cell, we collected and solubilized insoluble fractions using a buffer comprising 20 mM tris–HCl (pH 8.0) and 8 M urea for 16 h at 4 °C. After solubilization, supernatants containing the soluble recombinant CfWSCPs were purified by Ni2+-chelating affinity chromatography. After dialysis against 20 mM tris–HCl (pH 8.0), the supernatants containing soluble CfWSCP1-His or CfWSCP2-His were collected by centrifugation. The CfWSCP1-His and CfWSCP1-His preparations were used immediately in the reconstitution analyses.
Reconstitution of recombinant CfWSCPs
To obtain thylakoids, we homogenized spinach leaves in an electric blender with 20 mM tris–HCl (pH 8.0). After filtration through four layers of gauze, a thylakoid pellet was obtained by centrifugation and used in the following reconstitution experiments. Chls a and b were purified from spinach leaves according to our previously reported methods.Citation21) The solutions containing renatured recombinant apo-forms of CfWSCP1-His, CfWSCP2-His, or control (20 mM tris–HCl (pH 8.0) containing 10% (v/v) methanol, mixed with the thylakoid pellet (in 20 mM tris–HCl, pH 8.0), or with Chl a or Chl b (in 20 mM Tri-HCl, pH 8.0)) were incubated at 25 °C for 1 h under dark conditions. After incubation, we collected supernatant fractions that contained reconstituted holo-forms of CfWSCP1-His or CfWSCP2-His by centrifugation and measured absorption spectra of the supernatants before and after photoconversion.
Results and discussion
Detection of CfWSCP
Takamiya has investigated the distribution of Class I WSCPs among various plant species.Citation2) They analyzed spectral changes (i.e. the decrease in absorbance at 668 nm and the increase at 742 nm caused by light irradiation) of supernatants from plant homogenates and found that Class I WSCPs were present in plant species of the Chenopodiaceae, Amaranthaceae, and Polygonaceae families.Citation2) Native CaWSCPs have been detected in the ammonium sulfate precipitation fraction between 30 and 70% saturation.Citation17) To analyze the distribution of Class I WSCPs in plant species, we obtained precipitates between 30 and 70% ammonium sulfate saturation of homogenates from various plants and measured absorption spectra of the partially purified samples. The partially purified sample derived from C. ficifolium exhibited photoconversion (Fig. ), indicating that C. ficifolium possesses Class I WSCPs. It should be noted that the amount of C. ficifolium WSCP (CfWSCP) was too small for further purification. Therefore, further molecular characterization of native CfWSCPs was not possible.
Cloning genes encoding CfWSCPs
We previously identified an open reading frame of a gene encoding a Brussels sprout WSCP, a Class II WSCP, using a primer set corresponding to the 5′-untranslated regions (UTRs) and 3′-UTRs of other Class II WSCPs.Citation21) We thus attempted to amplify the open reading frame of CfWSCPs using a primer set corresponding to the 5′-UTR and 3′-UTR of CaWSCP, and obtained approximately 600 bp PCR products. Sequence analysis of the cloned PCR products revealed two clones with different open reading frames that share high homology with CaWSCP. We designated the open reading frames as CfWSCP1 and CfWSCP2 (Figure S1). Like CaWSCP, both CfWSCP1 and CfWSCP2 are members of the DUF538 superfamily. DUF538 superfamily members are distributed in Embryophyta.Citation33) In Arabidopsis thaliana, there are 22 genes encoding DUF538 proteins.Citation34) A phylogenetic tree of CaWSCP, CfWSCP1, CfWSCP2, and AtDUF538s is shown in Figure S2. The phylogenetic tree indicated that the evolutionary branching point between the Class I WSCPs and AtDUF538s is distant while that between CaWSCP and CfWSCPs is quite close. Alignment of deduced amino acid sequences of CaWSCP, CfWSCP1 and CfWSCP2 is shown in Fig. . CaWSCP possesses an N-terminal extension predicted to be a signal peptide and a C-terminal extension whose function has not been characterized.Citation33) CfWSCP1 and CfWSCP2 also possess these extensions. Furthermore, the cysteine residues corresponding to cystein2 and cystein30 that are essential for Chl-binding of CaWSCP Citation34) are conserved in CfWSCP1 and CfWSCP2. It should be noted that these cysteine residues are not conserved in all AtDUF538 proteins.Citation34)
Fig. 2. Alignment of deduced amino acid sequences of CaWSCP, CfWSCP1, and CfWSCP2.
Notes: The alignment was generated by ClustalX. Black and gray arrows are post-translational cleavage sites for the signal peptide and C-terminal extension peptide, respectively. In mature regions, the amino acid residues highlighted by black background are different between CaWSCP and CfWSCPs. Asterisks indicate the cysteine residues that are essential for the Chl-binding ability of CaWSCP.
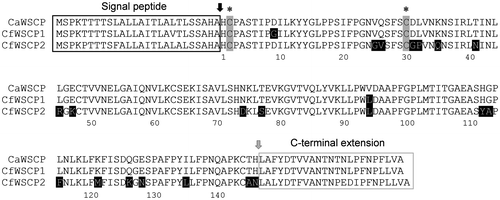
Expression of recombinant CfWSCP1 and CfWSCP2 in E. coli
To examine whether CfWSCP1 and CfWSCP2 are able to bind and photoconvert Chl as members of Class I WSCPs, we produced expression constructs tagged with C-terminal hexa-histidine for the deduced mature regions of CfWSCP1 and CfWSCP2 (CfWSCP1-His and CfWSCP2-His). Similar to CaWSCP-His,Citation33) almost all CfWSCP1-His and CfWSCP2-His expressed in E. coli BL21 (DE3) were present in the insoluble fraction of sonicated E. coli (Figure S3). The insoluble CfWSCPs were solubilized by 8 M urea treatment, then purified by Ni2+ affinity chromatography (Figure S3). Subsequently, the denatured CfWSCP1-His and CfWSCP2-His were refolded by dialysis, and the supernatants containing soluble CfWSCP1-His and CfWSCP2-His were collected by centrifugation (Figure S3). It should be noted that the refolded CaWSCP1-His and CfWSCP2-His (especially CfWSCP2-His) are unstable and tend to aggregate. Therefore the following reconstitution and photoconversion analysis was performed immediately after the refolding procedure.
Reconstitution and photoconversion of CfWSCP1-His and CfWSCP2-His
To reveal the Chl-binding and photoconversion abilities of CfWSCP1-His and CfWSCP2-His, the refolded CfWSCPs were mixed with thylakoid pellets prepared from spinach leaves. After incubation in the dark, supernatants containing reconstituted CfWSCP1-His or CfWSCP2-His were collected by centrifugation, then subjected to time-course spectrometric analysis. The absorption spectra of reconstituted CfWSCP1-His and CfWSCP2-His are shown in Fig. (A) and (B), respectively. When reconstituted CfWSCP1-His and CfWSCP2-His were illuminated, an absorption peak around 740 nm appeared, but no new peak appeared in the control (Fig. (C)), indicating that both CfWSCP1-His and CfWSCP2-His are photoconvertible.
Fig. 3. Absorption spectra (650–800 nm) of reconstituted CfWSCP1-His (A), CfWSCP2-His (B), and control (C).
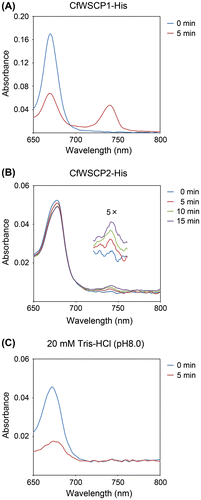
We measured the differential spectra of the CfWSCPs (Fig. ) to analyze changes in the absorption spectra during the photoconversion. In the differential spectra of CfWSCP1-His (Fig. (A)) and CfWSCP2-His (Fig. (B)), the three major peaks (432, 670, and 741 nm) were identical. In addition, three minor peaks (358, 395, and 563 nm) were present in the differential spectrum of CfWSCP1-His (Fig. (A)). The red-side peak at 741 nm, which is derived from a photoconverted pigment, was absent from the differential spectrum of the control (Fig. (C)), confirming that both CfWSCP1-His and CfWSCP2-His are photoconvertible.
Fig. 4. Differential spectra of CfWSCPs.
Notes: (A) Differential spectrum of reconstituted CfWSCP1-His (spectrum after 5 min irradiation, minus spectrum before irradiation), (B) Differential spectrum of reconstituted CfWSCP2-His (spectrum after 15 min irradiation, minus spectrum before irradiation), and (C) Differential spectrum of control fraction (20 mM tris–HCl (pH 8.0)) (spectrum after 5 min irradiation, minus spectrum before irradiation).
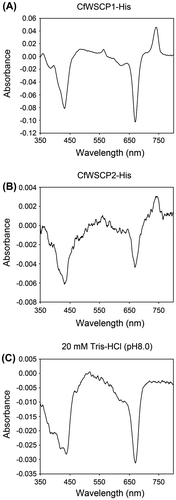
CaWSCP uses only Chl a as a substrate for photoconversion.Citation33) To examine the photoconvertibilities of CfWSCP1-His and CfWSCP2-His reconstituted with Chl a or Chl b, we attempted to reconstitute CfWSCPs with purified Chl a or Chl b in a buffer containing 10% methanol. We obtained CfWSCP1-His reconstituted with Chl a or Chl b. Compared with CaWSCP-His and CfWSCP1-His, CfWSCP2-His was quite unstable after refolding. Therefore, we could not reconstitute CfWSCP2-His with purified Chls in these conditions.
CfWSCP1-His reconstituted with Chl a was photoconvertible, while CfWSCP1-His reconstituted with Chl b was not, although the absorption peaks derived from Chl b were slightly decreased. The differential spectra (spectra after 5 min irradiation, minus spectra before irradiation) of the reconstituted proteins are shown in Fig. . The differential spectrum of CfWSCP1-His reconstituted with Chl a was identical to that of CfWSCP1-His reconstituted with the thylakoid pellet (Fig. (A)), but the differential spectrum of CfWSCP1-His reconstituted with Chl b was different. Furthermore, the photoconverted form of CaWSCP1-His reconstituted with Chl a had four peaks (361, 395, 563, and 742 nm) derived from photoconverted pigments, while the photoconverted form of CaWSCP1-His reconstituted with Chl b had no peaks derived from photoconverted pigments. These results are consistent with those reported previously for CaWSCP-His.Citation33) Our findings indicate that Chl a is the only substrate for the photoconversion in CfWSCP1. Additionally, the differential spectrum of CfWSCP2-His (Fig. (B)) was also similar to that of CfWSCP1-His reconstituted with Chl a (Fig. (A)), implying that CfWSCP2-His also uses Chl a as a substrate for the photoconversion. At present, the molecular mechanism of the photoconversion is poorly understood, although a few hypotheses have been proposed. Comparative analyses among Class I WSCPs can provide significant information to understand the photoconversion mechanism. In this study, we found two new genes encoding Class I WSCPs, and established expression systems for functional recombinant proteins. To reveal the mechanism of the photoconversion, we intend to conduct comparative analyses of these proteins.
Fig. 5. Differential spectra of CfWSCP1-His reconstituted with purified Chls.
Notes: (A) Differential spectrum of CfWSCP1-His reconstituted with Chl a (spectrum after 5 min irradiation, minus spectrum before irradiation), and (B) Differential spectrum of CfWSCP1-His reconstituted with Chl b (spectrum after 5 min irradiation, minus spectrum before irradiation). Measurements were performed in 20 mM tris–HCl buffer (pH 8.0) containing 10% methanol.
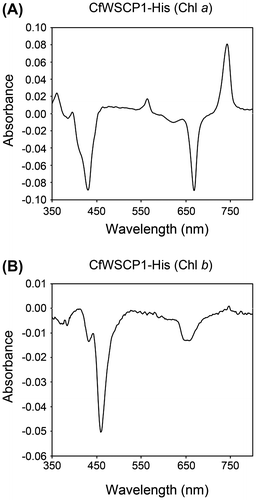
Conclusion
In this study, we found that C. ficifolium expresses Class I WSCPs. Furthermore, we found that the plant possess at least two genes encoding Class I WSCPs, CfWSCP1 and CfWSCP2. We also successfully expressed functional recombinant CfWSCP1-His and CfWSCP2-His. This finding confirmed that CfWSCP1 and CfWSCP2 are Class I WSCPs and that isoforms of Class I WSCP are present in C. ficifolium. These are the second examples of cloned Class I WSCPs.
Supplementary material
The supplemental material for this paper is available at http://dx.doi.org/10.1080/09168451.2014.972326.
Supplemental Materials
Download Zip (427.6 KB)Additional information
Funding
Notes
Abbreviations: Chl, chlorophyll; WSCP, water-soluble chlorophyll-binding protein.
The nucleotide sequences reported in this paper have been submitted to the DNA Data Bank of Japan (DDBJ) under accession numbers; AB972803 for CfWSCP1 and AB972804 for CfWSCP2.
References
- Satoh H, Uchida A, Nakayama K, Okada M. Water-soluble chlorophyll protein in Brassicaceae plants is a stress-induced chlorophyll-binding protein. Plant Cell Physiol. 2001;42:906–911.10.1093/pcp/pce117
- Takamiya A. Distribution of photoconvertible water-soluble chlorophyll protein complex CP668 in plants related to Chenopodium album. Carnegie Inst. Yearbook. 1973;72:330–336.
- Goedheer JC, Vos M. On the origin of the photoconvertible chlorophyll–protein complex Cp668 → Cp743 in Chenopodium and Amaranthus species. Acta Bot. Neerl. 1977;26:289–298.
- Takahashi S, Satoh H. Mini-review of the molecular properties and physiological functions of non-photoconvertible water-soluble chlorophyll-binding proteins (WSCPs) in Brassicaceae plants. In: Lang M, editor. Brassicaceae: characterization, functional genomics and health benefits. New York (NY): Nova Science Publishers; 2013. p. 111–120.
- Yakushiji E, Uchino K, Sugimura Y, Shiratori I, Takamiya F. Isolation of water-soluble chlorophyll protein from the leaves of Chenopodium album. Biochim. Biophys. Acta. 1963;75:293–298.10.1016/0006-3002(63)90615-2
- Terpstra W. Experiments on the extraction and photoconversion of Chenopodium chlorophyll protein CP 668. Biochim. Biophys. Acta. 1966;120:317–325.10.1016/0926-6585(66)90298-6
- Murata T, Odaka Y, Uchino K, Yakushiji E. Reconstitution of the photo-sensitive form of Chenopodium chlorophyll protein from its apoprotein. In: Shibata K, Takamiya A, Jagendorf AT, Fuller RC, editors. Comparative biochemistry and biophysics of photosynthesis. Tokyo: University of Tokyo Press; 1968. p. 222–228.
- Takamiya A, Kamimura Y, Kira A. A transient form of chlorophyll produced by flash photolysis of Chenopodium chlorophyll protein, CP668. In: Shibata K, Takamiya A, Jagendorf AT, Fuller RC, editors. Comparative biochemistry and biophysics of photosynthesis. Tokyo: University of Tokyo Press; 1968. p. 229–239.
- Oku T, Yoshida M, Tomita G. Heat stability of the phototransforming activity of Chenopodium chlorophyll protein. Plant Cell Physiol. 1972;13:183–186.
- Oku T, Yoshida M, Tomita G. The photoconversion of heat-treated Chenopodium chlorophyll protein and its pH dependence. Plant Cell Physiol. 1972;13:773–782.
- Hagar WG, French CS. Energy transfer in chlorophyll protein 668: evidence for a stable electronic state involvement in its phototransformation. Carnegie Inst. Yearbook. 1974;73:706–716.
- Oku T, Tomita G. The photoconversion of heat-treated Chenopodium chlorophyll protein and its pH dependence. Plant Cell Physiol. 1975;16:1009–1016.
- Hagar WG, Hiyama T. Characterization of the light-induced transient states of the chlorophyll proteins 668 and 743 from Atriplex rosea. Plant Physiol. 1979;63:1182–1186.10.1104/pp.63.6.1182
- Noguchi T, Kamimura Y, Inoue Y, Itoh S. Photoconversion of a water-soluble chlorophyll protein from Chenopodium album: resonance Raman and Fourier transform infrared study of protein and pigment structures. Plant Cell Physiol. 1999;40:305–310.10.1093/oxfordjournals.pcp.a029542
- Hirabayashi H, Amakawa M, Kamimura Y, Shino Y, Satoh H, Itoh S, Tamiaki H. Analysis of photooxidized pigments in water-soluble chlorophyll protein complex isolated from Chenopodium album. J. Photochem. Photobiol. 2006;183:121–125.10.1016/j.jphotochem.2006.03.003
- Ohtsuki T, Ohshima S, Uchida A. Purification, crystallization and preliminary X-ray diffraction analysis of water-soluble chlorophyll-binding protein from Chenopodium album. Acta Crystallogr. Sect. F Strct. Biol. Csyst. Commun. 2007;63:740–741.10.1107/S1744309107035658
- Takahashi S, Uchida A, Nakayama K, Satoh H. Three-step photoconversion of only three subunits of the water-soluble chlorophyll-binding protein tetramer from Chenopsodium album. Protein J. 2014;33:337–343.
- Satoh H, Nakayama K, Okada M. Molecular cloning and functional expression of a water-soluble chlorophyll Protein, a putative carrier of chlorophyll molecules in cauliflower. J. Biol. Chem. 1998;273:30568–30575.10.1074/jbc.273.46.30568
- Satoh H, Zanma A, Shinashi K. Molecular cloning and sequence analysis of a water-soluble chlorophyll protein cDNA from Japanese radish. J. Plant Physiol. 2002;159:325–327.10.1078/0176-1617-00704
- Bektas I, Fellenberg C, Paulsen H. Water-soluble chlorophyll protein (WSCP) of Arabidopsis is expressed in the gynoecium and developing silique. Planta. 2012;236:251–259.10.1007/s00425-012-1609-y
- Takahashi S, Yanai H, Nakamaru Y, Uchida A, Nakayama K, Satoh H. Molecular cloning, characterization and analysis of the intracellular localization of a water-soluble chl-binding protein from Brussels Sprouts (Brassica oleracea var. gemmifera). Plant Cell Physiol. 2012;53:879–891.10.1093/pcp/pcs031
- Takahashi S, Ono M, Uchida A, Nakayama K, Satoh H. Molecular cloning and expression of a water-soluble chlorophyll-binding protein from Japanese wild radish. J. Plant Physiol. 2013;170:406–412.10.1016/j.jplph.2012.10.007
- Takahashi S, Yanai H, Oka-Takayama Y, Zanma-Sohtome A, Fujiyama K, Uchida A, Nakayama K, Satoh H. Molecular cloning, characterization and analysis of the intracellular localization of a water-soluble chlorophyll-binding protein (WSCP) from Virginia pepperweed (Lepidium virginicum), a unique WSCP that preferentially binds chlorophyll b in vitro. Planta. 2013;238:1065–1080.10.1007/s00425-013-1952-7
- Schmidt K, Fufezan C, Krieger-Liszkay A, Satoh H, Paulsen H. Recombinant water-soluble chlorophyll protein from Brassica oleracea var. Botrys binds various chlorophyll derivatives. Biochemistry. 2003;42:7427–7433.
- Hughes JL, Razeghifard R, Logue M, Oakley A, Wydrzynski T, Krausz E. Magneto-optic spectroscopy of a protein tetramer binding two exciton-coupled chlorophylls. J. Am. Chem. Soc. 2006;128:3649–3658.10.1021/ja056576b
- Renger T, Trostmann I, Theiss C, Madjet ME, Richter M, Paulsen H, Eichler HJ, Knorr A, Renger G. Refinement of a structural model of a pigment−protein complex by accurate optical line shape theory and experiments. J. Phys. Chem. B. 2007;111:10487–10501.10.1021/jp0717241
- Renger T, Madjet ME, Müh F, Trostmann I, Schmitt FJ, Theiss C, Paulsen H, Eichler HJ, Knorr A, Renger G. Thermally activated superradiance and intersystem crossing in the water-soluble chlorophyll binding protein. J. Phys. Chem. B. 2009;113:9948–9957.10.1021/jp901886w
- Theiss C, Trostmann I, Andree S, Schmitt FJ, Renger T, Eichler HJ, Paulsen H, Renger G. Pigment−pigment and pigment−protein interactions in recombinant water-soluble chlorophyll proteins (WSCP) from cauliflower. J. Phys. Chem. B. 2007;111:13325–13335.10.1021/jp0723968
- Schmitt FJ, Trostmann I, Theiss C, Pieper J, Renger T, Fuesers J, Hubrich EH, Paulsen H, Eichler HJ, Renger G. Excited state dynamics in recombinant water-soluble chlorophyll proteins (WSCP) from cauliflower investigated by transient fluorescence spectroscopy. J. Phys. Chem. B. 2008;112:13951–13961.10.1021/jp8024057
- Pieper J, Rätsep M, Trostmann I, Paulsen H, Renger G, Freiberg A. Excitonic energy level structure and pigment−protein interactions in the recombinant water-soluble chlorophyll protein. I. Difference fluorescence line-narrowing. J. Phys. Chem. B. 2011;115:4042–4052.10.1021/jp111455g
- Pieper J, Rätsep M, Trostmann I, Schmitt FJ, Theiss C, Paulsen H, Eichler HJ, Freiberg A, Renger G. Excitonic energy level structure and pigment−protein interactions in the recombinant water-soluble chlorophyll protein. II. Spectral hole-burning experiments. J. Phys. Chem. B. 2011;115:4053–4065.10.1021/jp111457t
- Takahashi S, Uchida A, Nakayama K, Satoh H. The C-terminal extension peptide of non-photoconvertible water-soluble chlorophyll-binding proteins (Class II WSCPs) affects their solubility and stability: comparative analyses of the biochemical and chlorophyll-binding properties of recombinant Brassica, Raphanus and Lepidium WSCPs with or without their C-terminal extension peptides. Protein J. 2014;33:75–84.10.1007/s10930-013-9539-5
- Takahashi S, Yoshikawa M, Kamada A, Ohtsuki T, Uchida A, Nakayama K, Satoh H. The photoconvertible water-soluble chlorophyll-binding protein of Chenopodium album is a member of DUF538, a superfamily that distributes in Embryophyta. J. Plant Physiol. 2013;170:1549–1552.10.1016/j.jplph.2013.06.001
- Takahashi S, Seki Y, Uchida A, Nakayama K, and Satoh H, Cysteine-2 and Cys30 are essential for chlorophyll-binding activity of the water-soluble chlorophyll-binding protein (WSCP) of Chenopodium album. Biosci. Biotechnol. Biochem., doi:10.1080/09168451.2014.940274(in press).
- Thompson JD, Gibson TJ, Plewniak F, Jeanmougin F, Higgins DG. The CLUSTAL_X windows interface: flexible strategies for multiple sequence alignment aided by quality analysis tools. Nucleic. Acids. Res. 1997;25:4876–4882.10.1093/nar/25.24.4876
- Saitou N, Nei M. The neighbor-joining method: a new method for reconstructing phylogenetic trees. Mol. Biol. Evol. 1987;4:406–425.